JWST Features
Science
Feature Stories of the Solar System and Beyond
• February 16, 2022: So far, only two interstellar objects have been spotted buzzing through our solar system, but scientists think many more are lurking. When the next one is discovered, NASA’s powerful new James Webb Space Telescope may have a ringside seat for analyzing this interloper. Webb will help astronomers learn about its composition, formation history, and home system. For the first time, scientists will get an up-close-and-personal view of something born outside our solar system.
- One of the most exciting findings in planetary science in recent years is the discovery of interstellar objects passing through our solar system. So far, astronomers have confirmed only two of these interlopers from other star systems — 1I/'Oumuamua in 2017 and 2I/Borisov in 2018 — but many, many more are thought to exist. Scientists have had only limited ability to study these objects once discovered, but all of that is about to change with NASA's James Webb Space Telescope. 1)
![Figure 1: This artist’s illustration shows the first identified interstellar visitor, 1I/'Oumuamua, discovered in 2017. The wayward object swung within 24 million miles of the Sun before racing out of the solar system. 'Oumuamua still defies any simple categorization. It did not behave like a comet, and it had a variety of unusual characteristics. As the complex rotation of the object made it difficult to determine the exact shape, there are many models of what it could look like[image credit: ARTWORK: NASA, ESA, Joseph Olmsted (STScI), Frank Summers (STScI)]](https://eoportal.org/ftp/satellite-missions/j/JWST-Feature_170222/JWST-Feature_Auto1C.jpeg)
![Figure 2: This Hubble Space Telescope image of 2I/Borisov shows the first observed rogue comet, a comet from interstellar space that is not gravitationally bound to a star. It was discovered in 2019 and is the second identified interstellar interloper, after 'Oumuamua. 2I/Borisov looks a lot like the traditional comets found inside our solar system, which sublimate ices and cast off dust as they are warmed by the Sun. The wandering comet provided invaluable clues to the chemical composition, structure, and dust characteristics of planetary building blocks presumably forged in an alien star system. It’s rapidly moving away from our Sun and will eventually head back into interstellar space, never to return [image credit: IMAGE: NASA, ESA, David Jewitt (UCLA), IMAGE PROCESSING: Joseph DePasquale (STScI)]](https://eoportal.org/ftp/satellite-missions/j/JWST-Feature_170222/JWST-Feature_Auto1B.jpeg)
- "The supreme sensitivity and power of Webb now present us with an unprecedented opportunity to investigate the chemical composition of these interstellar objects and find out so much more about their nature: where they come from, how they were made, and what they can tell us about the conditions present in their home systems," explained Martin Cordiner, principal investigator of a Webb Target of Opportunity program to study the composition of an interstellar object.
- "The ability to study one of these and find out its composition — to really see material from around another planetary system close up — is truly an amazing thing," said Cordiner, an astrophysicist at NASA's Goddard Space Flight Center in Greenbelt, Maryland and The Catholic University of America. The first two interstellar objects detected were very different: One was very comet-like, and one was not. Cordiner and his team hope to find out how unique those objects were and whether they're representative of the broader population of interstellar objects.
Triggering Process
- Astronomers are constantly monitoring various sources of information, ranging from amateur observers to professional observatories, in the hopes of finding the next interstellar interloper. When the next such object is first detected, scientists won't immediately be certain if it's an interstellar object. They'll need additional observations over a period of days, weeks, or even months to confirm it — depending on its brightness.
- Once they have confirmation that the object came from outside the solar system based on its "hyperbolic" orbit, and they are certain the object didn't come from the outer reaches of our own solar system or the Oort cloud, they can calculate the trajectory of the object across the sky. If that trajectory intersects with Webb's viewing field, Cordiner and his team will make the observations.
The Science
- The team will use Webb's spectroscopic capabilities in both the near-infrared and mid-infrared bands to study two different aspects of the interstellar object. First, using the Near-Infrared Spectrograph (NIRSpec), they will analyze the chemical fingerprints of gases released by the object as any ices that might be present are vaporized by our Sun's heat. Second, with the Mid-Infrared Instrument (MIRI), they will observe any dust that the object is producing — small, microscopic particles; larger grains; and even pebbles that may be lifted off the surface and surrounding the object.
- With its high spectral resolution, NIRSpec can pick out the emission from individual gases, allowing the team to detect specific molecules such as water, methanol, formaldehyde, carbon dioxide, carbon monoxide, and methane. MIRI, in the mid-infrared, is more tuned to the heat spectrum produced by solid particles, such as dust grains or the object's nucleus.
Powerful New Insights
- In our own solar system, comets are icy remnants from the era of planet formation around our Sun, so they can provide unique insight into the chemical conditions present in the earliest history of our solar system. This Webb program has the ability to reveal — for the first time — similarly powerful insights into the chemistry of the formation of planets around other stars.
- Astronomers don't fully understand the exact chemical processes involved in forming planets. For example, how does a planet arise from simple chemical ingredients? Does it happen in the same way around all stars? Was there anything peculiar about the way our own planets formed around our Sun, compared with how they form around other stars elsewhere in the galaxy? If scientists can get proof of the chemical conditions present in other planetary systems by observing an interstellar object and seeing what it's made of, then they can get a much clearer picture of the true extent of chemistry that's possible in those other planetary systems.
A New Window with Webb
- Interstellar objects have not been observed before in these important near- and mid-infrared wavelength ranges, so the possibilities for new discoveries are quite profound. With trillions and trillions of interstellar objects buzzing around the galaxy, the team doesn't know what they are going to find, but they know that it will be fascinating.
- "With Webb, we can do really interesting science at much fainter magnitudes or brightnesses," explained teammate Cristina Thomas, an assistant professor of astronomy at Northern Arizona University. "Also, we've never been able to observe interstellar objects in this region of the infrared. It opens a lot of opportunities for the different compositional signatures that we're interested in. That's going to be a huge boon for us!"
• January 19, 2022: To understand galaxies, you have to understand how stars form. Over 100 researchers from around the world have collaborated to bring together observations of nearby spiral galaxies taken with the world’s most powerful radio, visible, and ultraviolet telescopes – and will soon add a full suite of high-resolution infrared images from NASA’s James Webb Space Telescope. With this groundbreaking data set, astronomers will be able to study stars as they start to form within dark, dusty gas clouds, untangle when those infant stars blow away that gas and dust, and identify more mature stars that are puffing off layers of gas and dust – all for the first time in a diverse set of spiral galaxies. 2)
- Spirals are some of the most captivating shapes in the universe. They appear in intricate seashells, carefully constructed spider webs, and even in the curls of ocean waves. Spirals on cosmic scales – as seen in galaxies – are even more arresting, not only for their beauty, but also for the overwhelming amount of information they contain. How do stars and star clusters form? Until recently, a complete answer used to lie out of reach, blocked by gas and dust. Within the first year of operations, NASA’s James Webb Space Telescope will help researchers complete a more detailed sketch of the stellar life cycle with high-resolution infrared-light images of 19 galaxies.
![Figure 3: This image of spiral galaxy NGC 3351 combines observations from several observatories to reveal details about its stars and gas. Radio observations from the Atacama Large Millimeter/submillimeter Array (ALMA) show dense molecular gas in magenta. The VLT's (Very Large Telescope) Multi Unit Spectroscopic Explorer (MUSE) instrument highlights where young massive stars illuminate their surroundings, set off in red. The Hubble Space Telescope’s images highlight dust lanes in white and newly formed stars in blue. High-resolution infrared images from the Webb Space Telescope will help researchers identify where stars are forming behind dust and study the earliest stages of star formation in this galaxy [image credit: Science: NASA, ESA, ESO-Chile, ALMA, NAOJ, NRAO; Image Processing: Joseph DePasquale (STScI)]](https://eoportal.org/ftp/satellite-missions/j/JWST-Feature_170222/JWST-Feature_Auto1A.jpeg)
- The telescope will also provide a few key “puzzle pieces” that were missing until now. “JWST touches on so many different phases of the stellar life cycle – all in tremendous resolution,” said Janice Lee, Gemini Observatory chief scientist at the National Science Foundation’s NOIRLab in Tucson, Arizona. “Webb will reveal star formation at its very earliest stages, right when gas collapses to form stars and heats up the surrounding dust.”
- Lee is joined by David Thilker of the Johns Hopkins University in Baltimore, Maryland, Kathryn Kreckel of Heidelberg University in Germany, and 40 additional members of the multi-wavelength survey program known as PHANGS (Physics at High Angular resolution in Nearby GalaxieS). Their mission? Not only to unravel the mysteries of star formation with Webb’s high-resolution infrared images, but also to share the datasets with the entire astronomical community to accelerate discovery.
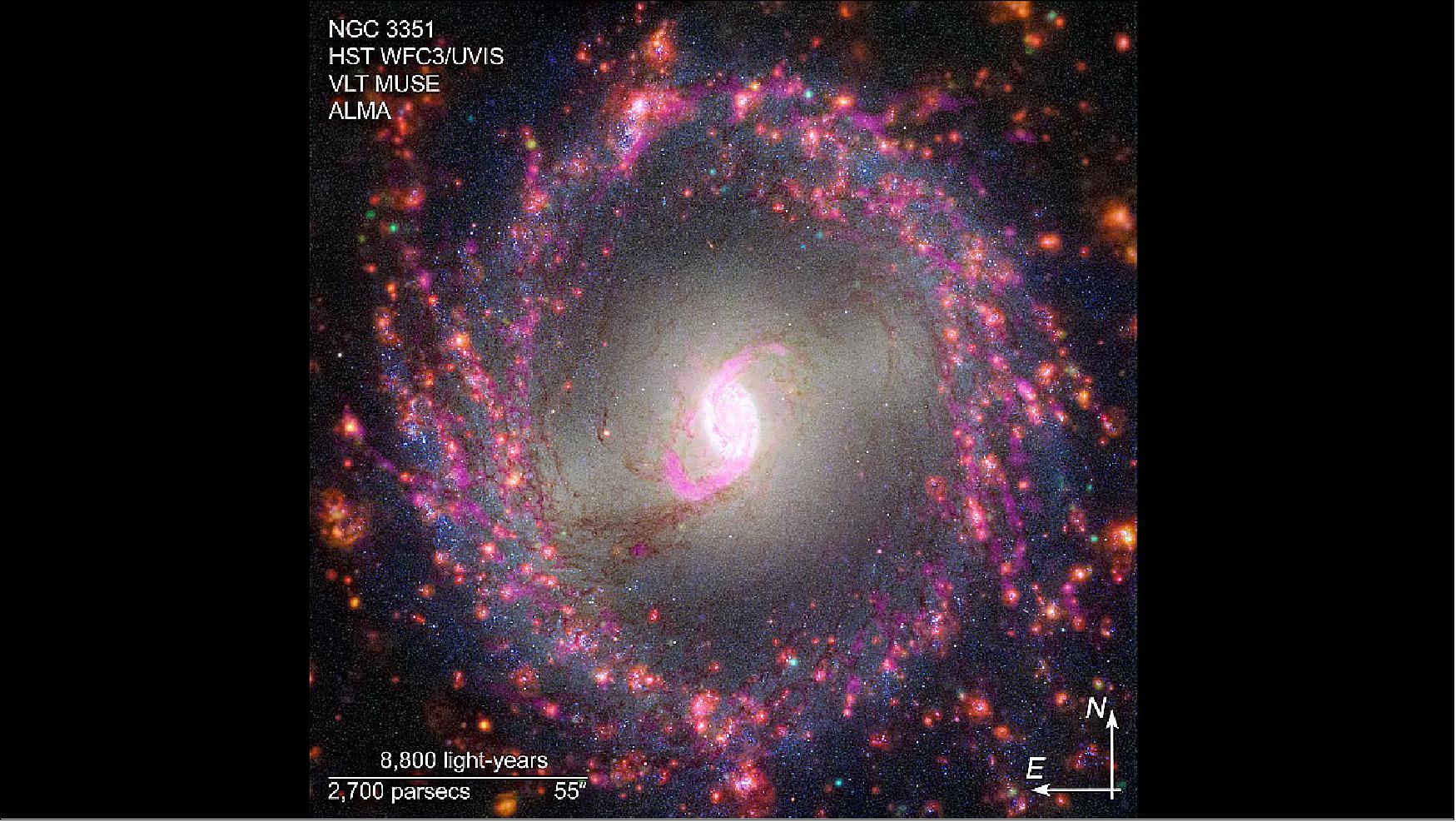
The Rhythms of Star Formation
- PHANGS is novel, in part, because it brought together more than 100 international experts to study star formation from beginning to end. They are targeting galaxies that can be seen face-on from Earth and that are, on average, 50 million light-years away. The large collaboration began with microwave light images of 90 galaxies from the Atacama Large Millimeter/submillimeter Array (ALMA) in Chile. Astronomers use this data to produce molecular gas maps to study the raw materials for star formation. Once the Very Large Telescope’s Multi Unit Spectroscopic Explorer (MUSE) instrument, also in Chile, came online, they obtained data known as spectra to study later phases of star formation of 19 galaxies, particularly after star clusters have cleared nearby gas and dust. The space-based Hubble Space Telescope has provided visible and ultraviolet light observations of 38 galaxies to add high-resolution images of individual stars and star clusters.
- The missing elements, which Webb will fill in, are largely in areas of the galaxies that are obscured by dust – regions where stars are actively beginning to form. “We’re going to clearly see star clusters in the hearts of these dense molecular clouds that before we only had indirect evidence of,” Thilker said. “Webb gives us a way to look inside these ‘star factories’ to see the freshly assembled star clusters and measure their properties before they evolve.”
- The new data will also help the team pinpoint the ages of stellar populations in a diverse sample of galaxies, which will help researchers build more accurate statistical models. “We’re always putting the context of the small scales into the big picture of galaxies,” explained Kreckel. “With Webb, we’ll trace the evolutionary sequence of each galaxy’s stars and star clusters.”
- Another important answer they’re seeking involves the dust surrounding the stars, within the interstellar medium. Webb will help them determine which areas of the gas and dust are associated with specific star-forming regions, and which are free-floating interstellar material. “This couldn't be done before, beyond the nearest galaxies. It will be transformative,” Thilker added.
- The team is also working to understand the timing of the star-formation cycle. “Timescales are critical in astronomy and physics,” Lee said. “How long does each stage of star formation last? How might those timelines vary in different galaxy environments? We want to measure when these stars free themselves from their gas clouds to understand how star formation is disrupted.”
Science for All
- These Webb observations will be taken as part of a Treasury program, which means they are not only available immediately to the public, but they will also be of broad and enduring scientific value. The team will work to create and release data sets that align Webb’s data to each of the complementary data sets from ALMA, MUSE, and Hubble, allowing future researchers to sift through each galaxy and their stellar populations easily, toggling on and off various wavelengths – and zoom into individual pixels of the images. They will provide inventories of different phases of the star-formation cycle, including regions of star formation, young stars, star clusters, and local dust properties.
- This research will be conducted as part of Webb’s General Observer (GO) programs, which are competitively selected using a dual-anonymous review system, the same system that is used to allocate time on the Hubble Space Telescope.
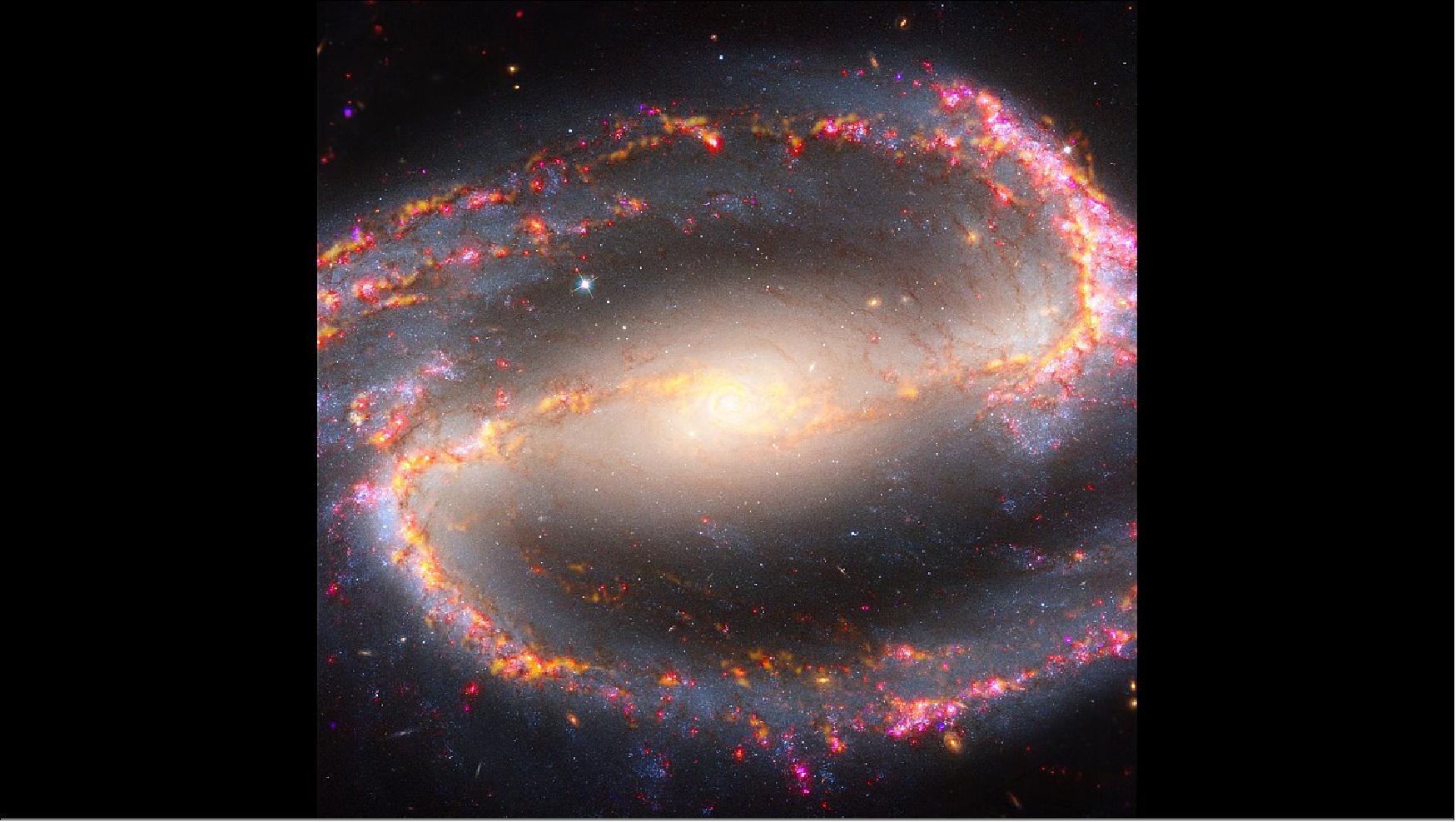
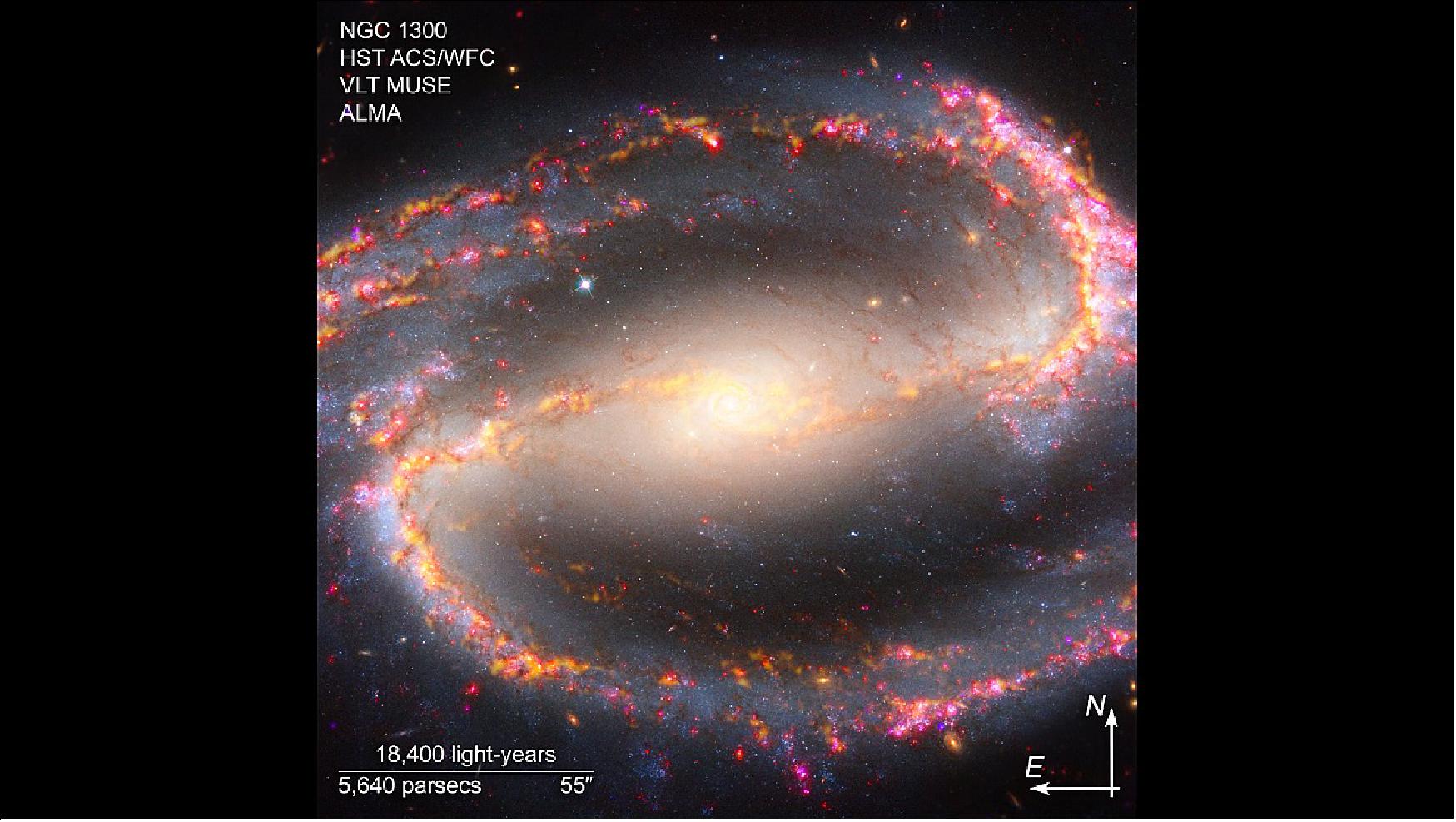
• December 8, 2021: Researchers will examine three active supermassive black holes – known as quasars – their host galaxies, and their neighborhoods to better detail these distant objects and the conditions of the early universe. 3)
- When astronomers discovered point-like objects in all-sky radio surveys in the 1950s, they weren’t sure how to categorize them. Were they stars, galaxies – or something else altogether? A great, new field of exploration opened. These quasi-stellar radio sources became known as quasars and today we better appreciate how fascinating they truly are: Quasars are active supermassive black holes that reveal themselves through their light shows. Gas and dust orbit these supermassive black holes continuously rubbing together to create heat and light, which we can detect.
