ARMADILLO (Attitude Related Maneuvers And Debris Instrument in Low (L) Orbit)
Non-EO
Technology and Research
Space Situational Awareness
Quick facts
Overview
Mission type | Non-EO |
Launch date | 25 Jun 2019 |
ARMADILLO (Attitude Related Maneuvers And Debris Instrument in Low (L) Orbit)
Spacecraft Launch Sensor Complement References
ARMADILLO is a nanosatellite (3U CubeSat) technology demonstration mission under development at the SDL (Satellite Design Laboratory) of the University of Texas at Austin. The mission is being designed and built by students at the University of Texas at Austin (UTA) in collaboration with Baylor University, Waco, TX. The primary mission goals are: 1) 2) 3)
• Characterize in-situ sub-millimeter level dust and debris particles in LEO (Low Earth Orbit) by sensing impacts at varying times, directions and locations
• Demonstrate ionospheric radio-occultation within a single CubeSat volume (10 cm x 10 cm x 10 cm) using a software-defined dual frequency GPS receiver
• Train students in best systems engineering practices by executing a complete spacecraft life cycle from concept design to mission operations.
The project uses the PDD (Piezo Dust Detector) developed at Baylor University to study and characterize the sub-millimeter sized dust and debris particles. For the PDD, the ARMADILLO mission serves as a demonstration mission in LEO . In addition, the spacecraft will obtain radio occultation measurements by collecting GPS measurements using a dual frequency, software-defined FOTON GPS receiver developed by the Radionavigation Lab at UTA.
In order to precisely point the PDD and FOTON GPS receiver, so as to obtain the best scientific results, the ARMADILLO nanosatellite will have a precise six degree-of-freedom attitude control system. The satellite will also establish optical navigation and provide an independent verification of the ADC unit with an in-house developed star tracker, as well as have the capability to reprogram the on-board computer while in orbit. 4)
In addition to the student ARMADILLO team at UTA, a science team has been assembled consisting of representatives from:
- CASPER (Center for Astrophysics, Space Physics, and Engineering Research); Baylor University, Co-Investigators, providing the PDD (Piezo Dust Detector)
- AFRL (Air Force Research Laboratory) / Orbital Drag Program
- NASA Orbital Debris Program Office
- MIT Haystack Observatory
- Astra Space
- MIT Earth, Atmospheric and Planetary Sciences / NASA/GSFC
- NSF (National Science Foundation) CubeSat Space Weather Initiative
- TU-Delft (Delft University of Technology), Delft, The Netherlands
- Institute of Space Systems; University of Stuttgart, Germany
- Cosmic Dust Group; Max Plank Institute for Nuclear Physics, Heidelberg, Germany.

Spacecraft
ARMADILLO is a 3U CubeSat, a nanosatellite is of size: 10.5 cm x 10.5 cm x 34.5 cm with a mass of ~ 4 kg. The nanosatellite has a confirmed launch opportunity with NASA's ELaNa (Educational Launch of Nanosatellites) program and in addition to that is participating in the UNP-7 (University Nanosatellite Program) competition of AFRL/AIAA. 5)
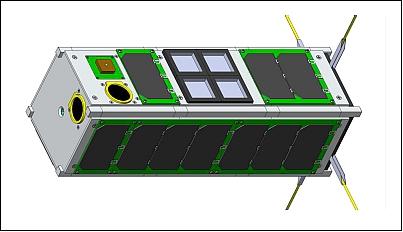

GN&C (Guidance, Navigation & Control) module: UTA/SDL is building a general purpose GN&C module, also referred to as ADCS (CubeSat Attitude Determination and Control Subsystem), with 6 degree-of-freedom maneuver capability. The GN&C module is capable of meeting multiple pointing constraints autonomously utilizing new constrained attitude control algorithms. While the GN&C module design will be tested in the Bevo-2 nanosatellite mission of NASA/MSFC (launch in 2013), the module will be reused in the ARMADILLO mission. The development of the GN&C module hardware is being supported through a NASA STTR (Small Technology Transfer) grant. 6) 7) 8)
The GN&C module has a volume of ~1U and is comprised of sensors, actuators, and embedded controllers necessary to perform 6 degree-of-freedom maneuvers (Figures 4 and 5). The system utilizes a set of 3 gyroscopes, two sun sensors, and a magnetometer (Honeywell HMR2300) for basic attitude determination. An external star tracker (not shown) provides more accurate attitude determination results when available. A set of 3 reaction wheels (Sinclair Interplanetary) are utilized for pointing control. The momentum is managed with a pair of magnetic torque rods. A compact cold-gas thruster allows for small translational impulses.
ADCS requirements:
• Three-axis stabilized spacecraft
• Rotational maneuvers: 0.1º/s –80º/s
• Pointing accuracy: 1º
• Maintain pointing: 1 orbit
• Position: ±1 km.


