PAMIR (Phased Array Multifunctional Imaging Radar)
Airborne Sensors
PAMIR (Phased Array Multifunctional Imaging Radar)
PAMIR is an airborne SAR/MTI (Synthetic Aperture Radar/Moving Target Indication) experimental instrument of FGAN/FHR (Forschungsgesellschaft für Angewandte Naturwissenschaften/Forschungsinstitut für Hochfrequenzphysik und Radartechnik - Defense Research Facility for Applied Science/Research, Institute for High-frequency Physics and Radar Techniques), Wachtberg near Bonn, Germany. The multifunctional PAMIR instrument is of AER (Airborne Experimental Radar) and AER-II heritage, both of FGAN/FHR. 1) 2) 3) 4) 5) 6) 7) 8) 9) 10) 11) 12) 13) 14) 15)

The overall objective of PAMIR is to provide a technology demonstration function with the provision of enhanced capabilities such as decimeter-resolution imagery at 30 km range, long range surveillance (up to 100 km at 0.3 m resolution), MTI of < 1m/s, a large scan flexibility, and the introduction of additional operational modes: multiple Spotlight, Scan-GMTI (Ground Moving Target Indication) and high-resolution ISAR (Inverse SAR). One of the main applications of PAMIR is the demonstration of wide-area surveillance for moving targets.
The GMTI concept relies on the analysis of the received pulses at the radar instrument with respect to the Doppler effect induced by the target motion. However, when the radar is moving, the echoes of fixed targets (clutter) are also Doppler shifted, thus compete with the moving target echoes. A joint processing in space and time, referred to as STAP (Space-Time Adaptive Processing), enables to separate the echoes of the moving targets from the clutter. A prerequisite for applying the space-time processing concept is the use of a multi-channel antenna, or an implementation of a single-channel antenna with time multiplexing.
Parameter | Value | Parameter | Value |
Center frequency | 9.45 GHz (X-band) | Channels | 5 parallel receive channels |
Bandwidth | 1800 MHz | Antenna type | Active phased array |
Pixel size (resolution) | 10 cm x 10 cm | T/R modules | 256 |
Range | 30 km up to 100 km | Transmit power | 1280 W (peak) |
Azimuth scan | ±45º (electronically) | Polarization | VV (Vivaldi), HH (Horn) |
Azimuth beamwidth | 2.8º | Moving target sensitivity | ≤ 1 m/s |
Elevation beam width | 12.5º | Elevation steering | mechanically |
Operational modes | Squinted stripmap SAR, Spotlight, Scan-GMTI (STAP), ISAR | ||
Aircraft | Transall C-160 |
Phased Array Antenna Design
Normally, the demands for a system layout for high resolution SAR imaging are completely contrary to the system demands for broadband MTI operation. Except for the spotlight mode and sliding spot mode, the resolution of a SAR system can never be finer than the half of the length of the physical antenna aperture, which in turn implies a very short antenna. However, for MTI operations support, several reasons favor a large antenna length (Ref. 6):
• The minimum detectable velocity is inversely proportional to the physical antenna length and asks for a long antenna.
• The radiated power budget (radar equation) leads to a high number of T/R modules; extending the antenna in height for this reason would lead to an unacceptable small illuminated swath width.
• A third important reason is seen in the advantage that the PRF azimuth ambiguity is decreased by a large antenna length. The discrepancy between these different system demands can be solved by taking a phased array antenna and e.g. implementation of the spotlight mode, which provides a high azimuth resolution also with a very large antenna, which has finally been chosen for PAMIR. A further discrepancy between SAR and MTI demands is the simultaneous bandwidth. Detection of moving targets is optimized by adaptation of the resolution cell to the target size (~ 6m). So, for MTI mode a sample rate of 25 MHz is desirable. For high resolution SAR operation, the maximum simultaneous bandwidth of 1.8 GHz is implemented.
A linear phased antenna length of 4.25 m was selected to enable a spotlight mode with 10 cm along track resolution (Figure 2). The array is fully filled to optimize the σο (Noise Equivalent Sigma Zero - normalized scattering coefficient). The main challenge in the design of such a wideband phased array was seen in the development of a switchable TTD (True Time Delay) network which is necessary to avoid a frequency dependent beam squint, especially at large scan angles.