- The three most distant quasars currently known were discovered since 2018 – each located more than 13 billion light-years away. The James Webb Space Telescope will offer researchers new views of these objects in high-resolution infrared light. With these powerful data, a research team aims to refine the calculations of the masses of their black holes, detail the stars in their host galaxies, and survey the galaxies in their neighborhoods. What’s more, their research may influence how we view this early era of the universe.
- Very distant, active supermassive black holes are the brightest beacons in the universe. Known as quasars, these behemoths are surrounded by equally distant galaxies. In recent decades, researchers have gone on a cosmic treasure hunt and identified the three most distant quasars known over the last three years – each more than 13 billion light-years from Earth. Astronomers theorize that it can take billions of years for supermassive black holes and their accompanying galaxies to form. How is it possible that these quasars became so gigantic, with billions of solar masses, in the first 700 million years of the universe? Once you can see past their glare, what do their accompanying galaxies look like? And what do their “neighborhoods” look like?
- These are questions Xiaohui Fan and Jinyi Yang, both of the University of Arizona, and Eduardo Bañados, of the Max Planck Institute for Astronomy in Heidelberg, Germany, with an international team of astronomers, will pursue with observations taken by the James Webb Space Telescope. “These are really valuable objects,” Fan said. “We structured this program to learn everything we could think of so our team and the greater astronomical community can fully explore these quasars.”
![Figure 7: Researchers will use all four instruments aboard the James Webb Space Telescope to study the three most distant quasars yet discovered. They will obtain new measurements of the masses of their central supermassive black holes, detail the stars and composition of their host galaxies, and observe nearby galaxies to learn more about their “neighborhoods” in the early universe. - The three targets of this research program at a glance: J0313-1806 dates back to 670 million years after the big bang and is 1.6 billion times more massive than our Sun. J1007+2115, or Pōniuā'ena, was detected approximately 700 million years after the big bang and is 1.5 billion times more massive than our Sun. The third target, J1342+0928, dates back to 690 million years after the big bang and is 800 million times the mass of our Sun [credits: ARTWORK: NASA, ESA, CSA, Joseph Olmsted (STScI)]](https://eoportal.org/ftp/satellite-missions/j/JWST-Feature_170222/JWST-Feature_Auto16.jpeg)
- Webb’s sensitivity to infrared light – including mid-infrared wavelengths that can only be captured from space – will allow the team to observe these objects, whose light has traveled for 13 billion years and has had its wavelengths stretched from ultraviolet and visible light into infrared light. Webb has unmatched sensitivity and spatial resolution, which will reveal complex structures in these distant objects.
- The team plans to observe and analyze the data on three scales: closely examining the quasars themselves, studying the stars in the surrounding host galaxies after removing the quasars’ light, and classifying the galaxies that lie nearby. “These quasars are very special objects,” explained Bañados. “That is why we want to provide the best characterization possible of each with Webb.”
‘Zooming’ in – and out
- Fan, Yang, and Bañados are wasting no opportunity: They will use almost every available instrument on Webb to observe these quasars. First, they will refine the measurements of the mass of each supermassive black hole. “The existence of these black holes challenges theoretical models,” Yang said. “We want to obtain more accurate measurements of their masses to improve our understanding of how they formed and grew so quickly.”
- To increase the precision of existing measurements from other observatories, they’ll turn to spectra – data that detail an object’s physical properties, including mass and chemical composition, delivered by Webb’s Near-Infrared Spectrograph (NIRSpec). This will allow the team to produce more accurate black hole masses.
- Next, they will focus on revealing the galaxies behind the quasars’ bright light. They will take very deep, detailed images of each target with Webb’s Near-Infrared Camera (NIRCam) and then use computer models to remove the quasars’ light from each. The final, processed images will give them the first views of the light from the stars in the host galaxies. The team will also obtain spectra with Webb’s Mid-Infrared Instrument (MIRI). No one can fully predict what they’ll learn. Were these ancient galaxies more compact? Do their stars contain more than hydrogen and helium? Webb will certainly yield new insights!
- The team will also obtain spectra of both the quasars and their host galaxies to trace how gas is moving in the host galaxies and determine if the active supermassive black holes are sending out hot winds that heat the galaxies’ gas. Although no one can watch a complete feedback loop in real time (it takes millions of years!), they can sample what’s present with NIRSpec and begin to observe the connections between the quasars and their host galaxies.
- They will also “zoom out” to see galaxies near these quasars. Webb’s expansive, high-resolution observations will help the team characterize the galaxies that are in the neighborhood by employing Webb’s Near-Infrared Imager and Slitless Spectrograph (NIRISS) and NIRCam.
- Finally, the researchers will also sample the large-scale environments around the quasars – the characteristics of the gas and dust. What was the universe like 700 or 800 million years after the big bang? This was a period known as the Era of Reionization, when the gas between galaxies was largely opaque. Only after the first billion years of the universe did the gas become fully transparent, allowing light to travel more easily. The team will measure everything that is between us and the quasars with NIRSpec. “We know that these quasars exist when the universe was about fifty percent neutral,” Bañados explained. “These targets represent an important age of the universe – essentially the peak of this transition. Webb will provide new constraints about what this period was like.”
- Fan, Yang, and Bañados will share the riches of this thorough observation program by releasing data and tools to the astronomical community to accelerate overall research of quasars in the early universe. “Webb will help us make the next quantum leap in understanding these objects,” said Fan.
- This research will be conducted as part of Webb’s General Observer (GO) programs, which are competitively selected using a dual-anonymous review system, the same system that is used to allocate time on the Hubble Space Telescope.
• November 17, 2021: Detailed atmospheric studies will provide key insights into some of the most common – and mysterious – planets known in the galaxy. More than half of the Sun-like star systems surveyed in the Milky Way harbor a mysterious type of planet unlike any in our own solar system. 4)
- Larger than Earth, smaller than Neptune, and orbiting closer to their stars than Mercury orbits the Sun, these warm-to-hot sub-Neptunes are the most common type of planet observed in the galaxy. But although researchers have been able to measure basic properties – including size, mass, and orbit – of hundreds of these planets, their fundamental nature remains unclear.
- Are they dense, Earth-like balls of rock and iron, blanketed in thick layers of hydrogen and helium gas? Or less dense mixtures of rock and ice, surrounded by steamy, water-rich atmospheres? With limited data and no planets of similar size and orbit in our own solar system to use for comparison, it has been difficult to answer these questions.
- “What are these planets? How do they form? Why doesn’t our solar system have them? These are fundamental questions,” explains Jacob Bean, an astronomer at the University of Chicago who has led numerous observations of exoplanets.
![Figure 8: Illustration of what exoplanet TOI-421 b might look like. TOI-421 b is a hot sub-Neptune-sized exoplanet orbiting a Sun-like star roughly 244 light-years from Earth. TOI-421 b is thought to have a clear atmosphere free of haze and clouds. This illustration is based on our knowledge of the planet and its host star, and predictions about the likely properties of the planet’s atmosphere. Spectroscopic data from Webb will help us better understand the composition of the planet’s atmosphere. - TOI-421 b is in between Earth and Neptune in terms of size (radius 2.68 times Earth), mass (7.2 times Earth), and density (2 times water). It orbits its star at a distance of only 0.056 astronomical unit (5.6% of the distance between Earth and the Sun), completing one orbit in 5.2 Earth-days. The star, TOI-421, has a radius 0.87 times that of the Sun, a mass 0.85 times the Sun, and a temperature of 5325 kelvins (~5050º Celsius), slightly cooler than the Sun [image credits: NASA, ESA, CSA, Dani Player (STScI)]](https://eoportal.org/ftp/satellite-missions/j/JWST-Feature_170222/JWST-Feature_Auto15.jpeg)
The Haze Problem
- The key to figuring out what sub-Neptunes are made of and how they formed is examining their atmospheres. But getting a clear view has not been easy.
- The most effective method of analyzing exoplanet atmospheres is a technique known as transmission spectroscopy. When the planet is transiting its star, some wavelengths (colors) of starlight are filtered out by gases in the planet’s atmosphere. Because each type of gas has a unique “signature,” or set of wavelengths that it absorbs, it’s possible to figure out what an atmosphere is made of based on patterns in the transmission spectrum.
- This technique has been successful for many exoplanets, but not for most sub-Neptunes. “There have been very few atmospheric observations of sub-Neptune planets,” explains Eliza Kempton of the University of Maryland, College Park, who specializes in theoretical modeling of exoplanet atmospheres. “And most of those have been dissatisfying in that the spectra have not revealed much in the way of spectral features that would allow us to identify the gases in the atmosphere.”
- The issue seems to be aerosols, tiny particles and droplets that make up clouds or haze. These particles scatter starlight, eroding the prominent spectral peaks into subtle undulations and rendering the spectrum virtually useless in terms of determining gas composition.
- But with Webb, researchers are confident that a much clearer view of sub-Neptunes is on the horizon. Two observation programs co-led by Bean and Kempton and scheduled for the first year of Webb operations will use Webb’s uniquely powerful capabilities to probe two sub-Neptune-sized planets: GJ 1214 b, the archetype sub-Neptune; and TOI-421 b, a more recent discovery.
The Sub-Neptune Archetype: GJ 1214 b
- GJ 1214 b, a warm sub-Neptune orbiting a nearby red dwarf star, has been the subject of dozens of investigations. Its short orbital period, large size relative to its star, and comparative proximity to Earth make it easy (as exoplanets go) to observe effectively, while its status as the benchmark sub-Neptune – and, according to Bean, “the most mysterious exoplanet that we know of” – make it a worthy target of investigation.
- The team will use Webb’s Mid-Infrared Instrument (MIRI) to stare at the GJ 1214 system nearly continuously for nearly 50 hours as the planet completes a little more than one full orbit. They will then analyze the data in three different ways to narrow down the possible combinations of gases and aerosols that make up GJ 1214 b’s atmosphere.
- Transmission Spectroscopy: If molecules like water, methane, or ammonia are abundant, they should be obvious in the transmission spectrum. Mid-infrared light should not be scattered by aerosols in the same way as visible and near-infrared light.
- Thermal Emission Spectroscopy: Mid-infrared light emitted by the planet itself will provide information about the planet’s temperature and reflectivity, both of which are affected by the atmosphere. A planet surrounded by dark, sooty, light-absorbing haze, for example, will be warmer than one covered in bright, reflective clouds.
- Phase Curve Temperature Mapping: Although Webb will not be able to observe GJ 1214 b directly (the planet is too close to its star), it is sensitive enough to measure very subtle changes in the total amount of light from the system as the planet orbits the star. Researchers will use GJ 1214 b’s phase curve, a graph of brightness versus phase (i.e., how much of the planet’s day side is facing the telescope) to map the average temperature of the planet with longitude. This will provide additional information about the circulation and make-up of the atmosphere.
Hot Sub-Neptune TOI-421 b
- It’s not clear what the aerosols surrounding warm sub-Neptunes like GJ 1214 b are made of, but they could be similar to those that make up smog-like haze found on Saturn’s moon Titan. To test this hypothesis, the researchers decided to target TOI-421 b, a planet that is similar in size and density to GJ 1214 b, but is thought to be too hot for sooty haze to exist.
- Webb will observe TOI-421 b twice as it transits its star, once using the Near-Infrared Imager and Slitless Spectrograph (NIRISS) and again with the Near-Infrared Spectrograph (NIRSpec), to produce a complete near-infrared transmission spectrum of the planet. If the hypothesis is correct and TOI-421 b’s skies are clear, the spectrum can be used to measure the abundance of molecules like water, methane, and carbon dioxide. If it turns out that TOI-421 b has an aerosol problem after all, the team will use the data to better understand what those aerosols are made of.
- Kempton and Bean are confident that by probing elusive sub-Neptune atmospheres in a number of different ways with Webb, scientists will finally begin to understand not just these two specific objects, but an entire class of planets.
- Both the MIRI observations of GJ 1214 b and the NIRISS and NIRSpec observations of TOI-421 b will be conducted as part of Webb’s Cycle 1 General Observers program. General Observers programs were competitively selected using a dual-anonymous review system, the same system used to allocate time on Hubble.
![Figure 9: Illustration showing what exoplanet GJ 1214 b could look like based on current information. GJ 1214 b, a warm sub-Neptune-sized exoplanet roughly 48 light-years from Earth, is one of the most studied exoplanets in the galaxy. Previous spectroscopic observations indicate that the planet is shrouded in aerosols (clouds or haze), which have thus far made it impossible to determine the composition of gases that make up its thick atmosphere. This illustration is based on our knowledge of the planet and its host star, and predictions about the likely properties of the planet’s atmosphere. Spectroscopic data from Webb will help us better understand the gases and aerosols that make up the planet’s atmosphere. - GJ 1214 b is in between Earth and Neptune in terms of size (radius 2.74 times Earth), mass (8.2 times Earth), and density (2.2 times water). It orbits its star at a distance of only 0.015 astronomical unit (1.5% of the distance between Earth and the Sun), completing one orbit in 1.58 Earth-days. The star, GJ 1214, is cool red dwarf star with a radius of just 0.22 times that of the Sun, a mass of just 0.18 times the Sun, and a temperature of just 3,250 kelvins (around 2,975 degrees Celsius), about 2,500 degrees cooler than the Sun. [artwork credits: NASA, ESA, CSA, Dani Player (STScI)]](https://eoportal.org/ftp/satellite-missions/j/JWST-Feature_170222/JWST-Feature_Auto14.jpeg)
![Figure 10: Illustration comparing the sizes of sub-Neptune exoplanets TOI-421 b and GJ 1214 b to Earth and Neptune. Both TOI-421 b and GJ 1214 b are in between Earth and Neptune in terms of radius, mass, and density. The low densities of the two exoplanets indicates that they must have thick atmospheres. The planets are arranged from left to right in order of increasing radius and mass: - Image of Earth from the Deep Space Climate Observatory: Earth is a rocky planet with an average radius of roughly 6,370 kilometers, a mass of about 6 billion trillion metric tons, and a density 5.5 times that of water. - llustration of TOI-421 b: TOI-421 b is a hot sub-Neptune exoplanet with a radius 2.68 times Earth, a mass 7.2 times Earth, and a density 2.05 times water. - Illustration of GJ 1214 b: GJ 1214 b is a warm sub-Neptune exoplanet with a radius 2.74 times Earth, a mass 8.2 times Earth, and a density 2.2 times water. - Image of Neptune from Voyager 2: Neptune is an ice giant with a radius 3.88 times that of Earth (giving it a volume nearly 58 times Earth), a mass 17 times Earth, and a density of only 1.6 times water. The illustration shows the planets to scale in terms of radius, but not location in space or distance from their stars. While Earth and Neptune orbit the Sun, TOI-421 b orbits a sun-like star roughly 244 light-years away, and GJ 1214 b orbits a small red dwarf star about 48 light-years away. [credits: illustration: NASA, ESA, CSA, Dani Player (STScI)]](https://eoportal.org/ftp/satellite-missions/j/JWST-Feature_170222/JWST-Feature_Auto13.jpeg)
• October 27, 2021: In its first year of operations, NASA’s James Webb Space Telescope will join forces with a global collaborative effort to create an image of the area directly surrounding the supermassive black hole at the heart of our Milky Way galaxy. The Event Horizon Telescope (EHT) is famous for its first image of the “shadow” of the black hole at the core of galaxy M87, and it has now turned its efforts to the more complex environment of Sagittarius A*, the Milky Way’s supermassive black hole. While M87’s core presented a steady target, Sagittarius A* exhibits mysterious flickering flares on an hourly basis, which make the imaging process much more difficult. Webb will assist with its own infrared images of the black hole region, providing data about when flares are present that will be a valuable reference to the EHT team. 5)
- On isolated mountaintops across the planet, scientists await word that tonight is the night: The complex coordination between dozens of telescopes on the ground and in space is complete, the weather is clear, tech issues have been addressed—the metaphorical stars are aligned. It is time to look at the supermassive black hole at the heart of our Milky Way galaxy.
- This “scheduling Sudoku,” as the astronomers call it, happens each day of an observing campaign by the Event Horizon Telescope (EHT) collaboration, and they will soon have a new player to factor in; NASA’s James Webb Space Telescope will be joining the effort. During Webb’s first slate of observations, astronomers will use its infrared imaging power to address some of the unique and persistent challenges presented by the Milky Way’s black hole, named Sagittarius A* (Sgr A*; the asterisk is pronounced as “star”).
- In 2017, EHT used the combined imaging power of eight radio telescope facilities across the planet to capture the historic first view of the region immediately surrounding a supermassive black hole, in the galaxy M87. Sgr A* is closer but dimmer than M87’s black hole, and unique flickering flares in the material surrounding it alter the pattern of light on an hourly basis, presenting challenges for astronomers.
- “Our galaxy’s supermassive black hole is the only one known to have this kind of flaring, and while that has made capturing an image of the region very difficult, it also makes Sagittarius A* even more scientifically interesting,” said astronomer Farhad Yusef-Zadeh, a professor at Northwestern University and principal investigator on the Webb program to observe Sgr A*.