A new numerical optimization approach, called SDP (Semi-Definite Programming) is being used to meet the attitude requirements of the Bevo-2 spacecraft. The scenario constructed from Bevo-2 hardware requirements consists of several attitude constraints. The sensitive star tracker, which is aligned along the x-axis, must avoid the Earth, the Sun, and the Moon all with different avoidance cone sizes. The star tracker has a FOV (Field of View) of ±20º. The sun sensor, which is aligned with the y-axis (Figure 6), must maintain the Sun within the sensor's ±70º FOV. An additional attitude constraint is given to keep the spacecraft attitude within a range in which an admissible rotation exists to allow the sun sensor to view the Sun at all times.
This constraint only comes into play when the sun is within 10º of the Earth limb and takes the form of a ±20º cone in the anti-Sun direction. Additionally, the vehicle body rates are limited to 5º/s to prevent the gyroscopes from saturating, and the reaction wheels are only able to deliver up to 1 mNm of torque.

Algorithm overview: Figure 7 shows a block diagram of the new discretized constrained attitude control scheme. The algorithm begins by discretizing the unit sphere using an efficient icosahedron based pixelization subroutine. The discretization subroutine allows for translating a unit vector into an integer that identifies the pixel that is closest to that vector and vice versa. With the attitude unit sphere discretized into a graph of pixels, an admissible and short path from the starting pointing vector to the desired pointing vector is found using the A* pathfinding algorithm.14 From this path, a series of quaternions are formed that describe the rotation sequence (with adjustments to maintain sun sensor line-of-sight with the Sun). Finally, the quaternion trajectory is followed using a constrained quaternion feedback controller.
The discretized pathfinding approach to constrained attitude control presented here was designed to be general and satisfies the pointing requirements of both the Bevo-2 and the ARMADILLO 3U nanosatellite (Ref. 6).
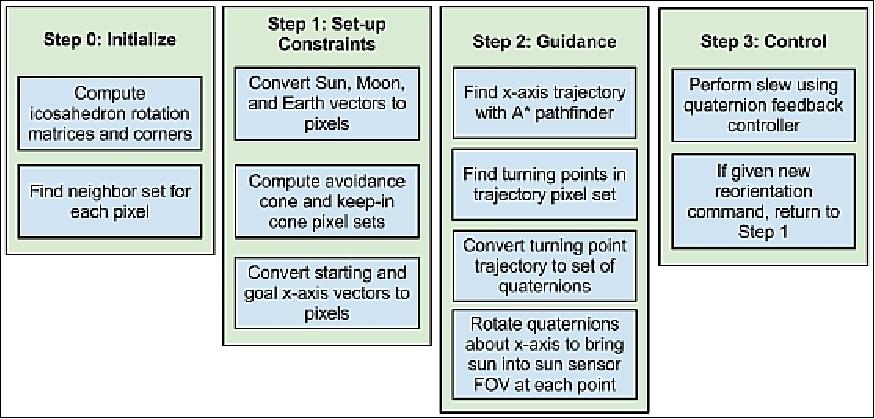
Cold-gas thruster: The thruster subsystem is responsible for providing a translational velocity change to the spacecraft. Coupled with the ADCS, the thruster subsystem allows for six degrees-of-freedom. 9)
The concept behind the propulsion system is to additively manufacture the main thruster module by means of the stereolithography process. In this manner, intricate features can be created and complex volumes can be used. This method is especially useful for small satellites. The propulsion system operates by releasing a saturated liquid propellant serially through three valves and a built-in converging-diverging nozzle. Through extensive tests, the propulsion system was measured with an Isp (specific impulse) that ranged from 65 seconds at 24ºC to over 100 seconds at 85ºC. The measured thrust force provided by the propulsion system ranged from 110 mN at 24ºC to over 170 mN at 85ºC.

CDH (Command and Data Handling) subsystem: The CDH subsystem is responsible for: 10)
- Command execution upon separating from the launch vehicle
- Managing the state of the satellite
- Communication with ground during a pass
- Data storage with redundancy
- Reprogramming FSW in-orbit.
Microprocessor: Phytec phyCORE-LPC3250 SOM (System on a Module).

EPS (Electrical Power Subsystem): The EPS generates power with about 25 UTJ (Ultra Triple Junction) surface mounted CICs (Solar Cell+Interconnects+Coverglass), 3 BCR (Battery Charge Regulators) of 2 x 8 W and 1 x 3 W, and peak power tracking. The power is distributed onboard: 11)
- Regulated bus of 5 V and 3.3 V limited at 2.5 A each
- Unregulated bus between 8.2 V and 6.2 V at 4 A
Battery: Use of 6 Varta PoLiFlex 503759C lithium polymer battery cells
- 2 batteries in series per pack
- 3 packs on battery board (2p,3s)
- ~1.19Ah per battery
- ~30 Wh of energy storage.
