BeeSat-1 (Berlin Experimental Educational Satellite-1)
Non-EO
Technical University of Berlin
Quick facts
Overview
Mission type | Non-EO |
Agency | Technical University of Berlin |
Launch date | 23 Sep 2009 |
BeeSat-1 (Berlin Experimental Educational Satellite-1)
BeeSat is a student picosatellite project (CubeSat standard) under development at the Institute of Aeronautics and Astronautics (ILR) of the TUB (Technical University of Berlin), Berlin, Germany. The overall objective is to demonstrate and verify new component technologies for picosatellites, in particular the qualification of microwheels in orbit - an enabling actuation device to improve the attitude control capabilities to a new functional level in the very restricted confines of a CubeSat. 1) 2) 3) 4) 5) 6)
The main design requirements of BeeSat can be summarized as follows:
- LEO orbit in the range 450 - 850 km
- Lifetime of 1 year with a one failure tolerant design
- 3-axis stabilization, verification of microwheels in orbit
- RF communications in UHF band
- Spacecraft operations at TUB

Spacecraft
BeeSat conforms to the CubeSat standard having a size of 10 cm x 10 cm x 10 cm, and a mass limit of 1 kg, permitting a launch with the P-POD deployment mechanism of CalPoly. The spacecraft is 3-axis stabilized, utilizing “microwheels” with brushless motors and a special bearing system, designed and developed by TUB/ILR in cooperation with Astro- und Feinwerktechnik Adlershof GmbH (Astrofein), and supported by the German Aerospace Center (DLR). The objective is to obtain a flight validation of the microwheel system.
Each microwheel of the development model is having an angular momentum of at least 1.5 x 10-4 Nms with a minimum torque of 1.5 x 10-6 Nm; the size of a single device is 20 mm in diameter and 15 mm in height and a mass of 30 gram. Three or four of the microwheels are suitable for an actuator system of a picosatellite providing a 3-axis stabilization capability. The microwheels are being controlled with a common electronics board which is connected to the spacecraft bus via a CAN (Controller Area Network) interface. 7)
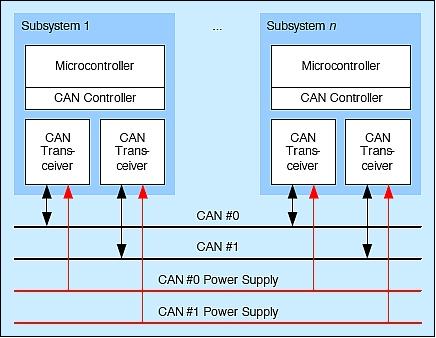

The BeeSat ADCS (Attitude Determination and Control Subsystem) utilizes 3 microwheels for 3-axis stabilization. With a total mass of 114.5 g, a torque of 4 x 10-5 Nm per wheel and a maximum power consumption of 1 W for the complete microwheel assembly including the wheel drive electronics, the qualification model of the reaction wheels exceeds the characteristics reached by the development model of Astro and TU Berlin. The small moment of inertia (1.17·x 10-5 kgm2) is sufficient for picosatellites, it adds to overall accuracy while the high revolution speed of up to 16000 rpm still allows absorption of an angular momentum that equals the BeeSat rotation rate at 7º/s.
In addition, a magnetic coil system (6 coils) is being used to desaturate the wheels and to control the satellite during orbital periods when the wheels might not be available. Attitude sensing is provided by a sun sensor system based on 6 PSD (Position-Sensitive Detector) photocells of Hamamatsu, two 3-axis magnetometers of Honeywell (HMC 1053), and 3 gyroscopes. The ADCS software supports 4 main attitude modes: a) inertial pointing, b) maximum power, c) Earth pointing, and d) rotation mode (as well as intermediate modes).