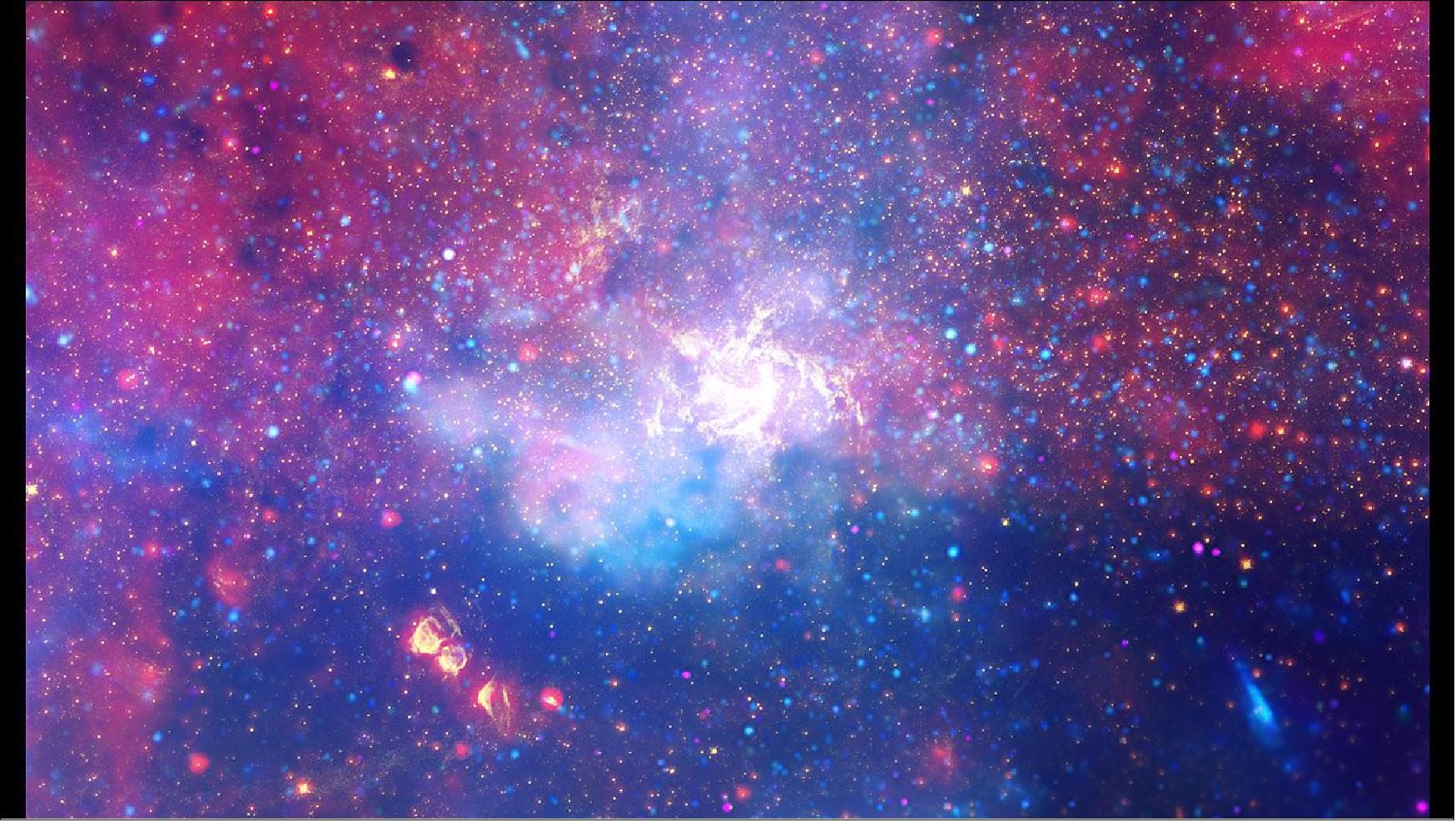
- The flares are due to the temporary but intense acceleration of particles around the black hole to much higher energies, with corresponding light emission. A huge advantage to observing Sgr A* with Webb is the capability of capturing data in two infrared wavelengths (F210M and F480M) simultaneously and continuously, from the telescope’s location beyond the Moon. Webb will have an uninterrupted view, observing cycles of flaring and calm that the EHT team can use for reference with their own data, resulting in a cleaner image.
- The source or mechanism that causes Sgr A*’s flares is highly debated. Answers as to how Sgr A*’s flares begin, peak, and dissipate could have far-reaching implications for the future study of black holes, as well as particle and plasma physics, and even flares from the Sun.
- “Black holes are just cool,” said Sera Markoff, an astronomer on the Webb Sgr A* research team and currently vice chairperson of EHT’s Science Council. “The reason that scientists and space agencies across the world put so much effort into studying black holes is because they are the most extreme environments in the known universe, where we can put our fundamental theories, like general relativity, to a practical test.”
- Black holes, predicted by Albert Einstein as part of his general theory of relativity, are in a sense the opposite of what their name implies—rather than an empty hole in space, black holes are the most dense, tightly-packed regions of matter known. A black hole’s gravitational field is so strong that it warps the fabric of space around itself, and any material that gets too close is bound there forever, along with any light the material emits. This is why black holes appear “black.” Any light detected by telescopes is not actually from the black hole itself, but the area surrounding it. Scientists call the ultimate inner edge of that light the event horizon, which is where the EHT collaboration gets its name.
- The EHT image of M87 was the first direct visual proof that Einstein’s black hole prediction was correct. Black holes continue to be a proving ground for Einstein’s theory, and scientists hope carefully scheduled multi-wavelength observations of Sgr A* by EHT, Webb, X-ray, and other observatories will narrow the margin of error on general relativity calculations, or perhaps point to new realms of physics we don’t currently understand.
- As exciting as the prospect of new understanding and/or new physics may be, both Markoff and Zadeh noted that this is only the beginning. “It’s a process. We will likely have more questions than answers at first,” Markoff said. The Sgr A* research team plans to apply for more time with Webb in future years, to witness additional flaring events and build up a knowledge base, determining patterns from seemingly random flares. Knowledge gained from studying Sgr A* will then be applied to other black holes, to learn what is fundamental to their nature versus what makes one black hole unique.
- So the stressful scheduling Sudoku will continue for some time, but the astronomers agree it’s worth the effort. “It’s the noblest thing humans can do, searching for truth,” Zadeh said. “It’s in our nature. We want to know how the universe works, because we are part of the universe. Black holes could hold clues to some of these big questions.”
- NASA’s Webb telescope will serve as the premier space science observatory for the next decade and explore every phase of cosmic history—from within our solar system to the most distant observable galaxies in the early universe, and everything in between. Webb will reveal new and unexpected discoveries, and help humanity understand the origins of the universe and our place in it. Webb is an international program led by NASA with its partners, ESA (European Space Agency) and the Canadian Space Agency.
• September 22, 2021: What was our Solar System like as it was forming billions of years ago? Over time, particles bumped into one another, building ever-larger rocks. Eventually, these rocks got big enough to form planets. We have some basic understanding of planet formation, but we don’t know the details – especially details about the solar system’s early chemical composition, and how it may have changed with time. And how did water make its way to Earth? While we can’t time travel to get the answers, we can detail how other planetary systems are forming right now – and learn quite a lot. Researchers will train one of Webb’s powerful instruments on the inner regions of 17 bright, actively forming planetary systems to begin to build an inventory of their contents. Element by element, they – along with researchers around the world – will be able to uncover what’s present and how the disks’ chemical makeup affects their contents, including planets that may be forming. 6)
- Planetary systems take millions of years to form, which introduces quite a challenge for astronomers. How do you identify which stage they are in, or categorize them? The best approach is to look at lots of examples and keep adding to the data we have – and NASA’s upcoming James Webb Space Telescope will be able to provide an infrared inventory. Researchers using Webb will observe 17 actively forming planetary systems. These particular systems were previously surveyed by the Atacama Large Millimeter/submillimeter Array (ALMA), the largest radio telescope in the world, for the Disk Substructures at High Angular Resolution Project (DSHARP).
- Webb will measure spectra that can reveal molecules in the inner regions of these protoplanetary disks, complementing the details ALMA has provided about the disks’ outer regions. These inner regions are where rocky, Earth-like planets can start to form, which is one reason why we want to know more about which molecules exist there.
- A research team led by Colette Salyk of Vassar College in Poughkeepsie, New York, and Klaus Pontoppidan of the Space Telescope Science Institute in Baltimore, Maryland, seek the details found in infrared light. “Once you switch to infrared light, specifically to Webb’s range in mid-infrared light, we will be sensitive to the most abundant molecules that carry common elements,” explained Pontoppidan.
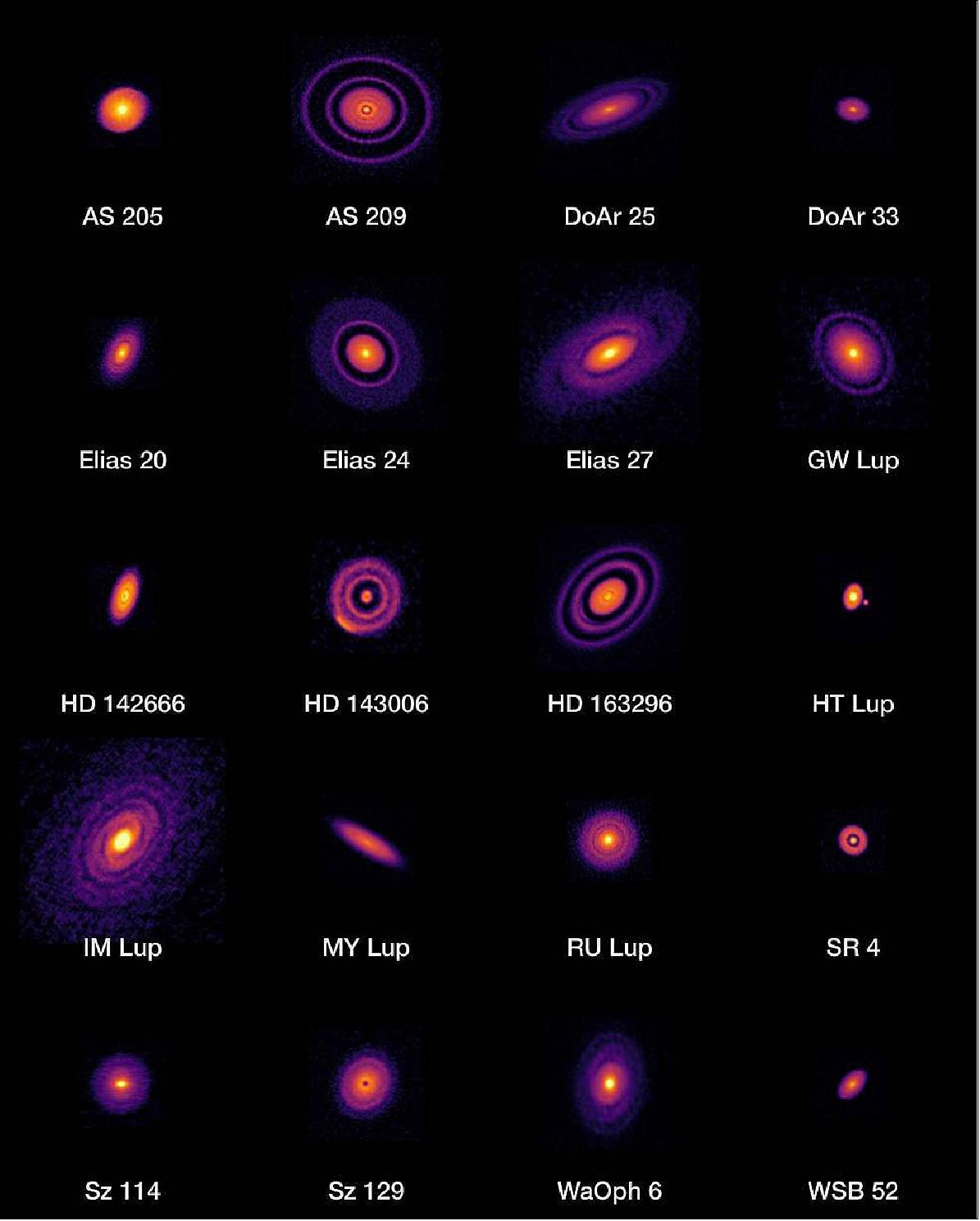
- Researchers will be able to assess the quantities of water, carbon monoxide, carbon dioxide, methane, and ammonia – among many other molecules – in each disk. Critically, they will be able to count the molecules that contain elements essential to life as we know it, including oxygen, carbon, and nitrogen. How? With spectroscopy: Webb will capture all the light emitted at the center of each protoplanetary disk as a spectrum, which produces a detailed pattern of colors based on the wavelengths of light emitted. Since every molecule imprints a unique pattern on the spectrum, researchers can identify which molecules are there and build inventories of the contents within each protoplanetary disk. The strength of these patterns also carries information about the temperature and quantity of each molecule.
- “Webb’s data will also help us identify where the molecules are within the overall system,” Salyk said. “If they’re hot, that implies they are closer to the star. If they’re cooler, they may be farther away.” This spatial information will help inform models that scientists build as they continue examining this program’s data.
- Knowing what’s in the inner regions of the disks has other benefits as well. Has water, for example, made it to this area, where habitable planets may be forming? “One of the things that’s really amazing about planets – change the chemistry just a little bit and you can get these dramatically different worlds,” Salyk continued. “That’s why we’re interested in the chemistry. We’re trying to figure out how the materials initially found in a system may end up as different types of planets.”
- If this sounds like a significant undertaking, do not worry – it will be a community effort. This is a Webb Treasury Program, which means that the data is released as soon as it’s taken to all astronomers, allowing everyone to immediately pull the data, begin assessing what’s what in each disk, and share their findings.
- “Webb’s infrared data will be intensively studied,” added co-investigator Ke Zhang of the University of Wisconsin–Madison. “We want the whole research community to be able to approach the data from different angles.”
Why the Up-Close Examination?
- Let’s step back, to see the forest for the trees. Imagine you are on a research boat off the coast of a distant terrain. This is the broadest view. If you were to land and disembark, you could begin counting how many trees there are and how many of each tree species. You could start identifying specific insects and birds and match up the sounds you heard offshore to the calls you hear under the treetops. This detailed cataloging is very similar to what Webb will empower researchers to do – but swap trees and animals for chemical elements.
- The protoplanetary disks in this program are very bright and relatively close to Earth, making them excellent targets to study. It’s why they were surveyed by ALMA. It’s also why researchers studied them with NASA’s Spitzer Space Telescope. These objects have only been studied in depth since 2003, making this a relatively newer field of research. There’s a lot Webb can add to what we know.
- The telescope’s Mid-Infrared Instrument (MIRI) provides many advantages. Webb’s location in space means that it can capture the full range of mid-infrared light (Earth’s atmosphere filters it out). Plus, its data will have high resolution, which will reveal many more lines and wiggles in the spectra that the researchers can use to tease out specific molecules.
- The researchers were also selective about the types of stars chosen for these observations. This sample includes stars that are about half the mass of the Sun to about twice the mass of the Sun. Why? The goal is to help researchers learn more about systems that may be like our own as it formed. “With this sample, we can start to determine if there are any common features between the disks’ properties and their inner chemistry,” Zhang continued. “Eventually, we want to be able to predict which types of systems are more likely to generate habitable planets.”
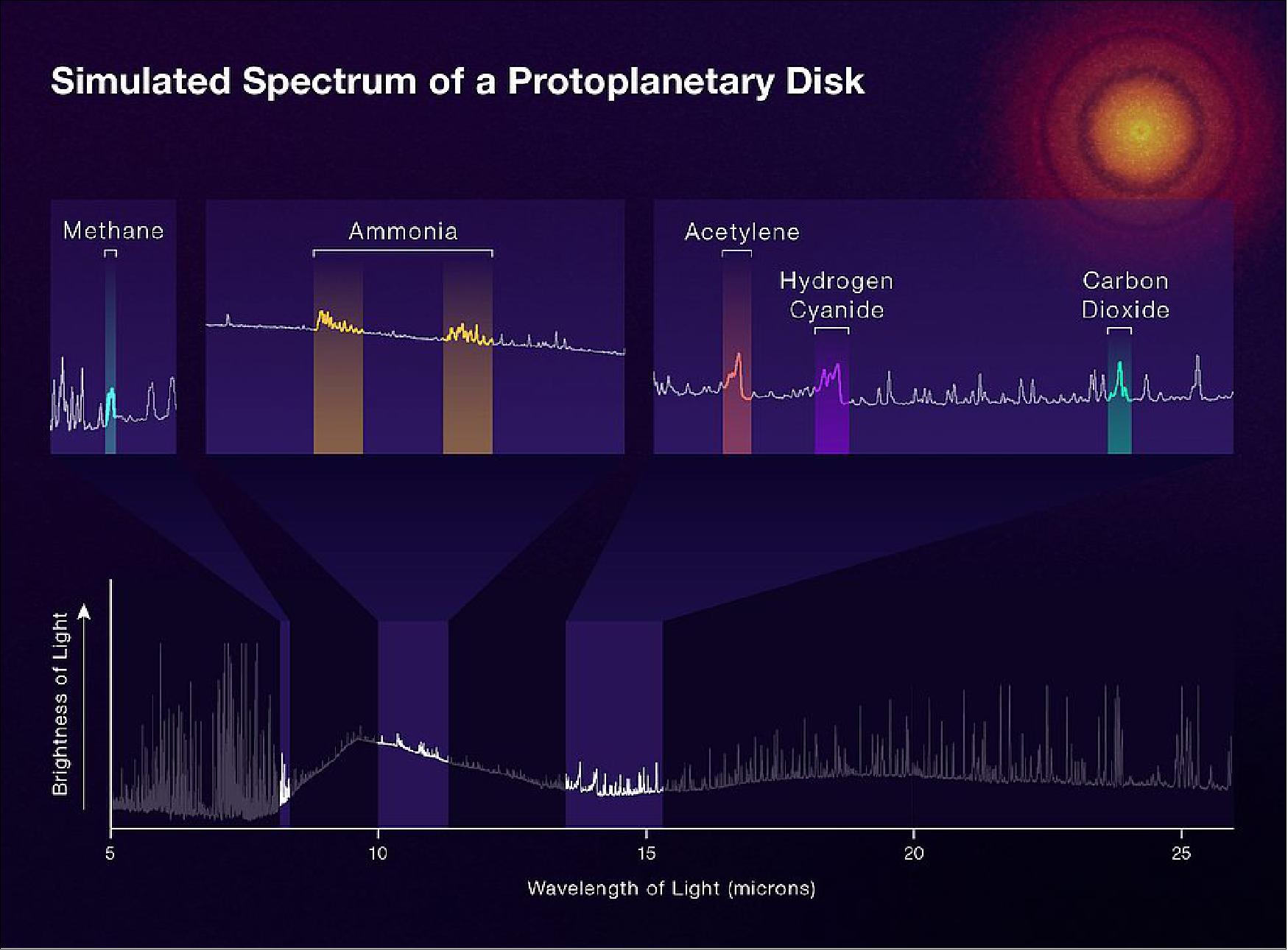
Beginning to Answer Big Questions
- This program may also help researchers begin to answer some classic questions: Are the forms taken by some of the most abundant elements found in protoplanetary disks, like carbon, nitrogen, and oxygen, “inherited” from the interstellar clouds that formed them? Or does the precise mix of chemicals change over time? “We think we can get to some of those answers by making inventories with Webb,” Pontoppidan explained. “It’s obviously a tremendous amount of work to do – and cannot be done only with these data – but I think we are going to make some major progress.”
- Thinking even more broadly about the incredibly rich spectra Webb will provide, Salyk added, “I’m hoping that we’ll see things that surprise us and then begin to study those serendipitous discoveries.”
- This research will be conducted as part of Webb General Observer (GO) programs, which are competitively selected using a dual-anonymous review system, the same system that is used to allocate time on the Hubble Space Telescope.
- The James Webb Space Telescope will be the world's premier space science observatory when it launches in 2021. Webb will solve mysteries in our solar system, look beyond to distant worlds around other stars, and probe the mysterious structures and origins of our universe and our place in it. Webb is an international program led by NASA with its partners, ESA (European Space Agency) and the Canadian Space Agency.
• August 18, 2021: Peering deeply into a huge patch of sky the size of three full Moons, NASA’s James Webb Space Telescope will undertake an ambitious program to study half a million galaxies. Called COSMOS-Webb, this survey is the largest project Webb will undertake during its first year. With more than 200 hours of observing time, it will build upon previous discoveries to make advances in three particular areas of study. These include revolutionizing our understanding of the Reionization Era; looking for early, fully evolved galaxies; and learning how dark matter evolved with galaxies’ stellar content. With its rapid public release of the data, this survey will be a primary legacy dataset from Webb for scientists worldwide studying galaxies beyond the Milky Way. 7)
- When NASA's James Webb Space Telescope begins science operations in 2022, one of its first tasks will be an ambitious program to map the earliest structures in the universe. Called COSMOS-Webb, this wide and deep survey of half-a-million galaxies is the largest project Webb will undertake during its first year.
- With more than 200 hours of observing time, COSMOS-Webb will survey a large patch of the sky—0.6 square degrees—with the Near-Infrared Camera (NIRCam). That's the size of three full moons. It will simultaneously map a smaller area with the Mid-Infrared Instrument (MIRI).
- "It's a large chunk of sky, which is pretty unique to the COSMOS-Webb program. Most Webb programs are drilling very deep, like pencil-beam surveys that are studying tiny patches of sky," explained Caitlin Casey, an assistant professor at the University of Texas at Austin and co-leader of the COSMOS-Webb program. "Because we're covering such a large area, we can look at large-scale structures at the dawn of galaxy formation. We will also look for some of the rarest galaxies that existed early on, as well as map the large-scale dark matter distribution of galaxies out to very early times."
- (Dark matter does not absorb, reflect, or emit light, so it cannot be seen directly. We know that dark matter exists because of the effect it has on objects that we can observe.)