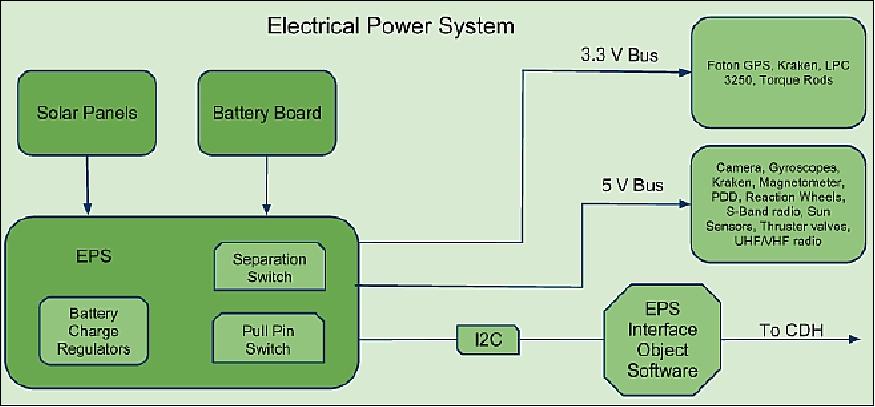
COM (Communications subsystem): COM consists of the following COTS components: 12)
- AstroDev Helium-100 UHF/VHF radio
- ClydeSpace CS-CPUT-STX-01 S-band transmitter
- ISIS deployable CDUV antenna
- Taoglas WLP.2450.24.4.A.02

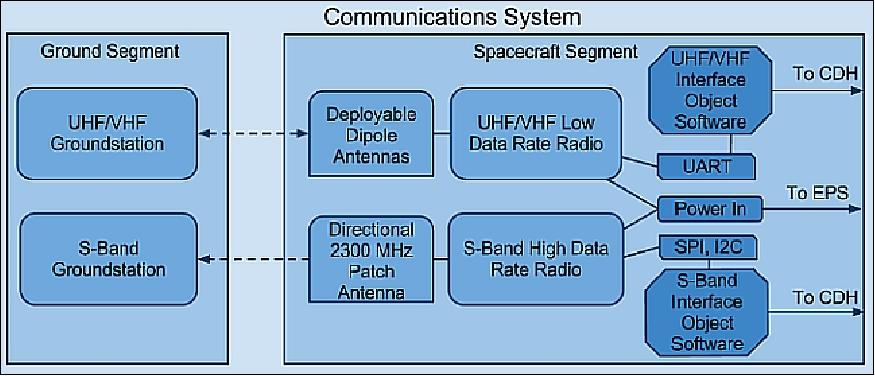
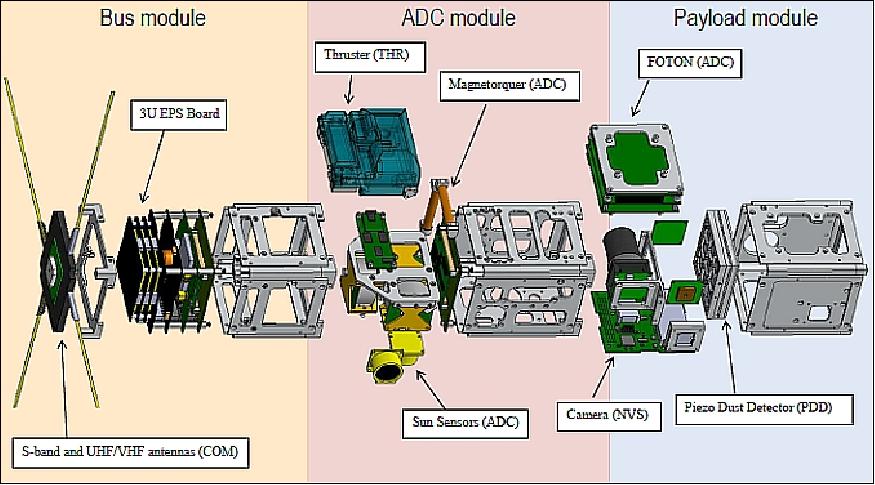
Launch
ARMADILLO is a secondary payload on the STP-2 rideshare mission of USAF, launched on 25 June 2019 (06:30 UTC) aboard a SpaceX Falcon Heavy launch vehicle from Launch Complex 39A at NASA's Kennedy Space Center. The STP-2 payload includes six FormoSat-7/COSMIC-2 satellites (primary payload, each with a mass of 280 kg), developed by NOAA and Taiwan's National Space Organization to collect GPS radio occultation data for weather forecasting. The mission also carries several NASA technology demonstrations. The STP-2 mission is led by the Air Force Space Command's Space and Missile Systems Center (SMC). The total IPS (Integrated Payload Stack) has a mass of 3700 kg. 13)
Secondary Payloads
• DSX (Demonstration and Science Experiments) mission of AFRL
• GPIM (Green Propellant Infusion Mission), a demonstration minisatellite of NASA (~180 kg). 14)
• FalconSat-7, a 3U CubeSat mission developed by the Cadets of the U.S. Air Force Academy (USAFA) at Colorado Springs, CO.