The bandwidth and weight constraints led to the choice of Vivaldi radiators (Figure 4) as single elements in the array. The best broadband matching was obtained with the apodally-fed Vivaldi structure. Eight elements in a column guarantee the 9.5º to 12.5º beamwidth in elevation dependent on the used frequency.
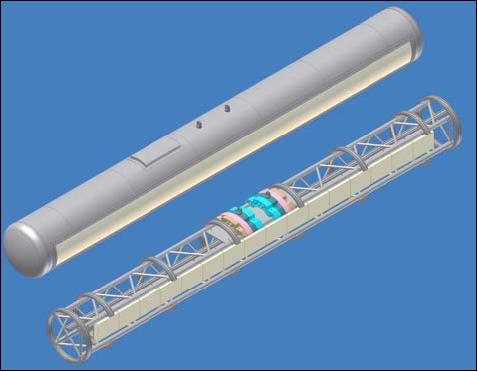
The PAMIR instrument design is modular and flexible. The electronically steerable phased array antenna system features 16 autonomous sub-arrays (panels), each of them using 16 radiators connected to a TRM (T/R Module).
The reconfigurable antenna aperture chosen for the interferometric investigations consists of three antenna subapertures (rows) with 3 subarrays in each row (Figure 3). Unequal distances between the rows reduce ambiguities. The size of the antenna aperture is adapted to the dimensions to the parachute door of the Transall C-160 aircraft, which serves as carrier platform.
To optimize the antenna elevation pattern to the slant range, the whole antenna structure can be turned mechanically in elevation by 10º additional to the 30º, by which each individual subarray is turnable.
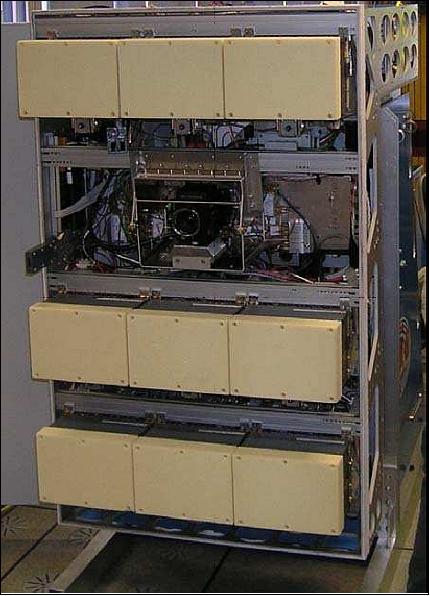
Wideband signal generation is achieved with an arbitrary waveform generator coupled to a switchable bank of five (LO (Local Oscillator) sources, permitting the generation of a 1.8 GHz bandwidth using the "stepped chirp" technique (analog combination of subapertures, the final beamforming process is done digitally within the processor). The receive chain is composed of five parallel channels ending in A/D converters with a clock rate of 400 MHz; the chain can also be operated at different subbands. Hence, the complete bandwidth can be covered by the parallel channels.
In addition, PAMIR features an integrated INS/DGPS (Inertial Navigation System/Differential GPS) motion sensor, a high-speed data recorder, various on-board calibration possibilities and a flexible object-oriented steering software. Each antenna panel can be steered separately in azimuth. A panel is composed of 16 radiating columns, T/R modules with 6 bit phase shifters and 6 bit attenuators, a TTD (True Time Delay) network, beam-formers, a power unit, and a digital interface.
An electronic beam scan with ±45º in azimuth coverage, without bandwidth dependent beam squint, implies the use of switchable true time delay networks up to the single element for high instantaneous bandwidth applications. The overall time delay of the complete RF path for TX or RX is accomplished in 2 steps: first, the time delay of the subarray, which is necessary to scan 16 single radiators over the above mentioned azimuth coverage is incorporated into the subarray structure. In a second step, the additional TTD is incorporated into a separate time delay unit. It is being used to focus the individual subarrays which may be distributed along a line with a maximum length of 4.25 m. The subarray internal TTD-line network with four switches and 7 delay lines per TTD RF path provides 16 different delay states with a time increment of 37 ps (picosecond, 1/3 wavelength).

Legend of Figure 4: View of the RF networks (from left to right) TTD combining network, GMTI combiner, T/R modules, cal combiner, and Vivaldi unit.