- COSMOS-Webb will study half-a-million galaxies with multi-band, high-resolution, near-infrared imaging , and an unprecedented 32,000 galaxies in the mid-infrared . With its rapid public release of the data, this survey will be a primary legacy dataset from Webb for scientists worldwide studying galaxies beyond the Milky Way.
![Figure 14: This sea of galaxies is the complete, original COSMOS field from the Hubble Space Telescope’s Advanced Camera for Surveys (ACS). The full mosaic is a composite of 575 separate ACS images, where each ACS image is about one-tenth the diameter of the full Moon. The jagged edges of the outline are due to the separate images that make up the survey field [credits: Science: NASA, ESA, Anton M. Koekemoer (STScI), Nick Scoville (Caltech)]](https://eoportal.org/ftp/satellite-missions/j/JWST-Feature_170222/JWST-Feature_AutoF.jpeg)
Building on Hubble's Achievements
- The COSMOS survey began in 2002 as a Hubble program to image a much larger patch of sky, about the area of 10 full moons. From there, the collaboration snowballed to include most of the world's major telescopes on Earth and in space. Now COSMOS is a multi-wavelength survey that covers the entire spectrum from the X-ray through the radio.
- Because of its location on the sky, the COSMOS field is accessible to observatories around the world. Located on the celestial equator , it can be studied from both the northern and southern hemispheres, resulting in a rich and diverse treasury of data.
- "COSMOS has become the survey that a lot of extragalactic scientists go to in order to conduct their analyses because the data products are so widely available, and because it covers such a wide area of the sky," said Rochester Institute of Technology's Jeyhan Kartaltepe, assistant professor of physics and co-leader of the COSMOS-Webb program. "COSMOS-Webb is the next installment of that, where we're using Webb to extend our coverage in the near- and mid-infrared part of the spectrum, and therefore pushing out our horizon, how far away we're able to see."
- The ambitious COSMOS-Webb program will build upon previous discoveries to make advances in three particular areas of study, including: revolutionizing our understanding of the Reionization Era; looking for early, fully evolved galaxies; and learning how dark matter evolved with galaxies' stellar content.
Goal 1: Revolutionizing Our Understanding of the Reionization Era
- Soon after the big bang, the universe was completely dark. Stars and galaxies, which bathe the cosmos in light, had not yet formed. Instead, the universe consisted of a primordial soup of neutral hydrogen and helium atoms and invisible dark matter. This is called the cosmic dark ages.
- After several hundred million years, the first stars and galaxies emerged and provided energy to reionize the early universe. This energy ripped apart the hydrogen atoms that filled the universe, giving them an electric charge and ending the cosmic dark ages. This new era where the universe was flooded with light is called the Reionization Era.
- The first goal of COSMOS-Webb focuses on this epoch of reionization, which took place from 400,000 to 1 billion years after the big bang. Reionization likely happened in little pockets, not all at once. COSMOS-Webb will look for bubbles showing where the first pockets of the early universe were reionized. The team aims to map the scale of these reionization bubbles.
- ”Hubble has done a great job of finding handfuls of these galaxies out to early times, but we need thousands more galaxies to understand the reionization process," explained Casey.
- Scientists don't even know what kind of galaxies ushered in the Reionization Era, whether they're very massive or relatively low-mass systems. COSMOS-Webb will have a unique ability to find very massive, rare galaxies and see what their distribution is like in large-scale structures. So, are the galaxies responsible for reionization living in the equivalent of a cosmic metropolis, or are they mostly evenly distributed across space? Only a survey the size of COSMOS-Webb can help scientists to answer this.
Goal 2: Looking for Early, Fully Evolved Galaxies
- COSMOS-Webb will search for very early, fully evolved galaxies that shut down star birth in the first 2 billion years after the big bang. Hubble has found a handful of these galaxies, which challenge existing models about how the universe formed. Scientists struggle to explain how these galaxies could have old stars and not be forming any new stars so early in the history of the universe.
- With a large survey like COSMOS-Webb, the team will find many of these rare galaxies. They plan detailed studies of these galaxies to understand how they could have evolved so rapidly and turned off star formation so early.
Goal 3: Learning How Dark Matter Evolved with Galaxies' Stellar Content
- COSMOS-Webb will give scientists insight into how dark matter in galaxies has evolved with the galaxies' stellar content over the universe's lifetime.
- Galaxies are made of two types of matter: normal, luminous matter that we see in stars and other objects, and invisible dark matter, which is often more massive than the galaxy and can surround it in an extended halo. Those two kinds of matter are intertwined in galaxy formation and evolution. However, presently there's not much knowledge about how the dark matter mass in the halos of galaxies formed, and how that dark matter impacts the formation of the galaxies.
- COSMOS-Webb will shed light on this process by allowing scientists to directly measure these dark matter halos through "weak lensing." The gravity from any type of mass—whether it's dark or luminous—can serve as a lens to "bend" the light we see from more distant galaxies. Weak lensing distorts the apparent shape of background galaxies, so when a halo is located in front of other galaxies, scientists can directly measure the mass of the halo's dark matter.
- "For the first time, we'll be able to measure the relationship between the dark matter mass and the luminous mass of galaxies back to the first 2 billion years of cosmic time," said team member Anton Koekemoer, a research astronomer at the Space Telescope Science Institute in Baltimore, who helped design the program's observing strategy and is in charge of constructing all the images from the program. "That's a crucial epoch for us to try to understand how the galaxies' mass was first put in place, and how that's driven by the dark matter halos. And that can then feed indirectly into our understanding of galaxy formation."
Quickly Sharing Data with the Community
- COSMOS-Webb is a Treasury program, which by definition is designed to create datasets of lasting scientific value. Treasury Programs strive to solve multiple scientific problems with a single, coherent dataset. Data taken under a Treasury Program usually has no exclusive access period, enabling immediate analysis by other researchers.
- "As a Treasury Program, you are committing to quickly releasing your data and your data products to the community," explained Kartaltepe. "We're going to produce this community resource and make it publicly available so that the rest of the community can use it in their scientific analyses."
- Koekemoer added, "A Treasury Program commits to making publicly available all these science products so that anyone in the community, even at very small institutions, can have the same, equal access to the data products and then just do the science."
- COSMOS-Webb is a Cycle 1 General Observers program. General Observers programs were competitively selected using a dual-anonymous review system, the same system that is used to allocate time on Hubble.
- The James Webb Space Telescope will be the world's premier space science observatory when it launches in 2021. Webb will solve mysteries in our solar system, look beyond to distant worlds around other stars, and probe the mysterious structures and origins of our universe and our place in it. Webb is an international program led by NASA with its partners, ESA (European Space Agency) and the Canadian Space Agency.
• July 21, 2021: Researchers will use NASA’s upcoming James Webb Space Telescope to study Beta Pictoris, an intriguing young planetary system that sports at least two planets, a jumble of smaller, rocky bodies, and a dusty disk. Their goals include gaining a better understanding of the structures and properties of the dust to better interpret what is happening in the system. Since it’s only about 63 light-years away and chock full of dust, it appears bright in infrared light – and that means there is a lot of information for Webb to gather. 8)
- Beta Pictoris is the target of several planned Webb observing programs, including one led by Chris Stark of NASA’s Goddard Space Flight Center in Greenbelt, Maryland, and two led by Christine Chen of the Space Telescope Science Institute (STScI) in Baltimore, Maryland. Stark’s program will directly image the system after blocking the light of the star to gather a slew of new details about its dust. Chen’s programs will gather spectra, which spread light out like a rainbow to reveal which elements are present. All three observing programs will add critical details to what’s known about this nearby system.
First, a Review of What We Know
- Beta Pictoris has been regularly studied in radio, infrared, and visible light since the 1980s. The star itself is twice as massive as our sun and quite a bit hotter, but also significantly younger. (The Sun is 4.6 billion years old, but Beta Pictoris is approximately 20 million years old.) At this stage, the star is stable and hosts at least two planets, which are both far more massive than Jupiter. But this planetary system is remarkable because it is where the first exocomets (comets in other systems) were discovered. There are quite a lot of bodies zipping around this system!
- Like our own solar system, Beta Pictoris has a debris disk, which includes comets, asteroids, rocks of various sizes, and plenty of dust in all shapes that orbit the star. (A debris disk is far younger and can be more massive than our solar system’s Kuiper Belt, which begins near Neptune’s orbit and is where many short-period comets originate.)
- This outside ring of dust and debris is also where a lot of activity is happening. Pebbles and boulders could be colliding and breaking into far smaller pieces — sending out plenty of dust.
![Figure 15: A debris disk, which includes comets, asteroids, rocks of various sizes, and plenty of dust, orbits the star Beta Pictoris, which is blocked at the center of this 2012 image by a coronagraph aboard the Hubble Space Telescope. This is the visible-light view of the system. NASA’s James Webb Space Telescope will view Beta Pictoris in infrared light, both using its coronagraphs and capturing data known as spectra to allow researchers to learn significantly more about the gas and dust in the debris disk, which includes lots of smaller bodies like exocomets [image credit: NASA, ESA, Daniel Apai (University of Arizona), Glenn Schneider (University of Arizona)]](https://eoportal.org/ftp/satellite-missions/j/JWST-Feature_170222/JWST-Feature_AutoE.jpeg)
Scrutinizing This Planetary System
- Stark’s team will use Webb’s coronagraphs, which block the light of the star, to observe the faint portions of the debris disk that surround the entire system. “We know there are two massive planets around Beta Pictoris, and farther out there is a belt of small bodies that are colliding and fragmenting,” Stark explained. “But what’s in between? How similar is this system to our solar system? Can dust and water ice from the outer belt eventually make its way into the inner region of the system? Those are details we can help tease out with Webb.”
- Webb’s imagery will allow the researchers to study how the small dust grains interact with planets that are present in that system. Plus, Webb will detail all the fine dust that streams off these objects, permitting the researchers to infer the presence of larger rocky bodies and what their distribution is in the system. They’ll also carefully assess how the dust scatters light and reabsorbs and reemits light when it’s warm, allowing them to constrain what the dust is made of. By cataloging the specifics of Beta Pictoris, the researchers will also assess how similar this system is to our solar system, helping us understand if the contents of our solar system are unique.
- Isabel Rebollido, a team member and postdoctoral researcher at STScI, is already building complex models of Beta Pictoris. The first model combines existing data about the system, including radio, near-infrared, far-infrared, and visible light from both space- and ground-based observatories. In time, she will add Webb’s imagery to run a fuller analysis.
- The second model will feature only Webb’s data – and will be the first they explore. “Is the light Webb will observe symmetrical?” Rebollido asked. “Or are there ‘bumps’ of light here and there because there is an accumulation of dust? Webb is far more sensitive than any other space telescope and gives us a chance to look for this evidence, as well as water vapor where we know there’s gas.”
Dust as a Decoder Ring
- Think of the debris disk of Beta Pictoris as a very busy, elliptical highway – except one where there aren’t many traffic rules. Collisions between comets and larger rocks can produce fine dust particles that subsequently scatter throughout the system.
- “After planets, most of the mass in the Beta Pictoris system is thought to be in smaller planetesimals that we can’t directly observe,” Chen explained. “Fortunately, we can observe the dust left behind when planetesimals collide.”
- This dust is where Chen’s team will focus its research. What do the smallest dust grains look like? Are they compact or fluffy? What are they made of?
- “We’ll analyze Webb’s spectra to map the locations of dust and gas – and figure out what their detailed compositions are,” Chen explained. “Dust grains are ‘fingerprints’ of planetesimals we can’t see directly and can tell us about what these planetesimals are made of and how they formed.” For example, are the planetesimals ice-rich like comets in our solar system? Are there signs of high-speed collisions between rocky planetesimals? Clearly analyzing if grains in one region are more solid or fluffy than another will help the researchers understand what is happening to the dust, and map out the subtle differences in the dust in each region.
- “I’m looking forward to analyzing Webb’s data since it will provide exquisite detail,” added Cicero X. Lu, a team member and a fourth-year Ph.D. student at Johns Hopkins University in Baltimore. “Webb will allow us to identify more elements and pinpoint their precise structures.”
- In particular, there’s a cloud of carbon monoxide at the edge of the disk that greatly interests these researchers. It’s asymmetric and has an irregular, blobby side. One theory is that collisions released dust and gas from larger, icy bodies to form this cloud. Webb’s spectra will help them build scenarios that explain its origin.
The Reach of Infrared
- These research programs are only possible because Webb has been designed to provide crisp, high-resolution detail in infrared light. The observatory specializes in collecting infrared light – which travels through gas and dust – both with images and spectra. Webb also has another advantage – its position in space. Webb will not be hindered by Earth’s atmosphere, which filters out some types of light, including several infrared wavelength bands. This observatory will allow researchers to gather a more complete range of infrared light and data about Beta Pictoris for the first time.
- These studies will be conducted as part of Webb Guaranteed Time Observations (GTO) and General Observers (GO) programs. The GTO programs are led by scientists who helped develop the key hardware and software components or technical and inter-disciplinary knowledge for the observatory. GO programs are competitively selected using a dual-anonymous review system, the same system that is used to allocate time on the Hubble Space Telescope.
- The James Webb Space Telescope will be the world's premier space science observatory when it launches in 2021. Webb will solve mysteries in our solar system, look beyond to distant worlds around other stars, and probe the mysterious structures and origins of our universe and our place in it. Webb is an international program led by NASA with its partners, ESA (European Space Agency) and the Canadian Space Agency.
• June 23, 2021: Looking back in time, Webb will see quasars as they appeared billions of years ago. Outshining all the stars in their host galaxies combined, quasars are among the brightest objects in the universe. These brilliant, distant and active supermassive black holes shape the galaxies in which they reside. Shortly after its launch, scientists will use Webb to study six of the most far-flung and luminous quasars, along with their host galaxies, in the very young universe. They will examine what part quasars play in galaxy evolution during these early times. The team will also use the quasars to study the gas in the space between galaxies in the infant universe. Only with Webb’s extreme sensitivity to low levels of light and its superb angular resolution will this be possible. 9)
- Quasars are very bright, distant and active supermassive black holes that are millions to billions of times the mass of the Sun. Typically located at the centers of galaxies, they feed on infalling matter and unleash fantastic torrents of radiation. Among the brightest objects in the universe, a quasar’s light outshines that of all the stars in its host galaxy combined, and its jets and winds shape the galaxy in which it resides.
- Shortly after its launch later this year, a team of scientists will train NASA’s James Webb Space Telescope on six of the most distant and luminous quasars. They will study the properties of these quasars and their host galaxies, and how they are interconnected during the first stages of galaxy evolution in the very early universe. The team will also use the quasars to examine the gas in the space between galaxies, particularly during the period of cosmic reionization , which ended when the universe was very young. They will accomplish this using Webb’s extreme sensitivity to low levels of light and its superb angular resolution.
![Figure 16: Quasar Outflow Illustration. This is an artist's concept of a galaxy with a brilliant quasar at its center. A quasar is a very bright, distant and active supermassive black hole that is millions to billions of times the mass of the Sun. Among the brightest objects in the universe, a quasar’s light outshines that of all the stars in its host galaxy combined. Quasars feed on infalling matter and unleash torrents of winds and radiation, shaping the galaxies in which they reside. Using the unique capabilities of Webb, scientists will study six of the most distant and luminous quasars in the universe [image credit: ARTWORK: NASA, ESA, Joseph Olmsted (STScI)]](https://eoportal.org/ftp/satellite-missions/j/JWST-Feature_170222/JWST-Feature_AutoD.jpeg)
Webb: Visiting the Young Universe
- As Webb peers deep into the universe, it will actually look back in time. Light from these distant quasars began its journey to Webb when the universe was very young and took billions of years to arrive. We will see things as they were long ago, not as they are today.
- “All these quasars we are studying existed very early, when the universe was less than 800 million years old, or less than 6 percent of its current age. So these observations give us the opportunity to study galaxy evolution and supermassive black hole formation and evolution at these very early times,” explained team member Santiago Arribas, a research professor at the Department of Astrophysics of the Center for Astrobiology in Madrid, Spain. Arribas is also a member of Webb’s Near-Infrared Spectrograph (NIRSpec) Instrument Science Team.
- The light from these very distant objects has been stretched by the expansion of space. This is known as cosmological redshift . The farther the light has to travel, the more it is redshifted. In fact, the visible light emitted at the early universe is stretched so dramatically that it is shifted out into the infrared when it arrives to us. With its suite of infrared-tuned instruments, Webb is uniquely suited to studying this kind of light.
Studying Quasars, Their Host Galaxies and Environments, and Their Powerful Outflows
- The quasars the team will study are not only among the most distant in the universe, but also among the brightest. These quasars typically have the highest black hole masses, and they also have the highest accretion rates — the rates at which material falls into the black holes.
- “We’re interested in observing the most luminous quasars because the very high amount of energy that they’re generating down at their cores should lead to the largest impact on the host galaxy by the mechanisms such as quasar outflow and heating,” said Chris Willott, a research scientist at the Herzberg Astronomy and Astrophysics Research Centre of the National Research Council of Canada (NRC) in Victoria, British Columbia. Willott is also the Canadian Space Agency’s Webb project scientist. “We want to observe these quasars at the moment when they’re having the largest impact on their host galaxies.”
- An enormous amount of energy is liberated when matter is accreted by the supermassive black hole. This energy heats and pushes the surrounding gas outward, generating strong outflows that tear across interstellar space like a tsunami, wreaking havoc on the host galaxy.
- Outflows play an important role in galaxy evolution. Gas fuels the formation of stars, so when gas is removed due to outflows, the star-formation rate decreases. In some cases, outflows are so powerful and expel such large amounts of gas that they can completely halt star formation within the host galaxy. Scientists also think that outflows are the main mechanism by which gas, dust and elements are redistributed over large distances within the galaxy or can even be expelled into the space between galaxies – the intergalactic medium. This may provoke fundamental changes in the properties of both the host galaxy and the intergalactic medium.
Examining Properties of Intergalactic Space During the Era of Reionization
- More than 13 billion years ago, when the universe was very young, the view was far from clear. Neutral gas between galaxies made the universe opaque to some types of light. Over hundreds of millions of years, the neutral gas in the intergalactic medium became charged or ionized, making it transparent to ultraviolet light. This period is called the Era of Reionization. But what led to the reionization that created the “clear” conditions detected in much of the universe today? Webb will peer deep into space to gather more information about this major transition in the history of the universe. The observations will help us understand the Era of Reionization, which is one of the key frontiers in astrophysics.
- The team will use quasars as background light sources to study the gas between us and the quasar. That gas absorbs the quasar’s light at specific wavelengths. Through a technique called imaging spectroscopy, they will look for absorption lines in the intervening gas. The brighter the quasar is, the stronger those absorption line features will be in the spectrum. By determining whether the gas is neutral or ionized, scientists will learn how neutral the universe is and how much of this reionization process has occurred at that particular point in time.
- “If you want to study the universe, you need very bright background sources. A quasar is the perfect object in the distant universe, because it’s luminous enough that we can see it very well,” said team member Camilla Pacifici, who is affiliated with the Canadian Space Agency but works as an instrument scientist at the Space Telescope Science Institute in Baltimore. “We want to study the early universe because the universe evolves, and we want to know how it got started.”
- The team will analyze the light coming from the quasars with NIRSpec to look for what astronomers call “metals,” which are elements heavier than hydrogen and helium. These elements were formed in the first stars and the first galaxies and expelled by outflows. The gas moves out of the galaxies it was originally in and into the intergalactic medium. The team plans to measure the generation of these first “metals,” as well as the way they’re being pushed out into the intergalactic medium by these early outflows.
The Power of Webb
- Webb is an extremely sensitive telescope able to detect very low levels of light. This is important, because even though the quasars are intrinsically very bright, the ones this team is going to observe are among the most distant objects in the universe. In fact, they are so distant that the signals Webb will receive are very, very low. Only with Webb’s exquisite sensitivity can this science be accomplished. Webb also provides excellent angular resolution, making it possible to disentangle the light of the quasar from its host galaxy.
- The quasar programs described here are Guaranteed Time Observations involving the spectroscopic capabilities of NIRSpec.
- he James Webb Space Telescope will be the world's premier space science observatory when it launches in 2021. Webb will solve mysteries in our solar system, look beyond to distant worlds around other stars, and probe the mysterious structures and origins of our universe and our place in it. Webb is an international program led by NASA with its partners, ESA (European Space Agency) and the Canadian Space Agency.
• April 21, 2021: Although more than 4,000 planets have been discovered around other stars, they don’t represent the wide diversity of possible alien worlds. Most of the exoplanets detected so far are so-called “star huggers”: they orbit so close to their host stars that they complete an orbit in days or weeks. These are the easiest to find with current detection techniques. 10)
- But there’s a vast, mostly uncharted landscape to hunt for exoplanets in more distant orbits. Astronomers have only begun to explore this frontier. The planets are far enough away from their stars that telescopes equipped with masks to block out a star’s blinding glare can see the planets directly. The easiest planets to spot are hot, newly formed worlds. They are young enough that they still glow in infrared light with the heat from their formation.