• NPSat-1 (Naval Postgraduate School Satellite-1) of the Naval Postgraduate School, Monterey, CA. A microsatellite of 86 kg.
• OCULUS-ASR (OCULUS-Attitude and Shape Recognition), a microsatellite (70 kg) of MTU (Michigan Technological University), Houghton, MI, USA.
• Prox-1, a microsatellite (71 kg) of SSDL (Space Systems Design Laboratory) at Georgia Tech.
• LightSail-2 of the Planetary Society, a nanosatellite (3U CubeSat, 5 kg) will be deployed from the parent satellite Prox-1.
• ARMADILLO of UTA (University of Texas at Austin), a nanosatellite (3U CubeSat) of ~ 4 kg.
• E-TBEx (Enhanced Tandem Beacon Experiment), a tandem pair (3U CubeSats) of SRI International.
• TEPCE (Tether Electrodynamics Propulsion CubeSat Experiment), a 3U CubeSat (3 kg) of NPS (Naval Postgraduate School).
• CP-9 , a joint CP-9/StangSat experiment, which is a collaboration between PolySat at Cal Poly and the Merritt Island High School, and is sponsored by the NASA LSP (Launch Services Program). CP-9 is a 2U CubeSat while StangSat is a 1U CubeSat.
• PSat-2 (ParkinsonSat-2), a student built 1.5U CubeSat of USNA (US Naval Academy) with a mass of 2 kg.
• BRICSAT-2, a student built 1.5U CubeSat of USNA (US Naval Academy) to demonstrate a µCAT electric propulsion system and carry a ham radio payload.
• OTB-1 (Orbital Test Bed-1) a minisatellite developed by SSTL (based on the SSTL-150 bus, 138 kg) and owned by General Atomics' Electromagnetic Systems Group (GA-EMS) of San Diego. One of the hosted payloads is NASA's DSAC (Deep Space Atomic Clock), a technology demonstration mission with the goal to validate a miniaturized, ultra-precise mercury-ion atomic clock that is 100 times more stable than today's best navigation clocks.
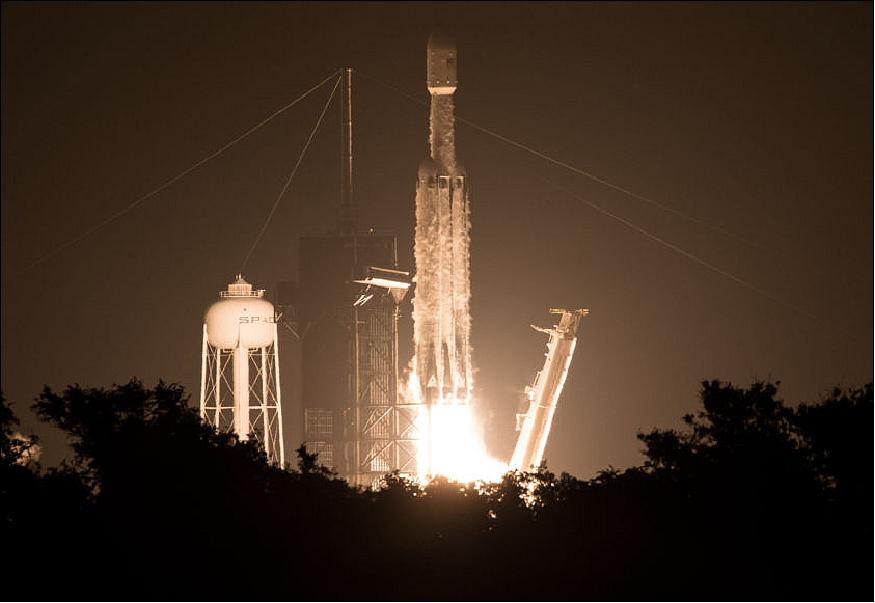
Orbits
The STP-2 mission will be among the most challenging launches in SpaceX history with four separate upper-stage engine burns, three separate deployment orbits, a final propulsive passivation maneuver and a total mission duration of over six hours. It will demonstrate the capabilities of the Falcon Heavy launch vehicle and provide critical data supporting certification for future National Security Space Launch (NSSL) missions. In addition, [the USAF] will use this mission as a pathfinder for the [military's systematic utilization of flight-proven] launch vehicle boosters.
The three orbits of the STP-2 mission for spacecraft deployment are:
1) The small secondary CubeSat satellites will be deployed into an elliptical orbit of ~300 x 860 km, inclination of ~28º. These are: OCULUS-ASR, TEPCE, E-TBEx, FalconSat-7, ARMADILLO, PSAT-2, BRICSAT, and CP-9/StangSat.