Subarray assembly: The subarrays are comprised of autonomous replaceable units accommodating a turnable 16-element Vivaldi unit, 16 T/R channels, each with two receiver outputs, a GMTI-RF combining network, a SAR combining network with the TTD lines in each path, the RF calibration combining network, and the control board. The antenna part of each subarray can be tilted mechanically over 30º in elevation by an individual step motor.
The complete control of all subarray related dynamic control processes is performed by a complex reprogrammable FPGA allowing a pulse-to-pulse setting change of the T/R modules and TTD networks. This feature enables flexible subaperture transmit sequences. Drift angles of the aircraft, which are measured by the INS (Inertial Navigation System), can be compensated by focus correction from pulse to pulse.

The RF networks embedding the nine subarrays in IFSAR configuration are shown in Figure 6. The GMTI and SAR receive output signals of each subarray are transferred to an RF switch matrix consisting of SP4T-, SP2T-switches and combiners, which perform mode dependent signal combinations with controlled time delay. The resulting RF matrix signals are switched to 5 identical receivers. Commanding the TTD lines is handled by the same protocol as the T/R module protocol with possible different TTD settings for transmit and receive from pulse to pulse.

System Control
The system control of PAMIR, shown in Figure 7, is adapted to the demands on an airborne multimode SAR system. The mission plan determines the position dependent focussing directions and mode dependent waveforms. The console processor calculates the time dependent steering vectors for the T/R modules and TTD networks and stores the data matrix within the direction RAM of each subarray. The GPS controlled mission timing and sequence runs completely computer controlled. INS based flight correction data lead to electronic compensation of the focusing direction. Each of the 3 subapertures got its own embedded processor PAnCo_TQM responsible for the effective data distribution to the connected subarrays (SA_AC in Figure 7), for operation security checks within the subarrays and for special calibration tasks of the radar frontend.
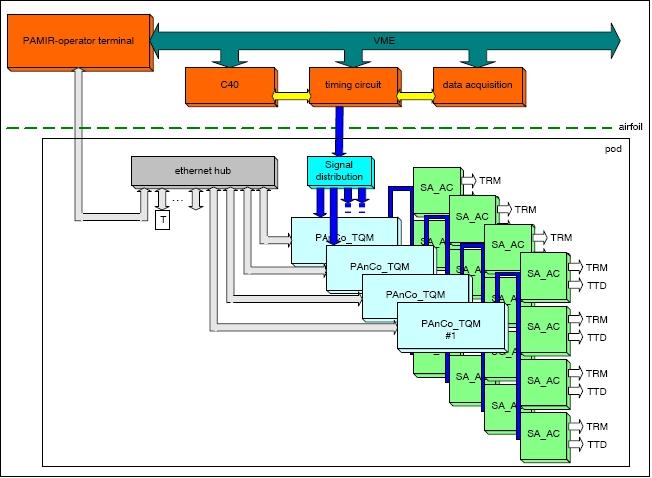
The instrument bandwidth demodulation management (of the entire bandwidth) is realized by the use of three support modes:
1) CS (Concurrent Subband) mode: The transmit pulse is generated over the entire bandwidth; the five receiving channels are tuned to five subbands covering the bandwidth in parallel. The method is used in support of high-quality very high resolution (VHR) mode requirements for high PRF (Pulse Repetition Frequency) demands.
2) De-ramp mode: The transmit pulse contains the entire bandwidth; during the receive period, a de-ramping signal is generated which may be used by each channel. The de-ramp mode is employed in small swath applications like ISAR (Inverse SAR), permitting the use of all channels in parallel for spatial processing (interferometric ISAR, ISAR with multi-aperture clutter suppression, ISAR with jammer suppression).
3) SB (Synthetic Bandwidth) mode: The bandwidth is split over a couple of pulses (e.g., 5), the combination of the subbands is done during processing. The method is used when the PRF demands permit such a support mode. The advantages of synthetic bandwidth support are: a) availability of all channels for spatial diversity, b) a high quality of the transmitted signal and the receiving signal acquisition, and c) a lower instantaneous bandwidth for the phased array antenna allowing to correct the phase-shifter settings from pulse to pulse. To avoid range ambiguities the center frequencies are chosen with no occurrence of neighboring subbands over the cycle period.