- This outer realm of exoplanetary systems is an ideal hunting ground for NASA’s upcoming James Webb Space Telescope. Webb will probe the atmospheres of nearby known exoplanets, such as HR 8799 and 51 Eridani b, at infrared wavelengths. Webb also will hunt for other distant worlds—possibly down to Saturn-size—on the outskirts of planetary systems that cannot be detected with ground-based telescopes.
- Before planets around other stars were first discovered in the 1990s, these far-flung exotic worlds lived only in the imagination of science fiction writers.
- But even their creative minds could not have conceived of the variety of worlds astronomers have uncovered. Many of these worlds, called exoplanets, are vastly different from our solar system’s family of planets. They range from star-hugging “hot Jupiters” to oversized rocky planets dubbed “super Earths.” Our universe apparently is stranger than fiction.
- That’s why astronomers have identified most of the more than 4,000 exoplanets found so far using indirect techniques, such as through a star’s slight wobble or its unexpected dimming as a planet passes in front of it, blocking some of the starlight.
- These techniques work best, however, for planets orbiting close to their stars, where astronomers can detect changes over weeks or even days as the planet completes its racetrack orbit. But finding only star-skimming planets doesn’t provide astronomers with a comprehensive picture of all the possible worlds in star systems.
- Another technique researchers use in the hunt for exoplanets, which are planets orbiting other stars, is one that focuses on planets that are farther away from a star’s blinding glare. Scientists, using specialized imaging techniques that block out the glare from the star, have uncovered young exoplanets that are so hot they glow in infrared light. In this way, some exoplanets can be directly seen and studied.
![Figure 17: Left: This is an image of the star HR 8799 taken by Hubble's Near Infrared Camera and Multi-Object Spectrometer (NICMOS) in 1998. A mask within the camera (coronagraph) blocks most of the light from the star. Astronomers also used software to digitally subtract more starlight. Nevertheless, scattered light from HR 8799 dominates the image, obscuring the four faint planets. The exoplanets were discovered in 2007, 2008, and 2010 in near-infrared ground-based images taken with the W.M. Keck Observatory in Hawaii and the Gemini North telescope in Chile. The system is 133 light-years from Earth. Right: A re-analysis of NICMOS data in 2011 uncovered three of the exoplanets, which were not seen in the 1998 images. Sophisticated software processing of the NICMOS data removes most of the scattered starlight to reveal the three planets orbiting HR 8799. Astronomers used this decade-old image to calculate the planets’ orbits. The fourth, innermost planet cannot be seen because it is on the edge of the NICMOS coronagraphic spot that blocks the light from the central star. Webb will probe the planets’ atmospheres at infrared wavelengths astronomers have rarely used to image distant worlds. Webb will also hunt for other distant worlds—possibly down to Saturn-size—on the outskirts of planetary systems that cannot be detected with ground-based telescopes [image credit: NASA, ESA, Rémi Soummer (STScI)]](https://eoportal.org/ftp/satellite-missions/j/JWST-Feature_170222/JWST-Feature_AutoC.jpeg)
- NASA’s upcoming James Webb Space Telescope will help astronomers probe farther into this bold new frontier. Webb, like some ground-based telescopes, is equipped with special optical systems called coronagraphs, which use masks designed to block out as much starlight as possible to study faint exoplanets and to uncover new worlds.
- Two targets early in Webb's mission are the planetary systems 51 Eridani and HR 8799. Out of the few dozen directly imaged planets, astronomers plan to use Webb to analyze in detail the systems that are closest to Earth and have planets at the widest separations from their stars. This means that they appear far enough away from a star’s glare to be directly observed. The HR 8799 system resides 133 light-years and 51 Eridani 96 light-years from Earth.
Webb's Planetary Targets
- Two observing programs early in Webb's mission combine the spectroscopic capabilities of the Near Infrared Spectrograph (NIRSpec) and the imaging of the Near Infrared Camera (NIRCam) and Mid-Infrared Instrument (MIRI ) to study the four giant planets in the HR 8799 system. In a third program, researchers will use NIRCam to analyze the giant planet in 51 Eridani.
- The four giant planets in the HR 8799 system are each roughly 10 Jupiter masses. They orbit more than 14 billion miles from a star that is slightly more massive than the Sun. The giant planet in 51 Eridani is twice the mass of Jupiter and orbits about 11 billion miles from a Sun-like star. Both planetary systems have orbits oriented face-on toward Earth. This orientation gives astronomers a unique opportunity to get a bird's-eye view down on top of the systems, like looking at the concentric rings on an archery target.
- Many exoplanets found in the outer orbits of their stars are vastly different from our solar system planets. Most of the exoplanets discovered in this outer region, including those in HR 8799, are between 5 and 10 Jupiter masses, making them the most massive planets ever found to date.
- These outer exoplanets are relatively young, from tens of millions to hundreds of millions of years old—much younger than our solar system’s 4.5 billion years. So they’re still glowing with heat from their formation. The images of these exoplanets are essentially baby pictures, revealing planets in their youth.
- Webb will probe into the mid-infrared, a wavelength range astronomers have rarely used before to image distant worlds. This infrared “window” is difficult to observe from the ground because of thermal emission from—and absorption in—Earth’s atmosphere.
- “Webb’s strong point is the uninhibited light coming through space in the mid-infrared range,” said Klaus Hodapp of the University of Hawaii in Hilo, lead investigator of the NIRSpec observations of the HR 8799 system. “Earth’s atmosphere is pretty difficult to work through. The major absorption molecules in our own atmosphere prevent us from seeing interesting features in planets.”
- The mid-infrared “is the region where Webb really will make seminal contributions to understanding what are the particular molecules, what are the properties of the atmosphere that we hope to find which we don’t really get just from the shorter, near-infrared wavelengths,” said Charles Beichman of NASA’s Jet Propulsion Laboratory in Pasadena, California, lead investigator of the NIRCam and MIRI observations of the HR 8799 system. “We’ll build on what the ground-based observatories have done, but the goal is to expand on that in a way that would be impossible without Webb.”
How Do Planets Form?
- One of the researchers’ main goals in both systems is to use Webb to help determine how the exoplanets formed. Were they created through a buildup of material in the disk surrounding the star, enriched in heavy elements such as carbon, just as Jupiter probably did? Or, did they form from the collapse of a hydrogen cloud, like a star, and become smaller under the relentless pull of gravity?
- Atmospheric makeup can provide clues to a planet’s birth. “One of the things we’d like to understand is the ratio of the elements that have gone into the formation of these planets,” Beichman said. “In particular, carbon versus oxygen tells you quite a lot about where the gas that formed the planet comes from. Did it come from a disk that accreted a lot of the heavier elements or did it come from the interstellar medium ? So it’s what we call the carbon-to-oxygen ratio that is quite indicative of formation mechanisms.”
- To answer these questions, the researchers will use Webb to probe deeper into the exoplanets’ atmospheres. NIRCam, for example, will measure the atmospheric fingerprints of elements like methane. It also will look at cloud features and the temperatures of these planets. “We already have a lot of information at these near-infrared wavelengths from ground-based facilities,” said Marshall Perrin of the Space Telescope Science Institute in Baltimore, Maryland, lead investigator of NIRCam observations of 51 Eridani b. “But the data from Webb will be much more precise, much more sensitive. We’ll have a more complete set of wavelengths, including filling in gaps where you can’t get those wavelengths from the ground.”
- The astronomers will also use Webb and its superb sensitivity to hunt for less-massive planets far from their star. “From ground-based observations, we know that these massive planets are relatively rare,” Perrin said. “But we also know that for the inner parts of systems, lower-mass planets are dramatically more common than larger-mass planets. So the question is, does it also hold true for these further separations out?” Beichman added, “Webb’s operation in the cold environment of space allows a search for fainter, smaller planets, impossible to detect from the ground.”
- Another goal is understanding how the myriad planetary systems discovered so far were created.
- “I think what we are finding is that there is a huge diversity in solar systems,” Perrin said. “You have systems where you have these hot Jupiter planets in very close orbits. You have systems where you don’t. You have systems where you have a 10-Jupiter-mass planet and ones in which you have nothing more massive than several Earths. We ultimately want to understand how the diversity of planetary system formation depends on the environment of the star, the mass of the star, all sorts of other things and eventually through these population-level studies, we hope to place our own solar system in context.”
- The NIRSpec spectroscopic observations of HR 8799 and the NIRCam observations of 51 Eridani are part of the Guaranteed Time Observations programs that will be conducted shortly after Webb’s launch later this year. The NIRCam and MIRI observations of HR 8799 is a collaboration of two instrument teams and is also part of the Guaranteed Time Observations program.
- The James Webb Space Telescope will be the world's premier space science observatory when it launches in 2021. Webb will solve mysteries in our solar system, look beyond to distant worlds around other stars, and probe the mysterious structures and origins of our universe and our place in it. Webb is an international program led by NASA with its partners, ESA (European Space Agency) and the Canadian Space Agency.
• March 17, 2021: As technology has improved over the centuries, so have astronomers' observations of nearby galaxy Centaurus A. They have peeled back its layers like an onion to discover that its wobbly shape is the result of two galaxies that merged more than 100 million years ago. It also has an active supermassive black hole, known as an active galactic nucleus, at its heart that periodically sends out twin jets. Despite these advancements, Centaurus A's dusty core is still quite mysterious. Webb's high-resolution infrared data will allow a research team to very precisely reveal all that lies at the center. 11)
- Centaurus A is a giant of a galaxy, but its appearances in telescope observations can be deceiving. Dark dust lanes and young blue star clusters, which crisscross its central region, are apparent in ultraviolet, visible, and near-infrared light, painting a fairly subdued landscape. But by switching to X-ray and radio light views, a far more raucous scene begins to unfold: From the core of the misshapen elliptical galaxy, spectacular jets of material have erupted from its active supermassive black hole – known as an active galactic nucleus – sending material into space well beyond the galaxy's limits.
- What, precisely, is happening at its core to cause all this activity? Upcoming observations led by Nora Lützgendorf and Macarena García Marín of the European Space Agency using NASA's James Webb Space Telescope will allow researchers to peer through its dusty core in high resolution for the first time to begin to answer these questions.
- "There's so much going on in Centaurus A," explains Lützgendorf. "The galaxy's gas, disk, and stars all move under the influence of its central supermassive black hole. Since the galaxy is so close to us, we'll be able to use Webb to create two-dimensional maps to see how the gas and stars move in its central region, how they are influenced by the jets from its active galactic nucleus, and ultimately better characterize the mass of its black hole."
![Figure 18: Centaurus A sports a warped central disk of gas and dust, which is evidence of a past collision and merger with another galaxy. It also has an active galactic nucleus that periodically emits jets. It is the fifth brightest galaxy in the sky and only about 13 million light-years away from Earth, making it an ideal target to study an active galactic nucleus – a supermassive black hole emitting jets and winds – with NASA's upcoming James Webb Space Telescope [image credit: X-ray: NASA/CXC/SAO; Optical: Rolf Olsen; Infrared: NASA /JPL-Caltech; Radio: NRAO/AUI/NSF/Univ.Hertfordshire/M.Hardcastle]](https://eoportal.org/ftp/satellite-missions/j/JWST-Feature_170222/JWST-Feature_AutoB.jpeg)
A Quick Look Back
- Let's hit "rewind" to review a bit of what is already known about Centaurus A. It's well studied because it's relatively nearby – about 13 million light-years away – which means we can clearly resolve the full galaxy. The first record of it was logged in the mid-1800s, but astronomers lost interest until the 1950s because the galaxy appeared to be a quiet, if misshapen, elliptical galaxy. Once researchers were able to begin observing with radio telescopes in the 1940s and '50s, Centaurus A became radically more interesting – and its jets came into view. In 1954, researchers found that Centaurus A is the result of two galaxies that merged, which was later estimated to have occurred 100 million years ago.
- With more observations in the early 2000s, researchers estimated that about 10 million years ago, its active galactic nucleus shot out twin jets in opposite directions. When examined across the electromagnetic spectrum, from X-ray to radio light, it's clear there is far more to this story that we still have to learn.
- "Multi-wavelength studies of any galaxy are like the layers of an onion. Each wavelength shows you something different," said Marín. "With Webb's near- and mid-infrared instruments, we'll see far colder gas and dust than in previous observations, and learn much more about the environment at the center of the galaxy."
Visualizing Webb's Data
- The team led by Lützgendorf and Marín will observe Centaurus A not only by taking images with Webb, but by gathering data known as spectra, which spread out light into its component wavelengths like a rainbow. Webb's spectra will reveal high-resolution information about the temperatures, speeds, and compositions of the material at the center of the galaxy.
- In particular, Webb's Near Infrared Spectrograph (NIRSpec) and Mid-Infrared Instrument (MIRI) will provide the research team with a combination of data: an image plus a spectrum from within each pixel of that image. This will allow the researchers to build intricate 2D maps from the spectra that will help them identify what's happening behind the veil of dust at the center – and analyze it from many angles in depth.
- Compare this style of modeling to the analysis of a garden. In the same way botanists classify plants based on specific sets of features, these researchers will classify spectra from Webb's MIRI to construct "gardens" or models. "If you take a snapshot of a garden from a great distance away," Marín explained, "You will see something green, but with Webb, we will be able to see individual leaves and flowers, their stems, and maybe the soil underneath."
- As the research team digs into the spectra, they'll build maps from individual parts of the garden, comparing one spectrum to another nearby spectrum. This is analogous to determining which parts contain which plant species based on comparisons of "stems," "leaves," and "flowers" as they go.
- "When it comes to spectral analysis, we conduct many comparisons," Marín continued. "If I compare two spectra in this region, maybe I will find that what was observed contains a prominent population of young stars. Or confirm which areas are both dusty and heated. Or maybe we will identify emission coming from the active galactic nucleus."
- In other words, the "ecosystem" of spectra has many levels, which will allow the team to better define precisely what is present and where it is – which is made possible by Webb's specialized infrared instruments. And, since these studies will build on many that came before, the researchers will be able to confirm, refine, or break new ground by identifying new features.
![Figure 19: Centaurus A's dusty core is apparent in visible light, but its jets are best viewed in X-ray and radio light. With upcoming observations from NASA's James Webb Space Telescope in infrared light, researchers hope to better pinpoint the mass of the galaxy's central supermassive black hole as well as evidence that shows where the jets were ejected [image credit: X-ray: NASA/CXC/SAO; Optical: Rolf Olsen; Infrared: NASA/JPL-Caltech; Radio: NRAO/AUI/NSF/Univ.Hertfordshire/M.Hardcastle]](https://eoportal.org/ftp/satellite-missions/j/JWST-Feature_170222/JWST-Feature_AutoA.jpeg)
Weighing the Black Hole in Centaurus A
- The combination of images and spectra provided by NIRSpec and MIRI will allow the team to create very high-resolution maps of the speeds of the gas and stars at the center of Centaurus A. "We plan to use these maps to model how the entire disk at the center of the galaxy moves to more precisely determine the black hole's mass," Lützgendorf explains.
- Since researchers understand how the gravity of a black hole governs the rotation of nearby gas, they can use the Webb data to weigh the black hole in Centaurus A. With a more complete set of infrared data, they will also determine if different parts of the gas are all behaving as anticipated. "I'm looking forward to fully filling out our data," Lützgendorf said. "I hope to see how the ionized gas behaves and twirls, and where we see the jets."
- The researchers are also hoping to break new ground. "It's possible we'll find things we haven't considered yet," Lützgendorf explains. "In some aspects, we'll be covering completely new territory with Webb." Marín wholeheartedly agrees, and adds that building on a wealth of existing data is invaluable. "The most exciting aspects about these observations is the potential for new discoveries," she said. "I think we might find something that makes us look back to other data and reinterpret what was seen earlier."
- These studies of Centaurus A will be conducted as part of Gillian Wright and Pierre Ferruit's joint MIRI and NIRSpec Guaranteed Time Observations programs. All of Webb's data will ultimately be stored in the publicly accessible Barbara A. Mikulski Archive for Space Telescopes (MAST) at the Space Telescope Science Institute in Baltimore.
- The James Webb Space Telescope will be the world's premier space science observatory when it launches in 2021. Webb will solve mysteries in our solar system, look beyond to distant worlds around other stars, and probe the mysterious structures and origins of our universe and our place in it. Webb is an international program led by NASA with its partners, ESA (European Space Agency) and the Canadian Space Agency.
• February 22, 2021: Early observations of stars in the local universe will pave the way for years of discovery across a range of science topics. 12)
- The combination of high resolution and infrared-detecting instruments on NASA's upcoming James Webb Space Telescope will reveal stars that are currently hidden even from the powerful Hubble Space Telescope. The wealth of additional star data will allow astronomers to investigate a range of questions, from star birth to star death, to the universe's elusive expansion rate. Early observations with Webb will demonstrate its ability to distinguish the individual light of stars in the local universe in a range of environments and provide astronomers with tools for making the most of Webb's powerful capabilities.
- "NASA's Hubble and Spitzer space telescopes have been transformative, opening the door to the infrared universe, beyond the realm of red visible light. Webb is a natural evolution of those missions, combining Spitzer's view of the infrared universe with Hubble's sensitivity and resolution," says Daniel Weisz of the University of California, Berkeley, the principal investigator on Webb's early release science (ERS) program on resolved populations of stars.
- Webb's ability to resolve individual stars that are shrouded behind gas and dust in visible light will be applicable to many areas of astronomical research. The goals of this ERS program are to demonstrate Webb's capabilities in the local universe and create free, open-source data analysis programs for astronomers to make the best use of the observatory as quickly as possible. Data from the ERS programs will be available to other astronomers immediately, and archived for future research via the Barbara A. Mikulski Archive for Space Telescopes (MAST).
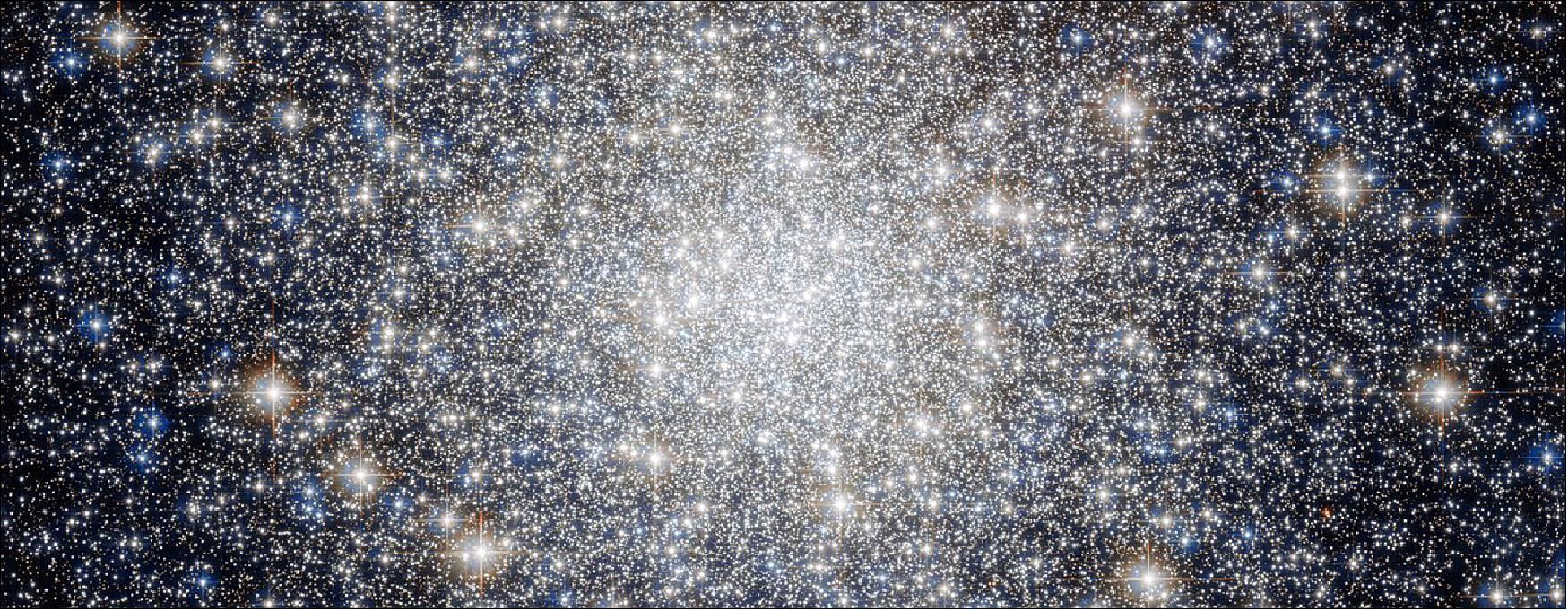
Insight into Dark Energy
- Webb's ability to pick out details for more individual stars than we have seen before will improve distance measurements to nearby galaxies, which Weisz says will be crucial to one of the biggest mysteries of modern-day astronomy: How fast is the universe expanding? A phenomenon called dark energy seems to be driving this expansion. Various methods for calculating the expansion rate have resulted in different answers—discrepancies astronomers hope Webb's data can help reconcile.