2) The second deployment batch of the STP-2 mission will occur at a circular altitude of 720 km and an inclination of 24º.
- Deployment of LightSail-2, Prox-1, and NPSat-1
- Deployment of OTB-1 with NASA's DSAC and GPIM
- The six FormoSat-7/COSMIC-2 satellites will be deployed into the initial circular parking orbit of 720 km. Eventually, they will be positioned in a low inclination orbit at a nominal altitude of ~520-550 km with an inclination of 24º (using their propulsion system). Through constellation deployment, they will be placed into 6 orbital planes with 60º separation.
3) The third and final deployment will be the Air Force Research Lab's DSX spacecraft as well as the ballast, which will be delivered to an elliptical MEO (Medium Earth Orbit) with a perigee of 6000 km and an apogee of 12000 km, inclination of 43º.
Sensor Complement
The payload module contains the following components (Ref. 5): 15)
• Primary payloads: PDD, FOTON GPS receiver and antennas
• In-house star tracker (as ADCS supplement)
• S-band radio (as COM supplement).
The payload module has a mass of 1080 g and a size of 10.5 cm x 10.5 cm x 11.5 cm.
PDD (Piezo Dust Detector)
Background
Space debris is a major concern for both government and industry space assets. With more debris posing a danger to vital communications, scientific and military satellites, cataloguing the space debris environment is a growing problem. The existing ground-based technology, however, is limited to sensing space debris particles which are larger than 1 cm. While the up-and-coming "Space Fence" will have the ability to detect smaller particles, it will primarily use this information to warn satellite operators if their satellite is in danger. However, there is an unmet need to provide in-situ data for scientists in order to characterize the space debris environment to a higher fidelity. 16) 17)
Debris is considered "trackable" if it is greater than 10 cm in diameter because it can be measured from ground-based radar systems. Unfortunately, even debris and dust particles smaller than 10 cm can cause tremendous damage to spacecraft and these particles cannot be tracked from the ground with current technology. The Aerospace Corporation explains the danger of these smaller size ranges of space debris, "An aluminum sphere 1.3 mm in diameter has damage potential similar to that of a .22 -caliber long rifle bullet. An aluminum sphere of 1 cm in diameter is comparable to a 200 kg safe traveling at 100 km/h. A fragment 10 cm long is roughly comparable to 25 sticks of dynamite."
Since these sub-millimeter size particles cannot be observed and tracked from the ground, very little is known about the particles including their composition, size and velocity. These three characteristics can tell scientists and engineers whether the particle is man-made or a natural remnant of an interplanetary object as well as the danger it could pose to orbiting satellites and science platforms.
ARMADILLO will study and characterize space debris in LEO using the PDD. In order to precisely point the PDD space debris and cosmic dust experiment to obtain the best scientific results, the ARMADILLO nanosatellite will have a precise six degree-of-freedom attitude and translational control system that includes an in-house developed cold-gas thruster. The satellite will also establish optical navigation and provide an independent verification of the GN&C unit with an in-house developed star tracker, as well as have the capability to reprogram the on-board computer while in orbit. Finally, metrics have been created and documented to fol-low the life cycle costs and design modularity used throughout the design and fabrication of this 3U CubeSat. These metrics will allow for the repeated use of a similar design by simply replacing certain payloads with other experiments (Ref. 16).
Instrument
The Center for Astrophysics, Space Physics and Engineering Research (CASPER) at Baylor University, Waco, Texas, USA, in collaboration with the Institute of Space Systems at the University of Stuttgart, Germany, and the Max-Planck Institute of Nuclear Physics, Heidelberg, Germany, is developing a low-cost miniaturized in-situ measurement instrument for dust and debris research. 18) 19)
The primary mission objective of the PDD is to perform in-situ measurements of the velocity and impact energy of sub-millimeter sized particles in various space environments in order to increase the knowledge about the sub-millimeter dust environments of comets, asteroids, planets and moons.
A secondary mission objective is the verification and validation of a modular, miniaturized dust and debris detector for future satellite or spacecraft missions. Another secondary mission objective is the collection of statistical data on the mass distribution of dust particles in various environments.
The development of PDD instrument is based on the following heritage:
• The DDS (Dust Detection System), launched on the Galileo spacecraft in October 1989, was an impact ionization detector with a sensitive area of 0.1 m2. The DDS discovered the Jovian streams and interstellar grains.
• In 1997, the CDA (Cosmic Dust Analyzer) was launched on board the Cassini mission. The hemispherical impact ionization detector with a central ion detector and a chemical analyzer target was the first instrument which combined capabilities to measure the velocity, mass, chemical composition, electric charge and direction of the particle simultaneously.
• The CIDA (Cometary and Interstellar Dust Analyzer), launched on the Stardust mission to the comet Wild 2 in February 1999, was an impact ionization detector and a time-of-flight ion mass spectrometer with a target area of 110 cm2.