The signal generation is achieved by a two-channel arbitrary waveform generator working at a clock frequency of 1 GHz and producing 10 bit quantized I- and Q-signals. These signals are quadrature modulated to an IF (Intermediate Frequency) producing a bandwidth up to 380 MHz. The full bandwidth waveform generation is accomplished with a switchable bank of five LO sources, each with a frequency separation of 380 MHz. In this way, chirps of 1.8 GHz bandwidth can be generated using the stepped chirp principle. The storage of predefined waveform configurations permits pulse-to-pulse waveform switching. The selection of transmit pulses within a subband of 380 MHz makes the waveform generation programmable over long support periods.
The data of the receive chain are multiplexed to time-stretch memories and read out with 50 Msample/s. A formatter combines the data from the five channels with auxiliary information (e.g. motion parameters) to a standard data stream routed to a disk array of 200 GByte capacity and a continuous data rate of 60 MByte/s.
A number of operating modes can be defined with the five receive channels and the five antenna sub-apertures combinations (all of which are identical independent), such as:
• Frequency partitioning into subbands
• STAP (Space-Time Adaptive Processing) clutter suppression followed by indication and positioning of moving targets
• Single pass cross-track interferometry
• Suppression of jammer signals
• Reconnaissance of deception jammers and ambiguity suppression.
Support Mode | Bandwidth (MHz) | Resolution (cm) | Demodulation | Channels | Beam mode |
Medium resolution (MR) | 380 | 35 | 1 LO | 1 | Fixed |
MR IFSAR (Interferometry SAR) | 380 | 35 | 1 LO | 2-5 | Fixed |
Scan-GMTI (Ground Moving Target Indication) | 380 | 35 | 1 LO | 5 | Scan |
HR (High Resolution) stripmap | 740 | 18 | CS | 2 | Fixed |
VHR (Very High Resolution) spotlight | 1820 | 7 | CS | 5 | Steering |
VHR IFSAR | 1820 | 7 | De-ramp | 2-5 | Steering |
ISAR (Inverse SAR) | 1820 | 7 | CS | 5 | Tracking |
One important application of PAMIR is the detection, velocity estimation and positioning of moving targets. This task is achieved via multi-channel signal processing algorithms such as STAP (Space-Time Adaptive Processing). The relevant support mode is Scan-GMTI which allows to detect moving targets rapidly in a wide area. To illuminate a larger ground surface than in a usual fixed squint mode, the antenna is steered to another azimuth angle after each burst and therefore successively scans different portions of the ground (Figure 8).

Time delay steering: A frequency-independent electronic beam scanning with ±45º azimuth coverage of a phased array antenna without bandwidth related beam squint requires the use of switchable TTD (True Time Delay) networks up to the single element, if high instantaneous relative bandwidths (> 1.5%) are being considered. Such a TTD network has been developed and implemented in the PAMIR system. 16)
Broadband beamforming for a given focussing direction is achieved by superimposing the phasing due to subarray time delays and the phase shifter state for each radiating element in order to get a frequency independent phase front direction. The subarray time delays are in turn composed of the progressing time delays from radiator to radiator, and the common time delay for all radiators in the subarray. This time delay concept leads to the time delay network shown in Figure 9 for an individual subarray. - The sum signal of two radiators leads to the subdelay component QTTD2 delaying the necessary subarray time delay for 45º scan. After accumulation of all radiator signals in the subarray the sum signal of the subarray is time delayed in QTTD4 for achieving the total delay required for the full aperture.

Pod-Mounted PAMIR Antenna on the Transall C-160 Aircraft
PAMIR was developed to investigate sophisticated technology capabilities characterized by high flexibility and multimode operation. The tasks of this airborne system are SAR imaging at very high resolution (10 cm at a range of 30 km, and 30 cm at 100 km in spotlight mode) and high resolution ISAR imaging of ground moving targets. Further objectives of the five parallel receiving channel array antenna are: a) processing techniques like ground moving target indication (GMTI) via space-time adaptive processing (STAP), b) demonstration of ECCM (Electronic Counter-Counter Measures), c) research in cross-track IFSAR (Interferometric SAR) with high resolution capabilities, along-track interferometric SAR, and bistatic SAR Ref. 16).
The high complexity of the wing-mounted carrying structure (pod) including all frontend subsystems is seen in Figure 10, which depicts a photo of the cylindrical pod with its 16 subarray apertures. An aluminum lattice structure with a strong central aluminum plate welded between the two longitudinal pipes serves as basic carrier structure. Eight attached ring elements support the outer cylindrical aluminum skin, which considerably contributes to the stability of the complete structure of 5 m length.