- "In order to do any of this science, calculating distances and then the universe's expansion rate, we need to be able to extract the light of individual stars from Webb images," Weisz says. "Our ERS program team will develop software that empowers the community to make those types of measurements."
The Stellar Lifecycle
- Seeing more stars will mean more insight into their lifecycle. Webb will provide new views of the full range of stages in a star's life, from formation to death.
- "Right now we are effectively limited to studying star formation in our own Milky Way galaxy, but with Webb's infrared capabilities we can see through the dusty cocoons that shelter forming protostars in other galaxies—like Andromeda, which is more metal-rich—and see how stars form in a very different environment," Weisz says.
- Astronomer Martha Boyer, also on this observing-program team, is interested in the insights Webb will provide toward the end of the stellar lifecycle, when stars become bloated, red, and dusty.
- "NASA's Spitzer Space Telescope showed us that dusty, evolved stars exist even in very primitive galaxies where they weren't expected, and now with Webb we will be able to characterize them and learn how our models of the star lifecycle line up with real observations," says Boyer, an instrument scientist on Webb's Near Infrared Camera (NIRCam) team at the Space Telescope Science Institute in Baltimore, Maryland.
The Early Universe via the Local Neighborhood
- Resolving and studying individual stars is necessary for understanding the bigger picture of how galaxies formed and function. Astronomers then can ask even bigger questions of how galaxies have evolved over time and space, from the distant, early universe to the Local Group—a collection of more than 20 nearby galaxies to which our galaxy belongs. Weisz explains that even though this observing program will be looking locally, there is evidence of the early universe to be discovered.
- "We will have Webb study a nearby, ultra-faint dwarf galaxy, a remnant of the first seed-galaxies to form in the universe, some of which eventually merged to form larger galaxies like the Milky Way," Weisz says. "At great distances these types of galaxies are too faint for even Webb to see directly, but small, local dwarf galaxies will show us what they were like billions of years ago."
- "We really need to understand the local universe in order to understand all of the universe," Boyer says. "The Local Group of galaxies is a sort of laboratory, where we can study galaxies in detail—every single component. In distant galaxies we can't resolve much detail, so we don’t know exactly what's going on. A major step towards understanding distant or early galaxies is to study this collection of galaxies that is within our reach."
- As the Webb mission progresses, Boyer and Weisz expect that astronomers will use the tools their team develops in unexpected ways. They emphasize that developing the program was an effort of the entire local-universe astronomy community, and they plan to continue that collaboration once the data come in. Their observing-program team plans to host a workshop to go over the results of the program with other astronomers and tweak the software they’ve developed, all with the goal of assisting members of the astronomy community in applying for time to use Webb for their research.
- "I think that is really important—the idea of working together to achieve big science, as opposed to a lot of us trying to compete," Weisz says.
- The James Webb Space Telescope will be the world's premier space science observatory when it launches in 2021. Webb will solve mysteries in our solar system, look beyond to distant worlds around other stars, and probe the mysterious structures and origins of our universe and our place in it. Webb is an international program led by NASA with its partners, ESA (European Space Agency) and the Canadian Space Agency.
• December 16, 2020: Forming solar systems are a bit unkempt—a profusion of gas and dust, and an array of molecules orbits a star that's still gathering material. Over time, some of the dust bumps into one another, forming larger and larger particles until planets begin forming. Researchers know quite a bit about the outer regions of these planet-forming disks, but the inner areas, extending about as far as Saturn in our solar system, and the forming planets they may contain aren't yet well studied. NASA's James Webb Space Telescope's specialization in mid-infrared light, specifically its collection of data known as spectra, will help researchers model what's going on at the centers of these systems with unprecedented detail. 13)
- We live in a mature solar system—eight planets and several dwarf planets (like Pluto) have formed, the latter within the rock- and debris-filled region known as the Kuiper Belt. If we could turn back time, what would we see as our solar system formed? While we can’t answer this question directly, researchers can study other systems that are actively forming—along with gas and dust that encircles their still-forming stars—to learn about this process.
- A team led by Dr. Thomas Henning of the Max Planck Institute for Astronomy in Heidelberg, Germany, will employ NASA's upcoming James Webb Space Telescope to survey more than 50 planet-forming disks in various stages of growth to determine which molecules are present and ideally pinpoint similarities, helping to shape what we know about how solar systems assemble.
- Their research with Webb will specifically focus on the inner disks of relatively nearby, forming systems. Although information about these regions has been obtained by previous telescopes, none match Webb's sensitivity, which means many more details will pour in for the first time. Plus, Webb's space-based location about a million miles (1.5 million kilometers) from Earth will give it an unobstructed view of its targets. "Webb will provide unique data that we can't get any other way," said Inga Kamp of the Kapteyn Astronomical Institute of the University of Groningen in the Netherlands. "Its observations will provide molecular inventories of the inner disks of these solar systems."
- This research program will primarily gather data in the form of spectra. Spectra are like rainbows—they spread out light into its component wavelengths to reveal high-resolution information about the temperatures, speeds, and compositions of the gas and dust. This incredibly rich information will allow the researchers to construct far more detailed models of what is present in the inner disks—and where. "If you apply a model to these spectra, you can find out where molecules are located and what their temperatures are," Henning explained.
- These observations will be incredibly valuable in helping the researchers pinpoint similarities and differences among these planet-forming disks, which are also known as protoplanetary disks. "What can we learn from spectroscopy that we can't learn from imaging? Everything!" Ewine van Dishoeck of Leiden University in the Netherlands exclaimed. "One spectrum is worth a thousand images."
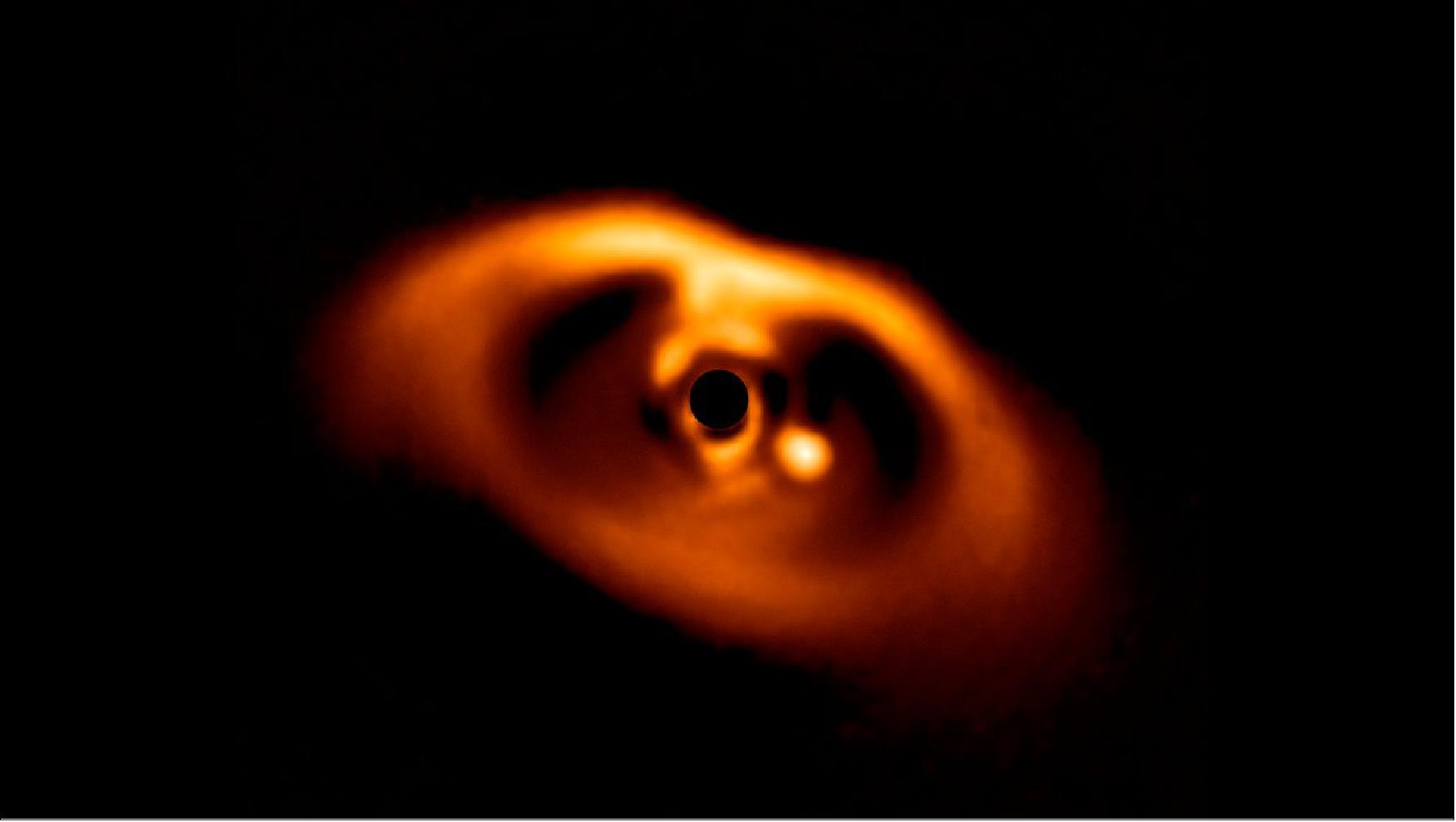
A 'Mountain' of New Data
- Researchers have long studied protoplanetary disks in a variety of wavelengths of light, from radio to near-infrared. Some of the team's existing data are from the Atacama Large Millimeter/submillimeter Array (ALMA) in Chile, which collects radio light. ALMA excels at constructing images of the outer disks. If you were to compare the span of their outer disks to the size of our Solar System, this region is past Saturn's orbit. Webb's data will complete the picture by helping researchers model the inner disks.
- Some data already exist about these inner disks—NASA's retired Spitzer Space Telescope served as a pathfinder—but Webb's sensitivity and resolution are required to identify the precise quantities of each molecule as well as the elemental compositions of the gas with its data, known as spectra. "What used to be a very blurry peak in the spectrum will consist of hundreds if not thousands of detailed spectral lines," van Dishoeck said.
- Webb's specialty in mid-infrared light is particularly important. It will enable researchers to identify the "fingerprints" of molecules like water, carbon dioxide, methane, and ammonia—which can't be identified with any other existing instruments. The observatory will also determine how starlight impacts the chemistry and physical structures of the disks.
- Protoplanetary disks are complex systems. As they form, their mix of gas and dust is distributed into rings across the system. Their materials travel from the outer disk to the inner disk—but how? "The inner portion of the disk is a very dynamic place," explains Tom Ray of the Dublin Institute for Advanced Studies in Ireland. "It's not only where terrestrial-type planets form, but it's also where supersonic jets are launched by the star."
- Jets emitted by the star lead to a mixing of elements in the inner and outer disks, both by sending out particles and permitting other particles to move inward. "We think that as material leaves, it loses its spin, or angular momentum, and that this allows other material to move inward," Ray continued. "These exchanges of material will obviously impact the chemistry of the inner disk, which we’re excited to explore with Webb."
Exciting Insights Await
- One of the research team's targets is TW Hydrae, which lies 196 light-years from Earth. This protoplanetary disk is close enough for Webb to image—which it will do while blocking the star to more clearly identify forming planets. It will also return a slew of spectra to detail the molecules in its inner disk. Existing data show a prominent inner area cleared of debris, which is where planets may be forming. Henning's team hopes to identify and characterize them with Webb.
- PDS 70 is farther at 370 light-years away. It also has a large gap in its inner ring, plus data have revealed that two forming planets, known as protoplanets, are present and gathering material. "Webb's mid-infrared measurements will help us refine what we know about them, as well as the material around them," Kamp explained.
- With dozens of targets on their list, it's difficult for team members to play favorites. "I love them all," Henning said. "One question I'd like to answer concerns the connection between the composition of planet-forming disks and the planets themselves. With Webb, we will observe far more detail about which types of material are available for a potential planet to accrete."
- After refining the data, his team will apply the discrete data points to models. "This will allow us to do a graphic reconstruction of these systems," he continued. These models will be shared with the astronomical community, enabling other scientists to examine the data, and make their own projections or glean new findings. These studies will be conducted through a Guaranteed Time Observations (GTO) program.
- The James Webb Space Telescope will be the world's premier space science observatory when it launches in 2021. Webb will solve mysteries in our solar system, look beyond to distant worlds around other stars, and probe the mysterious structures and origins of our universe and our place in it. Webb is an international program led by NASA with its partners, ESA (European Space Agency) and the Canadian Space Agency.
• November 18, 2020: Dust may seem insignificant, but it plays a huge role in the universe, from the formation of stars and planets to facilitating the complex chemistry that becomes the stuff of life—including us. Big questions like “How did we get here?” come down to dust, and yet the origin and formation process of cosmic dust has eluded scientists. Astronomers refer to the unexplained abundance of dust in galaxies as the “dust budget crisis.” It is a mystery that astronomers are excited to get to work solving using the specialized technology of NASA’s James Webb Space Telescope. 14)
- Discovering too much money in your bank account may not be what you would call a "crisis," but it would still be unexpected and you should figure out how it got there. Astronomers find themselves in a similar position when calculating the amount of dust galaxies should have; there is more dust than expected, and they don’t know where it’s coming from. This matters because cosmic dust is essential to the function of the universe: it shelters forming stars, becomes part of planets, and can contain the organic compounds that lead to life as we know it. Dust led to us.
- "What we refer to as the 'dust budget crisis' is the major problem in astronomy of not being able to account for all the dust that's observed in galaxies, both in the nearby and distant, early universe," says Ryan Lau of the Japan Aerospace Exploration Agency. Lau is leading an Director's Discretionary-Early Release Science Program with NASA's upcoming James Webb Space Telescope to study dust-producing Wolf-Rayet binary stars.
- Wolf-Rayet stars are very hot and very bright. There is evidence that Wolf-Rayet stars, through interactions with a companion star, produce large amounts of dust in a distinctive pinwheel pattern as the two stars orbit each other and their stellar winds collide. It is possible that these binary-star systems account for a large percentage of a galaxy's "dust budget." However, the intense luminosity and heat coming from the Wolf-Rayet stars has made it difficult to study the faint, more diffuse dust of these systems. This is where Webb comes in.
- "Webb has an unprecedented combination of spatial resolution and sensitivity in mid-infrared wavelengths that is really what enables us to conduct these interesting observations," Lau says. "We can achieve the spatial resolution from ground-based telescopes, but lack the sensitivity that Webb can achieve from its observing location in space, without the interference of Earth's atmosphere. Conversely, with previous infrared space-based telescopes like NASA’s Spitzer mission, we could achieve the sensitivity but lacked the spatial resolution."

Targeting Two Dust Factories
- Lau and the Director's Discretionary-Early Release Science (DD-ERS) team will use Webb to study two Wolf-Rayet binary systems, using the telescope's Mid-Infrared Instrument (MIRI) and Near Infrared Imager and Slitless Spectrograph (NIRISS). The WR140 binary system has been studied extensively in many wavelengths of light and so will provide a good baseline for gauging Webb's best observing modes for this kind of cosmic subject. Another Wolf-Rayet binary, WR137, will experience its stars’ closest approach to each other—when the most dust is thought to be produced—early in Webb's mission when the DD-ERS program observations are scheduled.
- Beyond new discoveries about the formation and chemical composition of dust, the DD-ERS program also will be among the first opportunities astronomers have to test out best practices for Webb’s instruments and processing the data it delivers.
- "This DD-ERS program will look at the best ways to maximize Webb’s dynamic range—the difference between the brightest and faintest objects it observes—and that will be useful to the astronomy community in many ways in the future; for example, in studying the dusty disk surrounding the bright center of an active galaxy, or finding a planet orbiting a bright star,” says Mansi Kasliwal, another astronomer on the DD-ERS team. Kasliwal led the laboratory at the California Institute of Technology where Lau performed his post-doctoral research on Wolf-Rayet binaries and developed the proposal for the DD-ERS program.
- Both Lau and Kasliwal agree that while the open question of how cosmic dust is created and disseminated throughout the universe is a fascinating one, it is really a stepping stone toward answering one of the biggest questions ever posed: How did we get here? As far as we know, Earth is an island of life in the universe, and in seeking to understand something as seemingly remote as cosmic dust, Lau says that we are ultimately seeking to understand ourselves. "Understanding the formation of dust is critical for us to trace our own cosmic origins," Lau says. "Webb is one of the most powerful scientific tools ever built in the quest to find answers to these fundamental questions."
- The James Webb Space Telescope will be the world's premier space science observatory when it launches in 2021. Webb will solve mysteries in our solar system, look beyond to distant worlds around other stars, and probe the mysterious structures and origins of our universe and our place in it. Webb is an international program led by NASA with its partners, ESA (European Space Agency) and the Canadian Space Agency.
• October 28, 2020: Beyond the orbit of Neptune, a diverse collection of thousands of dwarf planets and other relatively small objects dwells in a region called the Kuiper Belt. These often-pristine leftovers from our solar system's days of planet formation are called Kuiper Belt Objects, or Trans-Newtonian Objects. NASA's upcoming James Webb Space Telescope will examine an assortment of these icy bodies in a series of programs called Guaranteed Time Observations shortly after its launch in 2021. The goal is to learn more about how our solar system formed. 15)
- "These are objects that are in the graveyard of solar system formation," explained Cornell University's Jonathan Lunine, a Webb Interdisciplinary Scientist who will use Webb to study some of these targets. "They're in a place where they could last for billions of years, and there aren't many places like that in our solar system. We'd love to know what they're like."
- By studying these bodies, Lunine and his colleagues hope to learn about which ices were present in the early solar system. These are the coldest worlds to display geologic and atmospheric activity, so scientists are also interested in comparing them with the planets.
- Kuiper Belt Objects are very cold and faint, yet they glow in infrared light, which is at wavelengths beyond what our human eyes can see. Webb is specifically designed to detect infrared light. To study these distant objects, scientists mainly will use a technique called spectroscopy, which divides light into its individual colors to determine the properties of materials that interact with that light.
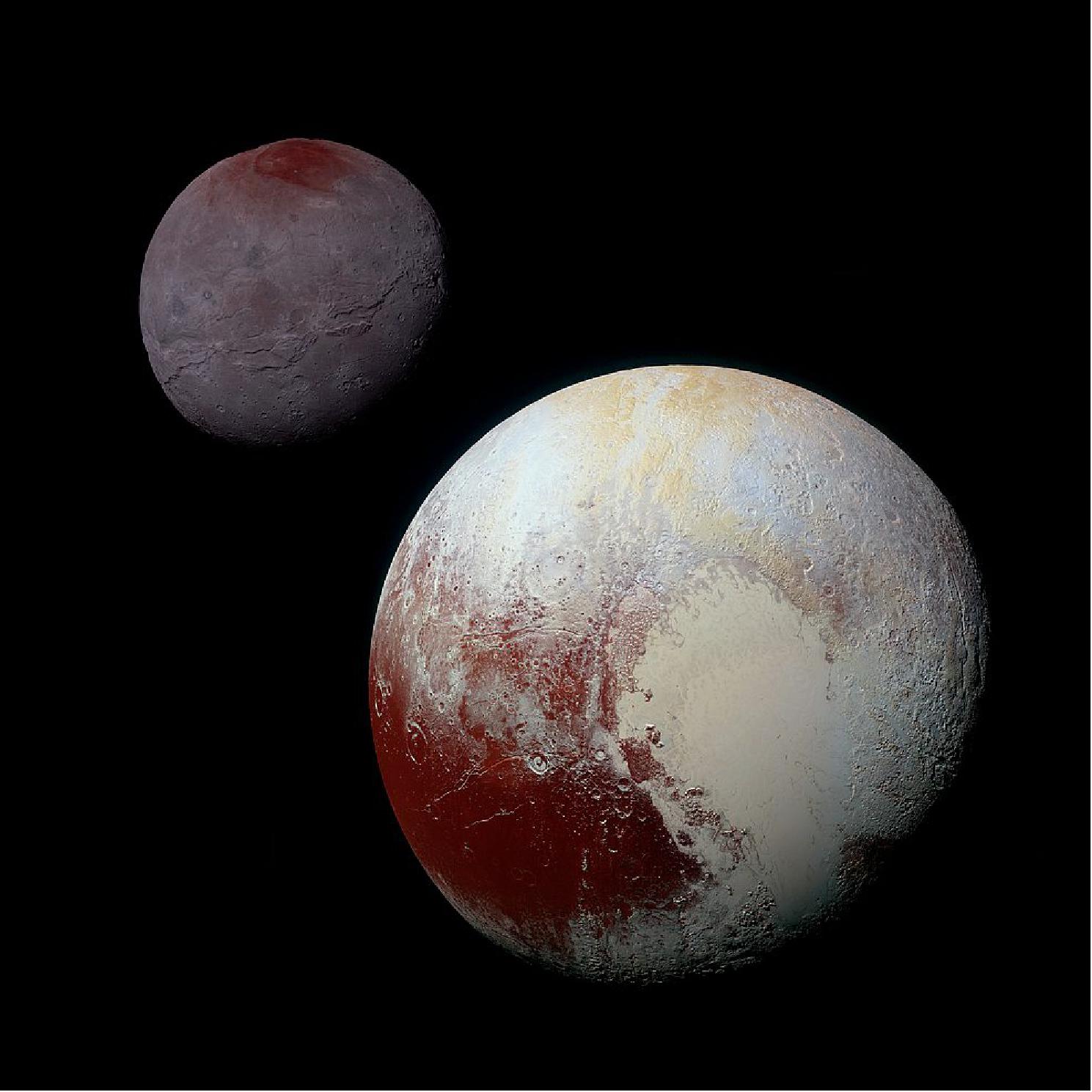
- "These are objects that are in the graveyard of solar system formation," explained Cornell University's Jonathan Lunine, a Webb Interdisciplinary Scientist who will use Webb to study some of these targets. "They're in a place where they could last for billions of years, and there aren't many places like that in our solar system. We'd love to know what they're like."