• BepiColombo is a European-Japanese space project with an expected launch in July 2014 to study the planet Mercury. The MDM (Mercury Dust Monitor), which is part of the Japanese MMO (Mercury Magnetospheric Orbiter), features piezoelectric sensor elements with a sensitive area of 64 cm2 for direct impact detection. MDM was tested at the Dust Accelerator Laboratory of the Max-Planck-Institute for Nuclear Physics in Heidelberg, Germany.
Measurement principle: The PDD measurement principle is shown in Figure 15. The detector features two grids: the first is grounded to the satellite and provides electromagnetic screening. The second grid is biased with about -100 V and connected to a CSP (Charge Sensitive Preamplifier). Electrodes are vaporized on each side of the piezo element. The front electrode of the piezo elements is connected to the signal ground, while the signal is taken from the back electrode and amplified by an op-amp.
A charged particle passing the second grid generates a signal on the grid output due to electrostatic induction. The impact on the target (piezo element) deforms the piezo element, generating a signal on the piezo output. In addition, high velocity impacts will create a plasma. The charges of the plasma are separated within the capacitor formed by the target and the second grid. The positive charges are accelerated to the second grid and generate another signal on the grid output.
During the instrument operation, detection of events without an actual particle hitting the piezo elements are expected due to electromagnetic and thermal noise.

For PDD to be integrated into a 3U CubeSat, the mission requirements call for a mass of < 0.5 kg. The whole detector has to fit intro ½U, which is less than 500 cm3 and the power consumption is limited to a total of 3 W.
Design: The PDD instrument consists of two detector units, the MDU (Main Detector Unit) and the SDU (Secondary Detector Unit), both are connected to a central electronics unit, the DCU (Detector Control Unit).
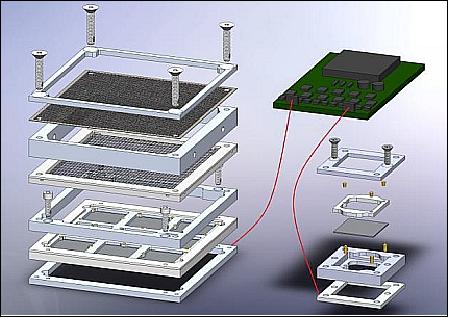
The MDU (Figure 17) consists of nine piezo sensor elements and two grids. Most of the parts of the MDU prototype are made of aluminum. The design concept of the MDU follows a stack principle of components like frames, sensor elements, insulating elements and connecting elements - without any moving or pressurized parts.
The piezo elements are COTS (Commercial-Off-The-Shelf) products at low cost. They are made of PZT-5 material and have the dimension of 21 mm x 21 mm x 0.55 mm. Both grids are made of stainless steel and have a thickness of 0.1 mm. The first grid has a transmission of approximately 82% and a spacing of 1 mm. The transmission of the second grid is approximately 86% and its spacing is 2 mm.

The design of the SDU (Secondary Detector Unit) matches the stack design of the MDU but features only one piezo sensor element without any grids (Figure 18). The same materials, piezo element, and pogo pins as for the MDU are used. The SDU is mounted on an opposite or perpendicular surface of the MDU in order to collect impact data from a different direction, e.g. deep space.
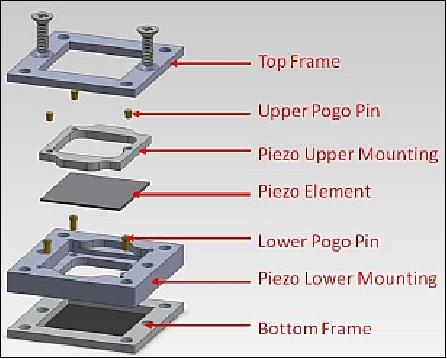
The DCU (Detector Control Unit) handles the signal capturing and processing of the PDD. The signals from the ten piezo elements and the grid are amplified by operational amplifiers (op-amp). Due to the very low signal from the grid, in addition to the op-amp, a CSP (Charge Sensitive Preamplifier) is used. The amplified, analogue, bi-polar voltage signals are converted into serial bit streams for the FPGA (Field Programmable Gate Array) processor by ADCs (Analog Digital Converters). The FPGA is used for event detection, data processing, storage and transmission as well as the communication with the on-board computer of the satellite.
The DCU prototype is powered by a +5 V supply rail provided by the satellite power supply. Therefore, additional DC-DC converters are needed to transform the +5 V into the needed supply rails for the DCU components. The DCU prototype includes one FPGA board, five piezo boards, one power supply board and one grid board.