Flight Campaigns of the Airborne PAMIR Instrument
In the last years several flight campaigns were undertaken providing SAR images of rural and urban areas with a spatial resolution down to 5 cm x 8 cm together with a very high dynamic range of up to 70 dB. Recently first SAR experiments using the linear antenna configuration under the wing of the Transall have been conducted.
• In August 2002 the first flight campaign was conducted. In a preliminary extension stage the sensor was operated with one receive channel and a mechanically steerable antenna array (120 W peak power) and field-tested in various modes like stripmap-, sliding-mode- and spotlight-SAR as well as ISAR (Ref. 5).
• In a second flight campaign in October 2003, the sensor was operated with three receive channels and an electronically steerable phased array. In addition to further experiments concerning SAR and ISAR, emphasis was given to the investigation of Scan-GMTI and bistatic modes (Ref. 5).
The evaluation of the acquired SAR images proved the sensor's capabilities with respect to spatial resolution, range and image dynamics. For near-range acquisition (up to 10 km) a geometrical resolution at the subdecimeter level (down to 5 cm x 8 cm) could already be achieved.
• During the flight campaign of November 2003, Scan/GMTI data were obtained with the PAMIR sensor. The preparation of the experiment and the choice of the scene were carried out to acquire data which are of particular interest for tracking. The experimental system carried by a Transall C-160 flew for approximately half an hour in an octagonal course and illuminated a scene of 6 km x 9 km in which both civilian and military vehicles were moving according to a planned scenario. The vehicles were divided into three different groups and some of them were equipped with GPS. Some of the instructions were to drive with varying velocities, to stop and to pass the other groups Ref.7).
• PAMIR flight campaign in 2005: In a first implementation, nine subarrays have been arranged in three subapertures with unequal distance for interferometric signal investigations and have been tested during a flight campaign in 2005.
• For the summer of 2008, a series of bistatic experiments are in planning between the airborne PAMIR instrument on Transall and the spaceborne TSX-SAR instrument on the TerraSAR-X spacecraft of DLR. 17) 18)
Several improvements are expected for bistatic SAR systems compared to present state-of-the-art monostatic SAR systems. The diversity of geometries in the bistatic case permits particularly adapted geometries for individual reconnaissance and surveillance tasks. In a close cooperation with ZESS (Center for Sensor Systems) at the University of Siegen, Germany, several spaceborne/airborne bistatic SAR experiments will be carried out with the TerraSAR-X satellite and the airborne system PAMIR. 19) 20)

References
1) J. H. G. Ender, A. R. Brenner, "PAMIR - A Wideband Phased Array SAR/MTI System," Proceedings of EUSAR 2002., Cologne, Germany, June 4-6, 2002, pp. 157-162
2) A. R. Brenner, J. H. G. Ender, "First Experimental Results Achieved with the new very Wideband SAR System PAMIR," Proceedings of EUSAR 2002, Cologne, Germany, June 4-6, 2002, pp. 81-86
3) J. H. G. Ender, P. Berens, A. R. Brenner, L. Rößing, U. Skupin, "Multi Channel SAR/MTI System Development at FGAN: From AER to PAMIR," Proceedings of IGARSS 2002, Toronto, Canada, June 24-28, 2002
4) H-M. J. Cantalloube, P. Dubois-Fernandez, "Airborne X-band SAR imaging with 10 cm resolution - Technical challenge and preliminary results," Proceedings of IEEE/IGARSS 2003, July 21-25, 2003
5) A. R. Brenner, J. H. G. Ender , "Airborne SAR Imaging with Subdecimeter Resolution," Proceedings of EUSAR 2004, Ulm, Germany, May 25-27, 2004