- By studying these bodies, Lunine and his colleagues hope to learn about which ices were present in the early solar system. These are the coldest worlds to display geologic and atmospheric activity, so scientists are also interested in comparing them with the planets.
- Kuiper Belt Objects are very cold and faint, yet they glow in infrared light, which is at wavelengths beyond what our human eyes can see. Webb is specifically designed to detect infrared light. To study these distant objects, scientists mainly will use a technique called spectroscopy, which divides light into its individual colors to determine the properties of materials that interact with that light.
A Wide Assortment
- The denizens of the Kuiper Belt come in various shapes and sizes. Some reside in pairs or multiples, while others have rings or moons. They exhibit a wide range of colors, which may indicate different formation histories or different exposure to sunlight.
- "Some seem to be redder in color, others are bluer. Why is that?" said Heidi Hammel, a Webb Interdisciplinary Scientist for solar system observations. She is also Vice President for Science at the Association of Universities for Research in Astronomy (AURA) in Washington, D.C. "Using Webb, we will be able to get information about surface chemistry that might be able to give us some clues into why there are these different populations in the Kuiper Belt."
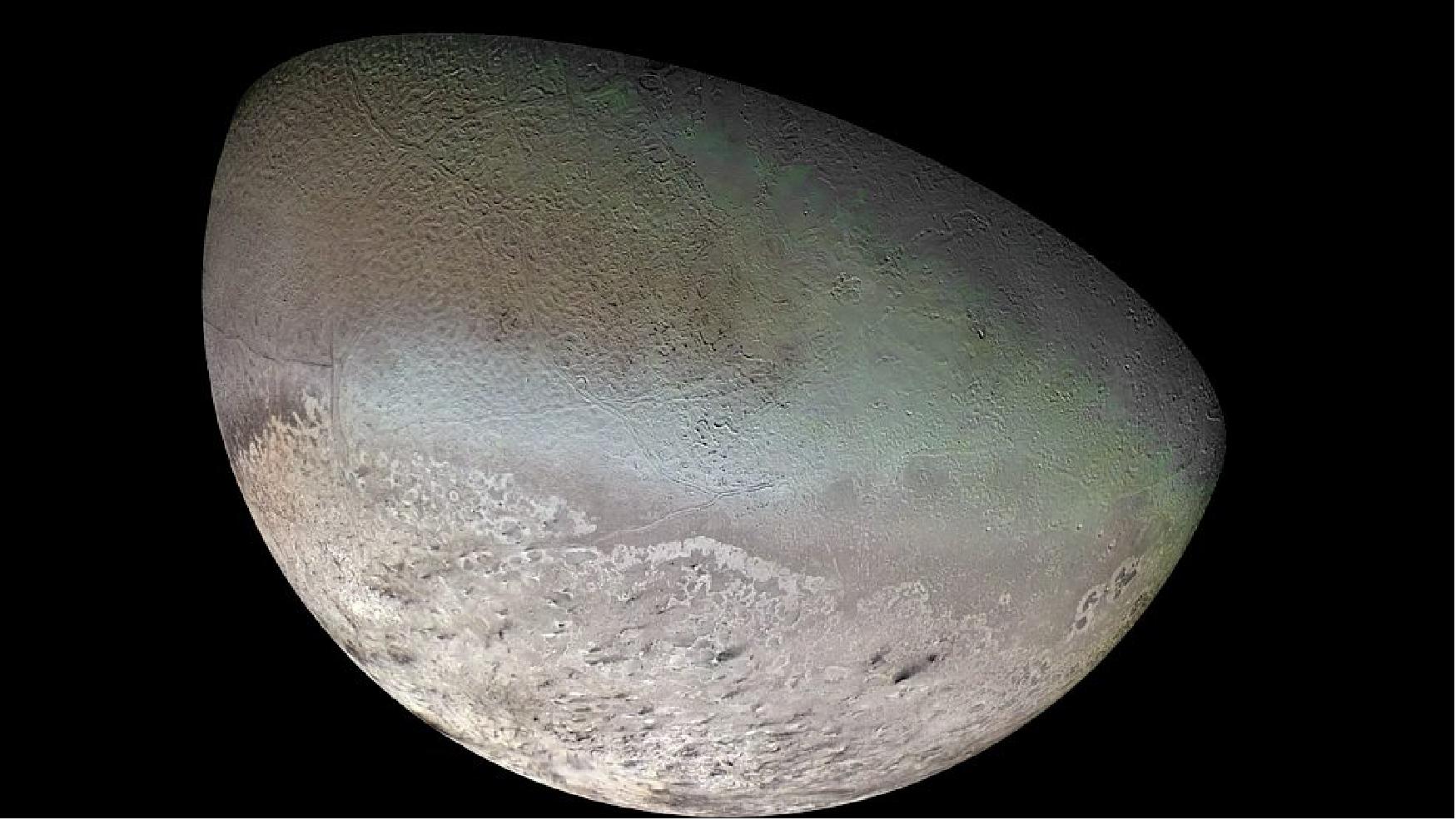
Kicked out of the Club
- Between Jupiter and Neptune, and crossing the orbit of one or more of the giant planets, lies a different population of objects called centaurs. These are small solar system bodies that have been ejected from the Kuiper Belt. In addition to observing current Kuiper Belt Objects, these Webb programs will study such solar system bodies that have been "kicked out of the club." These former Kuiper Belt Objects have orbits that have been dramatically disturbed, bringing them significantly closer to the Sun.
- "Because they cross the orbits of Neptune, Uranus, and Saturn, centaurs are short-lived. So they are typically only around for about 10 million years," explained John Stansberry of the Space Telescope Science Institute in Baltimore, Maryland. Stansberry is leading a different team that will use Webb to study Kuiper Belt Objects. "By that point, they have an interaction with one of the major planets that's very strong, and they either get thrown into the Sun or thrown out of the solar system."
- Another body that Webb will study is Neptune's moon Triton. The largest of the ice giant's 13 moons, Triton shares many similarities with Pluto. "Even though it's Neptune's moon, we have evidence to suggest that it is a Kuiper Belt Object that got too close to Neptune sometime in its past, and it was captured into orbit around Neptune," said Hammel. "Triton was studied by the Voyager 2 probe in 1989. That spacecraft data will provide us very important 'ground truth' for our Webb observations of Kuiper Belt Objects."
A Sampling of the Targets
- Here is a small sampling of some of the dozens of current and former Kuiper Belt Objects that Webb will observe:
a) Pluto and Charon: The dwarf planet Pluto and its largest moon, Charon, are two of the most well-known residents of the Kuiper Belt. Pluto boasts an atmosphere, haze, and seasons. It has geologic activity on its surface and may have an ocean in its interior. In addition to Charon, it hosts four other moons: Nix, Hydra, Styx, and Kerberos. The Webb data will complement the observations made by NASA's New Horizons spacecraft when it flew by the Pluto system in 2015.
b) Eris: Nearly the size of Pluto, Eris is the second-largest known dwarf planet in the solar system. At its farthest point, mysterious Eris is more than 97 times as far from the Sun as the Earth is. Because of its distance, it is difficult to observe, but Webb will tell scientists quite a bit about what kinds of ices are on its surface.
c)- Sedna: With its deep red hue, Sedna is actually located beyond the main Kuiper Belt. It takes approximately 11,400 years to complete one orbit, and the farthest point of that highly elongated orbit is estimated to be 940 times Earth's distance from the Sun.
d) Haumea: This large, rapidly spinning body is egg-shaped, and scientists would like to know why. In addition to moons, it also seems to have a ring system. With Webb, scientists hope to learn more about how those rings formed.
e) Chariklo: The largest centaur, Chariklo is also the first asteroid found to have a ring system. It was the fifth ring system found in our solar system—after Saturn, Jupiter, Uranus and Neptune. The rings are believed to be between two and four miles wide.
- Another program, called a Target of Opportunity, will observe a Kuiper Belt Object passing in front of a star, if such an alignment should occur during the first two years of Webb's lifetime. Called an occultation, this type of observation can reveal an object's size.
- The few spacecraft that have flown by Kuiper Belt Objects could only study these intriguing objects for a very short period of time. With Webb, astronomers can target more Kuiper Belt Objects over an extended time. The result will be new insights into our solar system's earliest history.
- The James Webb Space Telescope will be the world's premier space science observatory when it launches in 2021. Webb will solve mysteries in our solar system, look beyond to distant worlds around other stars, and probe the mysterious structures and origins of our universe and our place in it. Webb is an international program led by NASA with its partners, ESA (European Space Agency) and the Canadian Space Agency.
• July 31 2020: NASA’s James Webb Space Telescope will have a challenging early assignment in the solar system: observe the largest, fastest-rotating planet—Jupiter—as well as its faint rings and two of the four Galilean satellites: icy Ganymede and fiery Io. In addition to laying groundwork for the rest of Webb’s mission, the ambitious program should yield new scientific insights, not only into the Jovian system, but also the geological history of Earth and exoplanet science. 16)
Jupiter, named for the king of the ancient Roman gods, commands its own mini-version of our solar system of circling satellites; their movements convinced Galileo Galilei that Earth is not the center of the universe in the early 17th century. More than 400 years later, astronomers will use NASA’s James Webb Space Telescope to observe these famous subjects, pushing the observatory’s instruments to their fullest capabilities and laying the groundwork for far-reaching scientific discovery.
A diverse team of more than 40 researchers, led by astronomers Imke de Pater of the University of California, Berkeley and Thierry Fouchet of the Observatoire de Paris, have designed an ambitious observing program that will conduct some of Webb’s first scientific observations in the solar system—studying Jupiter, its ring system, and two of its moons: Ganymede and Io.
“It will be a really challenging experiment,” said de Pater. “Jupiter is so bright, and Webb’s instruments are so sensitive, that observing both the bright planet and its fainter rings and moons will be an excellent test of how to get the most out of Webb’s innovative technology.”

Jupiter: In addition to calibrating Webb’s instruments for Jupiter’s brightness, astronomers must also take into account the planet’s rotation, because Jupiter completes one day in only 10 hours. Several images must be stitched together in a mosaic to fully capture a certain area—the famous storm known as the Great Red Spot, for example—a task made more difficult when the object itself is moving. While many telescopes have studied Jupiter and its storms, Webb’s large mirror and powerful instruments will provide new insights.
We know that the immediate atmosphere above the Great Red Spot is colder than other areas of Jupiter, but at higher altitudes, in the mesosphere, the atmosphere appears to be warmer. We will use Webb to investigate this phenomenon,” de Pater said.
Webb will also examine the atmosphere of the polar region, where NASA’s Juno spacecraft discovered clusters of cyclones. Webb’s spectroscopic data will provide much more detail than has been possible in past observations, measuring winds, cloud particles, gas composition, and temperature.
Future solar system observations of the giant planets with Webb will benefit from the lessons learned in these early observations of the Jovian system. The team is tasked with developing methods for working with Webb observations of solar system planets, which can be used later by other scientists.
Rings: All four of the gas giant planets of the solar system have rings, with Saturn’s being the most prominent. Jupiter’s ring system is composed of three parts: a flat main ring; a halo inside the main ring, shaped like a double-convex lens; and the gossamer ring, exterior to the main ring. Jupiter’s ring system is exceptionally faint because the particles that make up the rings are so small and sparse that they do not reflect much light. Next to the brightness of the planet they practically disappear, presenting a challenge for astronomers.
“We are really pushing the capabilities of some of Webb’s instruments to the limit to get a unique new set of observations,” said co-investigator Michael Wong of the University of California, Berkeley. The team will test observing strategies to deal with Jupiter’s scattered light, and build models for use by other astronomers, including those studying exoplanets orbiting bright stars.
The team will look to make new discoveries in the rings as well. De Pater noted that there may be undiscovered “ephemeral moonlets” in the dynamic ring system, and potential ripples in the ring from comet impacts, like those observed and traced back to the impact of Comet Shoemaker-Levy 9 in 1994.
Ganymede: Several features of icy Ganymede make it fascinating for astronomers. Aside from being the largest moon in the solar system, and larger even than the planet Mercury, it is the only moon known to have its own magnetic field. The team will investigate the very outer parts of Ganymede’s atmosphere, its exosphere, to better understand the moon’s interaction with particles in Jupiter’s magnetic field.
There is also evidence that Ganymede may have a liquid saltwater ocean beneath its thick surface ice, which Webb will investigate with detailed spectroscopic study of surface salts and other compounds. The team’s experience studying Ganymede’s surface may be useful in the future study of other icy solar system moons suspected of having subsurface oceans, including Saturn’s moon Enceladus and fellow Jovian satellite Europa.
Io: In dramatic contrast to Ganymede is the other moon the team will study, Io, the most volcanically active world in the solar system. The dynamic surface is covered with hundreds of huge volcanoes that would dwarf those on Earth, as well as lakes of molten lava and smooth floodplains of solidified lava. Astronomers plan to use Webb to learn more about the effects of Io’s volcanoes on its atmosphere.
“There is still much we don’t know about Io’s atmospheric temperature structure, because we haven’t had the data to distinguish the temperature at different altitudes,” said de Pater. “On Earth we take for granted that as you hike up a mountain, the air gets cooler—would it be the same on Io? Right now we don’t know, but Webb may help us to find out.”
Another mystery Webb will investigate on Io is the existence of “stealth volcanoes,” which emit plumes of gas without the light-reflecting dust that can be detected by spacecraft like NASA’s Voyager and Galileo missions, and so have thus far gone undetected. Webb’s high spatial resolution will be able to isolate individual volcanoes that previously would have appeared as one large hotspot, allowing astronomers to gather detailed data on Io’s geology.
Webb will also provide unprecedented data on the temperature of Io’s hotspots, and determine if they are closer to volcanism on Earth today, or if they have a much higher temperature, similar to the environment on Earth in the early years after its formation. Previous observations by the Galileo mission and ground observatories have hinted at these high temperatures; Webb will follow up on that research and provide new evidence that may settle the question.
Team Effort: Webb’s detailed observations will not supplant those of other observatories, but rather coordinate with them, Wong explained. “Webb’s spectroscopic observations will cover just a small area of the planet, so global views from ground-based observatories can show how the detailed Webb data fit in with what’s happening on a larger scale, similar to how Hubble and the Gemini Observatory provide context for Juno’s narrow, close-up observations.”
In turn, Webb’s study of Jupiter’s storms and atmosphere will complement Juno data, including radio signals from lightning, which Webb does not detect. “No one observatory or spacecraft can do it all,” Wong said, “so we are very excited about combining data from multiple observatories to tell us much more than we could learn from only a single source.”
This research is being conducted as part of a Webb Early Release Science (ERS) program. This program provides time to selected projects early in the observatory’s mission, allowing researchers to quickly learn how best to use Webb’s capabilities, while also yielding robust science.
The James Webb Space Telescope will be the world’s premier space science observatory when it launches in 2021. Webb will solve mysteries in our solar system, look beyond to distant worlds around other stars, and probe the mysterious structures and origins of our universe and our place in it. Webb is an international program led by NASA with its partners, ESA (European Space Agency) and the Canadian Space Agency.
Mapping the Early Universe with NASA's Webb Telescope
• June 24, 2020: Astronomers and engineers have designed telescopes, in part, to be "time travelers." The farther away an object is, the longer its light takes to reach Earth. Peering back in time is one reason why NASA's upcoming James Webb Space Telescope specializes in collecting infrared light: These longer wavelengths, which were initially emitted by stars and galaxies as ultraviolet light more than 13 billion years ago, have stretched, or redshifted, into infrared light as they traveled toward us through the expanding universe. 17) 18)
Although many other observatories, including NASA's Hubble Space Telescope, have previously created "deep fields" by staring at small areas of the sky for significant chunks of time, the Cosmic Evolution Early Release Science (CEERS) Survey, led by Steven L. Finkelstein of the University of Texas at Austin, will be one of the first for Webb. He and his research team will spend just over 60 hours pointing the telescope at a slice of the sky known as the Extended Groth Strip, which was observed as part of Hubble's Cosmic Assembly Near-infrared Deep Extragalactic Legacy Survey or CANDELS.
"With Webb, we want to do the first reconnaissance for galaxies even closer to the big bang," Finkelstein said. "It is absolutely not possible to do this research with any other telescope. Webb is able to do remarkable things at wavelengths that have been difficult to observe in the past, on the ground or in space."
Mark Dickinson of the National Science Foundation's National Optical-Infrared Astronomy Research Laboratory in Arizona, and one of the CEERS Survey co-investigators, gives a nod to Hubble while also looking forward to Webb's observations. "Surveys like the Hubble Deep Field have allowed us to map the history of cosmic star formation in galaxies within a half a billion years of the big bang all the way to the present in surprising detail," he said. "With CEERS, Webb will look even farther to add new data to those surveys."
![Figure 26: The CEERS Survey researchers will use the James Webb Space Telescope to observe the Extended Groth Strip in infrared light. Their observations employ three of the telescope’s instruments and will provide both images and spectra of the objects in the field — which includes at least 50,000 galaxies — helping to expand what we know about galaxies in the very early universe [image credits: NASA, ESA, and M. Davis (University of California, Berkeley)]](https://eoportal.org/ftp/satellite-missions/j/JWST-Feature_170222/JWST-Feature_Auto3.jpeg)
Delivering the Unseen
What was the early universe like? There are certainly many data points, but not enough to create an exhaustive census of its conditions. Plus, researchers' knowledge and assumptions are updated frequently — each time a new deep exposure is released. "Every time we look farther, we find galaxies earlier and earlier than we thought possible," said CEERS Survey co-investigator Jeyhan Kartaltepe of the Rochester Institute of Technology in New York. "The conditions in the very early universe had to be right for galaxies to form — and they formed and became massive very quickly."
"The universe was more compact at this time, which means stars and galaxies could have formed at a greater efficiency," Finkelstein added. "Some models predict we'll find 50 galaxies at earlier eras more distant than Hubble can reach, but others predict we will only find a few. In both cases, the data will help us constrain galaxy formation in the early universe."
The CEERS Survey team hopes to identify an abundance of distant objects, including the most distant galaxies in the universe, early galaxy mergers and interactions, the first massive or supermassive black holes, and even earlier quasars than previously identified. These potential "firsts" are only the beginning of the value of this research: The team, which is made up of over 100 researchers from around the world, will go on to classify many objects in the field. "These data will help demonstrate what the structure of the universe was like at various periods," Finkelstein explained.
Hitting "Rewind"
Perhaps the most exciting element of this research is how the team will use the data to uncover new findings about an important period of the universe's history called the "Era of Reionization." The big bang set off a series of events, leading to the cosmic microwave background, the dark ages, the first stars and galaxies — and then to the Era of Reionization. During this period, the gas in the universe transformed from mostly neutral, meaning it was opaque to ultraviolet light, and became completely ionized, which allowed it to be transparent. Ionization means the atoms were stripped of their electrons — eventually leading to the "clear" conditions detected in much of the universe today.