Requirement | PDD parameters | Margin |
Mass: < 500 g | 393 g | 21.3% |
Volume: < 500 cm3 | Yes | 12.75% |
Power: 3 3 W | 2.98 W | 0.6% |
Data volume: ~ 150 kB/day | 150.1 kB/day | estimated |

FOTON (Fast, Orbital, TEC, Observables, and Navigation) GPS receiver
FOTON is a spaceborne, dual-frequency, software-defined compact GPS receiver. FOTON is of CASES (Connected Autonomous Space Environment Sensor) heritage, an earth-bound receiver, developed by the University of Texas at Austin, Cornell University, and Astra LLC. The Radionavigation Laboratory at UTA developed the space-based FOTON occultation and precision orbit determination GPS receiver. 20) 21) 22) 23)

Dual-frequency spaceborne GPS receivers have many mission-enabling capabilities as compared to their single-frequency counterparts. The ionosphere-corrected range measurement allows POD (Precise Orbit Determination) of the spacecraft to centimeter level, which has been successfully demonstrated in several space missions that have studied the Earth. Furthermore, the dual-frequency measurement allows observation of the ionospheric delay, which supports GPS radio occultation and space weather experiments. With the emergence of a second civilian GPS signal, dual-frequency measurements may be used for future real-time space applications such as autonomous formation flying, satellite networks, and cooperative control (e.g., satellite inspection and rendezvous). 24)
While dual-frequency GPS receivers have been used in space for more than two decades, the size, power, and cost of this technology is an important driver for future space missions. The growing availability of launch opportunities for very small satellites known as nanosatellites and CubeSats raises the possibility of more affordable access to space measurements if the observation quality is sufficient to support the user's needs.
Use of COTS components for FOTON:
- Bobyn RF front end
- TI C6457 digital signal processor
- Interface board (Z-Board)
- Volume: 0.5U CubeSat form factor (8.3 cm x 9.6 cm x 3.8 cm)
- Mass: 326 g
- Power: 4.5 W (current operation), <1 W orbit average power (requirement); duty cycling allows <1 W orbit average power.
FOTON software:
- Tracks GPS L1 C/A, L2C, and L5 signals
- Configurable for tracking other L-band signals
- Arbitrary number of channels, limited by data downlink.

Radio occultation observation:
• Rising/setting GPS satellite transmits through multiple layers of ionosphere
• Rising/setting GPS satellite transmits through multiple layers of ionosphere delay/total electron content (TEC).
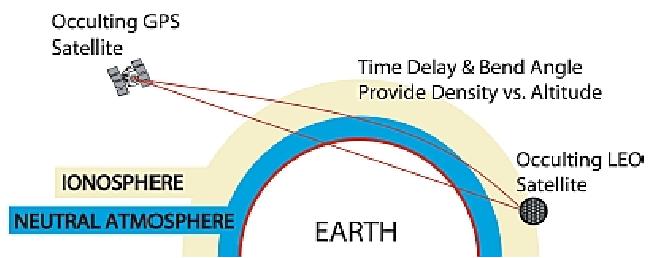
References
1) "ARMADILLO," UTA, URL: http://armadillo.ae.utexas.edu/index.php?option=com_content&view=category&layout=blog&id=7&Itemid=14
2) "ARMADILLO- System Concept Review, February 24, 2011, URL: [web source no longer available]
3) "ARMADILLO Mission Overview," UTA, March 6, 2012, URL: [web source no longer available]
4) Christopher Ryan McBryde, E. Glenn Lightsey, "A Star Tracker Design for CubeSats," Proceedings of the 2012 IEEE Aerospace Conference, Big Sky, Montana, USA, March 3-10, 2012
5) "Structures and Integration (STR)," UTA, March 6, 2012, URL: [web source no longer available]
6) Henri Christian Kjellberg, E. Glenn Lightsey, "A Constrained Attitude Control Module for Small Satellites," Proceedings of the 26th Annual AIAA/USU Conference on Small Satellites, Logan, Utah, USA, August 13-16, 2012, paper: SSC12-III-7
7) Henri Christian Kjellberg, "Design of a CubeSat Guidance, Navigation, and Control Module," Master Thesis at UTA, August 2011, URL: http://repositories.lib.utexas.edu/bitstream/handle/2152/ETD-UT-2011-08-3924/KJELLBERG-THESIS.pdf?sequence=1
8) "CubeSat Attitude Determination and Control Module," UTA, March 6, 2012, URL: [web source no longer available]
9) S. Arestie, E. G. Lightsey, B. Hudson, "Development of A Modular, Cold Gas Propulsion System for Small Satellite Applications," 2012, URL: http://lightsey.ae.utexas.edu/publications/development-of-a-modular-cold-gas-propulsion-system-for-small-satellite-applications/
10) "Command and Data Handling – ‘The Brains of the Operation'," UTA, March 6, 2012, URL: [web source no longer available]