6) H. Wilden, B. Poppelreuter, O. Saalmann, A. Brenner, J. Ender, "Design and realisation of the PAMIR antenna frontend," Proceedings of EUSAR 2004, Ulm, Germany, May 25-27, 2004
7) D. Cerutti-Maori, U. Skupin, "First Experimental SCAN/MTI Results Achieved With the Multi-Channel SAR-System PAMIR," Proceedings of EUSAR 2004, Ulm, Germany, May 25-27, 2004
8) J. H. G. Ender, D. Cerutti-Maori, W. Bürger, "Radar antenna architectures and sampling strategies for space based moving target recognition," Proceedings of IGARSS 2005, Seoul, Korea, July 25-29, 2005
9) H. Wilden, A. Brenner, O. Peters, O. Saalmann, B. Poppelreuter, "New frontend configuration of PAMIR for GMTI and interferometric SAR purposes," Proceedings of EUSAR 2006, Dresden, Germany, May 16-18, 2006
10) D. Cerutti-Maori, W. Bürger, J. H. G. Ender, A. R. Brenner, "Wide Area Surveillance of Moving Targets with the SAR/GMTI System PAMIR," Proceedings of EUSAR 2006, Dresden, Germany, May 16-18, 2006
11) W. Bürger, A. R. Brenner, D. Cerutti-Maori, J. H. G. Ender, "Localisation in Ground Moving Target Indication:
Techniques and Experimental Results," Proceedings of EUSAR 2006, Dresden, Germany, May 16-18, 2006
12) J. H. G. Ender, I. Walterscheid, A. R. Brenner, "New aspects of bistatic SAR: processing and experiments," Proceedings of EUSAR 2006, Dresden, Germany, May 16-18, 2006
13) A. R. Brenner, J. H. G. Ender, "Demonstration of advanced reconnaissance techniques with the airborne SAR/GMTI sensor PAMIR," IEE Proceedings, Radar, Sonar and Navigation, April 2006, Vol. 153, Issue 2, pp. 152-162
14) H. Wilden, O. Peters, O. Saalmann, B. Poppelreuter, A. Brenner, "PAMIR with Reconfigurable Antenna Frontend," Proceedings of The European Conference on Antennas and Propagation: EuCAP 2006 (ESA SP-626), Nov. 6-10, 2006, Nice, France.
15) D. Cerutti-Maori, C. H. Gierull, J. H. G. Ender, "First experimental demonstration of GMTI improvement through antenna switching," Proceedings of EUSAR 2008, 7th European Conference on Synthetic Aperture Radar, June 2-5, 2008, Friedrichshafen, Germany
16) H. Wilden, O. Saalmann, A. Schmidt, O. Peters, A. Brenner, "A pod with a very long broadband time steered array antenna for PAMIR," Proceedings of EUSAR 2008, 7th European Conference on Synthetic Aperture Radar, June 2-5, 2008, Friedrichshafen, Germany
17) J. H. G. Ender, A. R. Brenner, J. Klare, I. Walterscheid, M. Weiss, "Bistatic exploration using TerraSAR-X and the airborne SAR sensor PAMIR," http://www.dlr.de/tsx/documentation/splinter2/MTH081.pdf
18) J. H. G. Ender, "Bistatic SAR Experiments with the TerraSAR-X Satellite and the Airborne SAR-Sensor PAMIR," URL: http://www.fhr.fgan.de/fhr/fhr_c628_f7_en.html
19) T. Espeter, I. Walterscheid, J. Klare, C. Gierull, A. Brenner, J. Ender, O. Loffeld, "Progress of Hybrid Bistatic SAR: Synchronization Experiments and First Imaging Results," Proceedings of EUSAR 2008, 7th European Conference on Synthetic Aperture Radar, June 2-5, 2008, Friedrichshafen, Germany
20) J. H. G. Ender, J. Klare, I. Walterscheid, A. R. Brenner, M. Weiss, C. Kirchner, H. Wilden, O. Loffeld, A. Kolb, W. Wiechert, M. Kalkuhl, S. Knedlik, U. Gebhardt, H. Nies, K. Natroshvili, S. Ige, A. Medrano Ortiz, A. Amankwah, "Bistatic exploration using spaceborne and airborne SAR sensors: A close collaboration between FGAN, ZESS, and FOMAAS," Proceedings of IGARSS 2006 & 27th Canadian Symposium on Remote Sensing, Denver CO, USA, July 31 to Aug. 4, 2006
The information compiled and edited in this article was provided by Herbert J. Kramer from his documentation of: "Observation of the Earth and Its Environment: Survey of Missions and Sensors" (Springer Verlag) as well as many other sources after the publication of the 4th edition in 2002. - Comments and corrections to this article are always welcome for further updates (eoportal@symbios.space).