Many questions remain about this unique time in our universe. For example, what was responsible for converting the gas from neutral to ionized? And how long did it take before the universe became significantly less opaque and much more transparent?
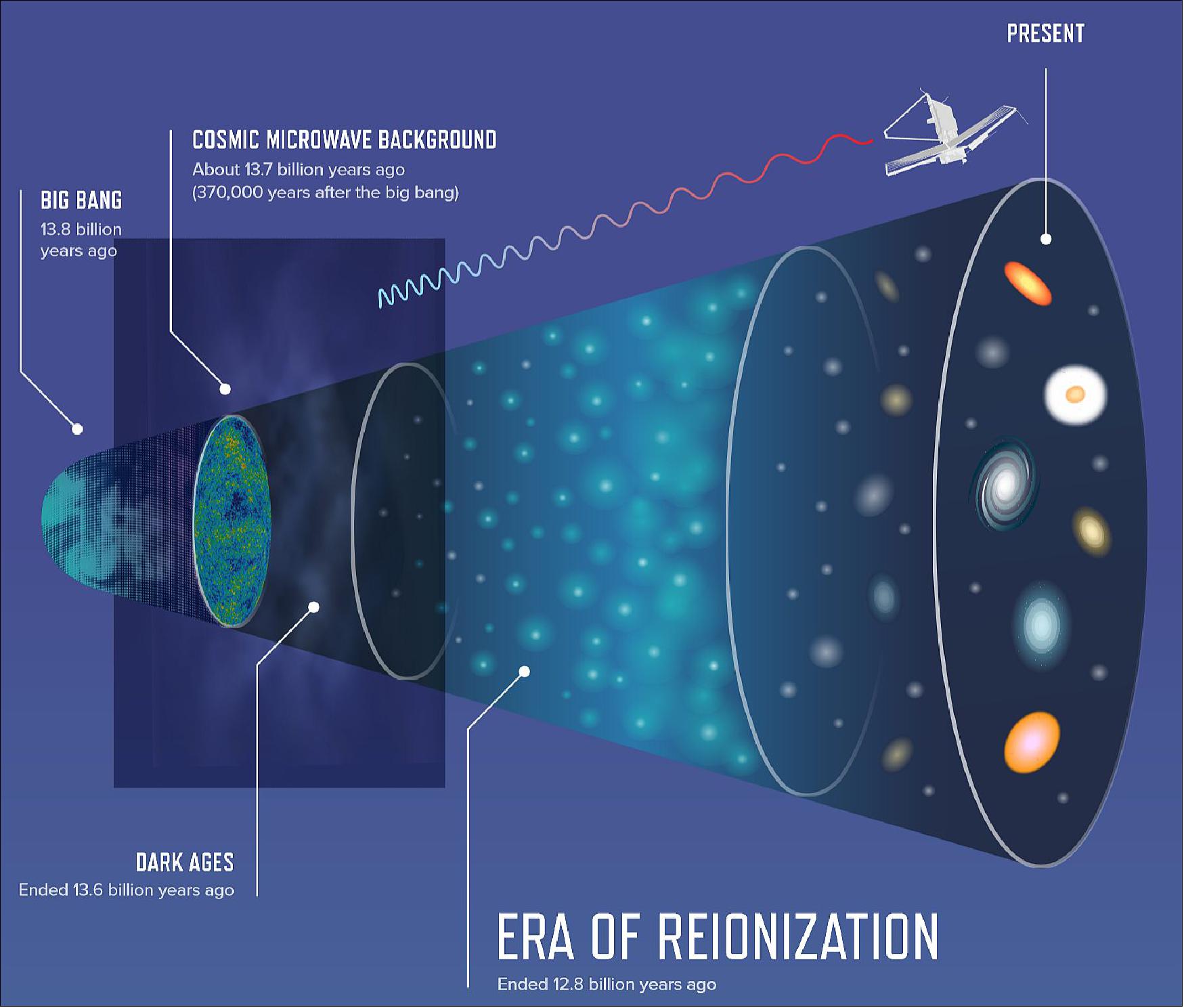
"We think this happened when ultraviolet light escaped young, forming galaxies," Dickinson explained. "There may be other factors. For example, early accreting black holes may also have emitted ultraviolet light that eventually helped transform the gas."
Where the galaxies appear on the sky offers another clue. "We'll examine reionization-era galaxies to see if they are clustered together in the same regions or if they are more isolated," said Kartaltepe. "We have a lot of ideas about what causes galaxies to grow and become more massive, but we need more comprehensive information about these galaxies to fully understand how they initially grew and evolved."
The presence of galactic mergers or interactions — or lack thereof — will also help the team trace the conditions of the environment during the Era of Reionization. "The CEERS Survey will give us hints about how this period proceeded," Dickinson adds. "We will certainly learn about the galaxies we think are responsible, and also hope to learn about the ionizing radiation that escaped them."
The team has designed the CEERS Survey to provide as much complementary data as possible for many targets in this field of view. They will employ three of Webb's instruments, in several modes, to obtain images of the Extended Groth Strip, in addition to spectra. Spectra are invaluable data since they help researchers identify the colors, temperatures, motions, and masses of each target, and provide a much more in-depth look at the chemical makeup of distant objects.
"That's the difference with Webb's NIRSpec (Near-Infrared Spectrograph)," Dickinson emphasized. "We'll open the spectrograph's microshutter slits to individually observe hundreds of galaxies to obtain their spectra for the first time."
Beginning to Build a Census
In the months following the initial data release, the CEERS Survey researchers will create and post new tools and catalogs any researcher can use to analyze the data, including masses of galaxies, galaxy shapes, and photometric redshifts. "With the same set of observations, hundreds of researchers can conduct hundreds of science experiments," Kartaltepe said. "We're also going to find things we didn't even think to ask, which is one more reason why the CEERS Survey research will be so rewarding."
"Our hope is that the CEERS Survey will influence future distant galaxy surveys with Webb," Finkelstein added. "It will also demonstrate to the community that observing with a variety of instruments and modes are very valid ways to increase Webb's scientific yield."
This research is being conducted as part of a Webb Early Release Science (ERS) program. This program provides time to selected projects early in the telescope's mission, allowing researchers to quickly learn how best to use Webb's capabilities, while also yielding robust science.
The James Webb Space Telescope will be the world's premier space science observatory when it launches in 2021. Webb will solve mysteries in our solar system, look beyond to distant worlds around other stars, and probe the mysterious structures and origins of our universe and our place in it. Webb is an international program led by NASA with its partners, ESA (European Space Agency) and the Canadian Space Agency.
NASA's Webb Telescope Will Study an Iconic Supernova
• February 28, 2019: Within a galaxy known as the Large Magellanic Cloud, a star exploded 160,000 years ago. In 1987, light from that exploding star reached Earth. Over the past 32 years, astronomers have studied Supernova 1987A to learn about the physics of supernovas and their gaseous remnants. Those observations have revealed a surprising amount of dust, up to an entire sun’s worth. NASA’s infrared James Webb Space Telescope will study the dust within SN 1987A to learn about its composition, temperature and density. 19)
In February 1987, light from an exploding star arrived at Earth after traveling across 160,000 light-years of space. It was the closest supernova humanity had seen in centuries. Thirty-two years later, the light of the supernova itself has faded, but astronomers continue to study its remains for clues about how stars live and die. Scientists will use NASA's James Webb Space Telescope to observe Supernova 1987A (SN 1987A), as it is known, in order to gain new insights into the physics of the explosion and its aftermath.
When you look at a photo of SN 1987A, two features stand out: a clumpy outer ring that looks like a pearl necklace, and an inner blob. The outer ring is material that the star shed thousands of years ago. When the supernova's blast wave hit this ring, it caused the previously invisible material to heat up and glow. The inner blob is material ejected when the star exploded.
That ejected material revealed a surprise when astronomers observed it with the European Space Agency’s infrared Herschel Space Observatory. They found that it contained an entire sun's worth of cold dust. More recently, NASA’s SOFIA (Stratospheric Observatory for Infrared Astronomy) mission studied the ring and detected 10 times more dust than expected, indicating a growing amount of dust there, too.
Theories suggest that any dust within the ring that predated the explosion should have been destroyed by the blast wave, and the ejecta itself should be too hot for new dust to form. As a result, there should be little dust within SN 1987A. Yet observations tell a different story.
"Something has produced dust there. We need Webb to answer questions like, how was the dust produced, and what is it made of?" said lead researcher Margaret Meixner of the Space Telescope Science Institute and Johns Hopkins University, both in Baltimore, Maryland.
![Figure 28: Multiwavelength view of Supernova 1987A. Astronomers combined observations from three different observatories ALMA (Atacama Large Millimeter/submillimeter Array), red; Hubble, green; Chandra X-ray Observatory, blue) to produce this colorful, multiwavelength image of the intricate remains of Supernova 1987A [image credit: NASA, ESA, A. Angelich (NRAO/AUI/NSF); Hubble credit: NASA, ESA, R. Kirshner; Chandra credit: NASA/CXC/Penn State/K. Frank et al.; ALMA credit: ALMA (ESO/NAOJ/NRAO) and R. Indebetouw (NRAO/AUI/NSF)]](https://eoportal.org/ftp/satellite-missions/j/JWST-Feature_170222/JWST-Feature_Auto1.jpeg)
What is Dust, and Why is it Important?
Cosmic dust is different from the dust bunnies that you find under your furniture. It's smaller, mainly consisting of µm-sized particles like those in smoke. And rather than being made of bits of hair or clothing fibers, cosmic dust is composed of a variety of chemical elements like carbon, silicon and iron all stuck together. As a result, measuring the composition of a particular patch of cosmic dust is challenging because the signatures of the elements blend together.
"We have no clue what the dust in Supernova 1987A is made of – whether it's rocky and silicate-rich, or sooty and carbon-rich. Webb will let us learn not only the composition of the dust, but its temperature and density," explained Olivia Jones of the United Kingdom Astronomy Technology Center, a co-investigator on the project.
As dust from dying stars spreads through space, it carries essential elements to help seed the next generation of star and planet formation. "Dust is what the planets are made out of, what we're made out of. Without dust, you have no planets," said Jones.
Dust also is important for the evolution of galaxies. Observations have shown that distant, young galaxies had lots of dust. Those galaxies weren't old enough for sun-like stars to create so much dust, since sun-like stars last for billions of years. Only more massive, short-lived stars could have died soon enough and in large enough numbers to create the vast quantities of dust astronomers see in the early universe.
The Birth of a Supernova Remnant
The team plans to examine SN 1987A with two of Webb's instruments: the Mid-Infrared Imager (MIRI) and the Near-Infrared Spectrograph (NIRSpec). With imaging, Webb will reveal features of SN 1987A far beyond any previous infrared observations due to its exquisite resolution. Astronomers expect to be able to map the temperature of the dust within both the supernova ejecta and the surrounding ring. They can also study the interaction of the blast wave with the ring in great detail.
Webb's true power will come from its spectroscopic measurements. By spreading light out into a rainbow spectrum of colors, scientists can determine not only chemical compositions but also temperatures, densities, and speeds. They can examine the physics of the blast wave, and determine how it is affecting the surrounding environment. They can also watch the evolution of the ejected material and ring over time.
"We're witnessing the birth of a supernova remnant," said Patrice Bouchet of DRF/Irfu/Astrophysics Department, CEA-Saclay in France, a co-principal investigator for the MIRI European Consortium. "This is a once-in-a-lifetime event."
"Supernova 1987A is an object that continually surprises people," said Meixner. "This is one you'll want to keep your eyes open for!"
The observations described here will be taken as part of Webb's Guaranteed Time Observation (GTO) program. The GTO program provides dedicated time to the scientists who have worked with NASA to craft the science and instrument capabilities of Webb throughout its development.
The James Webb Space Telescope will be the world's premier space science observatory when it launches in 2021. Webb will solve mysteries of our solar system, look beyond to distant worlds around other stars, and probe the mysterious structures and origins of our universe and our place in it. Webb is an international project led by NASA with its partners, the European Space Agency (ESA) and the Canadian Space Agency.
How to Weigh a Black Hole Using JWST
October 17, 2018: At first glance, the galaxy NGC 4151 looks like an average spiral. Examine its center more closely, though, and you can spot a bright smudge that stands out from the softer glow around it. That point of light marks the location of a supermassive black hole weighing about 40 million times as much as our Sun. 20)
Astronomers will use NASA’s James Webb Space Telescope to measure that black hole’s mass. The result might seem like a piece of trivia, but its mass determines how a black hole feeds and affects the surrounding galaxy. And since most galaxies contain a supermassive black hole, learning about this nearby galaxy will improve our understanding of many galaxies across the cosmos.
“Some central questions in astrophysics are: How does a galaxy’s central black hole grow with time; how does the galaxy itself grow with time; and how do they affect each other? This project is a step toward answering those questions,” explained Misty Bentz of Georgia State University, Atlanta, the principal investigator of the project.
![Figure 29: The spiral galaxy NGC 4151 has a bright, active core powered by a supermassive black hole. Webb will weigh the black hole by measuring the motions of stars at the galaxy’s center [image credits: NASA, ESA, and J. DePasquale (STScI)]](https://eoportal.org/ftp/satellite-missions/j/JWST-Feature_170222/JWST-Feature_Auto0.jpeg)
Probing a galaxy’s core: There are several methods of weighing supermassive black holes. One technique relies on measuring the motions of stars in the galaxy’s core. The heavier the black hole, the faster nearby stars will move under its gravitational influence.
NGC 4151 represents a challenging target, because it contains a particularly active black hole that is feeding voraciously. As a result, the material swirling around the black hole, known as an accretion disk, shines brightly. The light from the accretion disk threatens to overwhelm the fainter light from stars in the region.
“With Webb’s beautifully shaped mirrors and sharp ‘vision,’ we should be able to probe closer to the galaxy’s center even though there’s a really bright accretion disk there,” said Bentz.
The team expects to be able to investigate the central 1,000 light-years of NGC 4151, and be able to resolve stellar motions on a scale of about 15 light-years.
A thousand spectra at once: To achieve this feat, the team will use Webb’s NIRSpec (Near-Infrared Spectrograph) integral field unit, or IFU. It will be the first IFU flown in space, and it has a unique capability.
Webb’s IFU takes the light from every location in an image and splits it into a rainbow spectrum. To do this it employs almost 100 mirrors, each of them precision crafted to a specific shape, all squeezed into an instrument the size of a shoebox. Those mirrors effectively slice a small square of the sky into strips, then spread the light from those strips out both spatially and in wavelength.
In this way a single image yields 1,000 spectra. Each spectrum tells astronomers not only about the elements that make up the stars and gas at that exact point of the sky, but also about their relative motions.
Despite Webb’s exquisite resolution, the team won’t be able to measure the motions of individual stars. Instead, they will get information about groups of stars very close to the center of the galaxy. They will then apply computer models to determine the gravitational field affecting the stars, which depends on the size of the black hole.
“Our computer code generates a bunch of mock stars – tens of thousands of stars, mimicking the motions of real stars in the galaxy. We put in a variety of different black holes and see what matches the observations the best,” said Monica Valluri of the University of Michigan, a co-investigator on the project.
The result of this technique will be compared with a second one that focuses on the gas at the galaxy’s center, rather than the stars.
“We should get the same answer, no matter what technique we use, if we’re looking at the same black hole,” said Bentz. “NGC 4151 is one of the best targets for making that comparison.”
These observations will be taken as part of the Director’s Discretionary-Early Release Science program. The DD-ERS program provides time to selected projects enabling the astronomical community to quickly learn how best to use Webb’s capabilities, while also yielding robust science.
The James Webb Space Telescope will be the world's premier space science observatory. Webb will solve mysteries of our solar system, look beyond to distant worlds around other stars, and probe the mysterious structures and origins of our universe and our place in it. Webb is an international project led by NASA with its partners, the European Space Agency (ESA) and the Canadian Space Agency (CSA).
References
1) Ann Jenkins, Christine Pulliam, ”Studying the Next Interstellar Interloper with Webb,” Webb Space Telescope News Release, 16 February 2022, URL: https://webbtelescope.org
/contents/news-releases/2022/news-2022-008.html
2) ”Capturing All That Glitters in Galaxies with NASA’s Webb,” Webb Space Telescope, 19 January, 2022, URL: https://webbtelescope.org/contents/news-releases/2022/news-2022-007.html
3) ”Scouting Ancient Supermassive Black Holes with NASA’s Webb,” Webb Space Telescope, 8 December 2021, URL: https://webbtelescope.org/contents/news-releases/2021/news-2021-056.html
4) ”Webb Primed to Lift the Haze Surrounding Sub-Neptunes,” Webb Space Telescope News Release 2021-054, 17 November 2021, URL: https://webbtelescope.org/contents/news-releases/2021/news-2021-054
5) ”NASA’s Webb Will Join Forces with the Event Horizon Telescope to Reveal the Milky Way’s Supermassive Black Hole,” Webb News Release ID: 2021-053, 27 October 2021, URL: https://webbtelescope.org/contents/news-releases/2021/news-2021-053.html#section-id-2
6) ”NASA’s Webb to Explore Forming Planetary Systems,” NASA's JWST News Release, 22 September 2021, URL: https://webbtelescope.org/contents/news-releases/2021/news-2021-052.html
7) ”Mapping the Universe's Earliest Structures with COSMOS-Webb,” NASA JWST News Release, 18 August 2021, URL: https://webbtelescope.org/contents/news-releases/2021/news-2021-042.html
8) Claire Blome, Christine Pulliam, ”NASA’s Webb to Explore a Neighboring, Dusty Planetary System,” NASA JWST, 21 July 2021, URL: https://webbtelescope.org
/contents/news-releases/2021/news-2021-032.html
9) ”NASA’s Webb Will Use Quasars to Unlock the Secrets of the Early Universe,” NASA , ESA, CSA, STScI News Release 2021-026, 23 June 2021, URL: https://webbtelescope.org
/contents/news-releases/2021/news-2021-026.html
10) ”NASA's Webb to Study Young Exoplanets on the Edge,” NASA News Release, 21 April 2021, URL: https://webbtelescope.org/contents/news-releases/2021/news-2021-023.html
11) ”Peering into a Galaxy's Dusty Core to Study an Active Supermassive Black Hole,” Webb News Release ID: 2021-15, 17 March 2021, URL: https://webbtelescope.org/contents/news-releases/2021/news-2021-15
12) ”NASA’s Webb Telescope Will Show Us More Stars at Higher Resolution—Here’s What That Means for Astronomy,” NASA's JWST Release ID: 2021-12, 22 February 2021, URL: https://webbtelescope.org/contents/news-releases/2021/news-2021-12
13) ”Detailing the Formation of Distant Solar Systems with NASA's Webb Telescope — Researchers will conduct a survey to compare planet-forming disks,” NASA News Release 10: 2020-60, 16 December 2020, URL: https://webbtelescope.org/contents/news-releases/2020/news-2020-60
14) ”NASA’s Webb Telescope Will Investigate the Intertwined Origins of Dust and Life,” NASA JWST, 18 November 2020, URL: https://webbtelescope.org/contents/news-releases/2020/news-2020-57
15) Ann Jenkins (STSI),”NASA’s Webb To Examine Objects in the Graveyard of the Solar System,” NASA Feature, 28 October 2020, URL: https://www.nasa.gov/feature/goddard/
2020/nasa-s-webb-to-examine-objects-in-the-graveyard-of-the-solar-system/
16) ”NASA's Webb Telescope Will Study Jupiter, its Rings, and Two Intriguing Moons,” NASA News Release 2020-38, 31 July 2020, URL: https://webbtelescope.org/contents/news-releases/2020/news-2020-38
17) Claire Blome (STScI), ”Mapping the Early Universe with NASA's Webb Telescope,” NASA Feature, 24 June 2020, URL: https://www.nasa.gov/feature/goddard
/2020/mapping-the-early-universe-with-nasas-webb-telescope
18) ”Cosmic Reionization,” URL: https://hubblesite.org/uploads/
image_file/image_attachment/32192/Webb-Eor-infographic-full_res.pdf
19) Christine Pulliam,”Webb Will Probe the Dusty Remains of Supernova 1987A,” STSI News, 28 February 2019, Release ID: 2019-13, URL: https://webbtelescope.org/articles/2019-13
20) Christine Pulliam, ”How to Weigh a Black Hole Using NASA’s Webb Space Telescope,” NASA, 17 October 2018, URL: https://www.nasa.gov/feature/goddard/
2018/how-to-weigh-a-black-hole-using-nasa-s-webb-space-telescope
The information compiled and edited in this article was provided by Herbert J. Kramer from his documentation of: ”Observation of the Earth and Its Environment: Survey of Missions and Sensors” (Springer Verlag) as well as many other sources after the publication of the 4th edition in 2002. - Comments and corrections to this article are always welcome for further updates (eoportal@symbios.space).