11) "Electrical Power System- EPS, UTA, March 6, 2012, URL: [web source no longer available]
12) "Communications," March 6, 2012, UTA, URL: [web source no longer available]
13) Stephen Clark, "Falcon Heavy launches on military-led rideshare mission, boat catches fairing," Spaceflight Now, 25 June 2019, URL: https://spaceflightnow.com/2019/06/25/falcon-heavy-launches-on-military-led-rideshare-mission-boat-catches-fairing/
14) "GPIM Spacecraft to Validate Use of 'Green' Propellant," NASA, Aug. 19, 2014, URL: http://www.nasa.gov/content/gpim-spacecraft-to-validate-use-of-green-propellant/
15) "Payload: FOTON, PDD," UTA, March 6, 2012, URL: [web source no longer available]
16) Katharine M. Brumbaugh, Henri C. Kjellberg, E. Glenn Lightsey, Alexander Wolf, Rene Laufer, "In-situ sub-millimeter space debris detection using CubeSats," 35th Annual AAS (American Astronautical Society) Guidance & Control Conference, Breckenridge, Co. Feb. 3-8, 2012, AAS 12-001, URL: [web source no longer available]
17) F. Odom III, G. Richter, J. Brown, B. Martinsen, R. Cai, M. Fellows, A. Wolf, C. Montag, P. Young, J. A. Carmona-Reyes, J. Schmoke, M. Cook, B. Garner, I. Gravagne, K. Pin, L. Shedd, T. Groskreutz, T. Hegle, N. Mulenos, J. Stone, C. Wiley, V. Yanga, D. Eustice, K. Flachsbart, N. Steele, C. Tilley, P. Friudenberg, D. Penshorn, L. Henderson, E. Cavazos, A. Nabili, E. Cox, A. Cox, J. Wood, L. Devine, J. Curran, A. Mendiola, C. Falkner, R. Laufer, R. Srama, K. E. Schubert, L. S. Matthews, G Lightsey, T. W. Hyde, "Piezoelectric dust detector design and calibration for the ARMADILLO program," 46th Lunar and Planetary Science Conference (2015), The Woodlands, TX, USA, March 16-20, 2015, URL: http://www.hou.usra.edu/meetings/lpsc2015/pdf/2191.pdf
18) Alexander Wolf, Rene Laufer, Ralf Srama, Georg Herdrich, E. Glenn Lightsey, Carsten Wiedemann, Truell W. Hyde, Hans-Peter Röser, "A modular, miniaturized, low-mass in-situ dust detector for piggyback payload opportunities on small spacecraft, landers and rovers," Proceedings of the 63rd IAC (International Astronautical Congress), Naples, Italy, Oct. 1-5, 2012, paper: IAC-12-B4.8.8
19) A. Wolf, R. Laufer, G. Lightsey, G. Herdrich, R. Srama, H.-P. Röser, T. W. Hyde, "Piezo Dust Detector (PDD) - A modular miniaturized in-situ measurement instrument for dust research," 43rd Lunar and Planetary Science Conference , The Woodlands, TX, USA, March 19-23, 2012, URL: http://www.lpi.usra.edu/meetings/lpsc2012/pdf/2136.pdf
20) "ARMADILLO: Fast, Orbital, TEC, Observables, and Navigation (FOTON) Receiver," UTA, March 6, 2012, URL: [web source no longer available]
21) Andrew J. Joplin, E. Glenn Lightsey, Todd E. Humphreys, "Development and Testing of a Miniaturized, Dual-Frequency, Software-Defined GPS Receiver for Space Applications," UTA Radionavigation Laboratory, Proceedings of ION ITM, Newport Beach, California, 2012, Feb. 1, 2012, URL: http://radionavlab.ae.utexas.edu/images/stories/files/presentations/joplin_C4_pres.pdf
22) http://armadillo.ae.utexas.edu/index.php?option=com_content&view=article&id=14:foton&catid=7:mission&Itemid=15
23) Andrew Jonathan Joplin, "Development and Testing of a Miniaturized, Dual-Frequency, Software-Defined GPS Receiver for Space Applications," Master Thesis at UTA, Dec. 2011, URL: http://radionavlab.ae.utexas.edu/images/stories/files/papers/joplin_thesis_20119.pdf
24) Andrew J. Joplin, E. Glenn Lightsey, Todd E. Humphreys, "Development and Testing of a Miniaturized, Dual-Frequency GPS Receiver for Space Applications," 2012, URL: http://radionavlab.ae.utexas.edu/images/stories/files/papers/joplin_itm_2012.pdf
25) "Dual Frequency Reprogrammable Software-Defined GPS Receiver for CubeSats," UTA, URL: http://austinsat.net/datasheets/FOTON%20Spec%20Shet%20rev3.pdf
The information compiled and edited in this article was provided by Herbert J. Kramer from his documentation of: "Observation of the Earth and Its Environment: Survey of Missions and Sensors" (Springer Verlag) as well as many other sources after the publication of the 4th edition in 2002. - Comments and corrections to this article are always welcome for further updates (eoportal@symbios.space).