Note: Most new technologies of ADCS (not the microwheels), along with the algorithms, were demonstrated and validated in TUPEX (TU Berlin Picosatellite Experiment), a payload flown in a sounding rocket campaign of DLR and SSC (Swedish Space Corporation), referred to as REXUS (Rocket-borne Experiments for University Students). The launch of REXUS took place on April 5, 2006 in Kiruna Sweden. - On the main board, three gyroscopes were measuring the angular velocity of the rocket in three perpendicular axes. Two accelerometers did the same for linear accelerations, each measuring along two axes. A 3-D magnetic field sensor was integrated to identify changes in the Earth's magnetic field during the flight. Installed on separate PCBs on the rocket's surface, prototypes of 2-D sun sensors and triple-junction GaAs solar cells could benefit from direct sunlight. 8)

The OBDH (Onboard Data Handling Subsystem) utilizes two redundant units based on an ARM7 microcontroller (Philips LPC2292), a 16 MB flash memory for program code (1 MB for telemetry data and 2 MB SRAM), and a redundant CAN bus interface for the communication with the microwheels.
The OBDH employs the TinyBOSS operating system of FIRST (Fraunhofer Institute of Computer Architecture and Software Technology), Berlin. TinyBOSS is an adapted version of BOSS, a real-time operating system being successfully flown on the BIRD (Bi¿Spectral Infrared Detection) microsatellite mission of DLR (launch Oct. 22, 2001).
Note: the acronym BOSS stood originally for “BIRD Operating System (Simple)” - it is now being used for many other applications, not only for those in space. 9)
Power is being provided by surface-mounted solar cells (1.36 W min at EOL). Four Li-ion battery cells are being used for spacecraft operations during the ecliptic phase of the orbit. The power bus provides a voltage of 3.3 V and 5 V to the S/C subsystems, utilizing DC/DC converters. The average power consumption is 0.5 W.
The OBC, also referred to as MCU (Mission Control Unit), is being implemented in cold redundancy (with a watchdog and circuit breaker, current limiter, and several software images). The main features of the OBC are:
- MCU: NXP LPC2292, 60 MHz
- 2 MByte SRAM, 20 MByte flash memory
- 48 measurement acquisition channels with 12 bit resolution
- Typical electrical power consumption: 150 mW.
BeeSat is the first picosatellite implemented with a fault-tolerant design (with exception of experimental payloads).

The spacecraft frame is made of an aluminum quadrate tube structure, featuring good stability and permitting a simple assembly of the structure.
RF communications: BeeSat employs a redundant communications system using 0.5 W of output power on the UHF amateur radio band (435-436 MHz). The system is designed for a bit rate of 4.8 or 9.6 kbit/s and GMSK (Gaussian Minimum Shift Keying) modulation. The uplink has a bit rate of 4.8 kbit/s. In addition to the nominal telemetry and telecommand functions, the implementation of a digipeater service and a beacon signal (call sign DP0BEE on CW) has been approved. The communication protocol uses the CCSDS standard. BeeSat will be operated by students from the TUB control center.
Also, a miniaturized highly efficient S-band transmitter was developed for picosatellite applications. The development was in cooperation with IQ-Wireless and TUB with funding support from DLR.
Frequency, modulation | S-band, DQPSK (Differential Quadrature Phase Shift Keying) |
Coding, bit rate | Turbo code, up to 1 Mbit/s |
Instrument dimensions | ca. 95 mm x 50 mm x 15 mm |
Instrument mass, power | 0.1 kg, 1 W |
BER (Bit Error Rate) | 10-5 |



Launch
BeeSat was launched as a secondary payload on Sept. 23, 2009 on a PSLV launcher from SDSC¿SHAR on the east coast of India. Use of the SPL (Single Picosatellite Launcher) of Astro- und Feinwerktechnik Adlershof GmbH (Berlin) for the deployment of the CubeSats. Further secondary payloads on this flight were:
• SwissCube, a CubeSat of Ecole Polytechnique Federale de Lausanne, Lausanne, Switzerland
• ITUpSat (Istanbul Technical University PicoSatellite-1), Istanbul, Turkey.
• UWE-2 (University of Würzburg Experimentalsatellit), Würzburg, Germany
• Rubin-9.1 and Rubin-9.2 nanosatellites of OHB-System, Bremen, Germany
The primary payload on this flight (PSLC-C14) was OceanSat-2 of ISRO (Indian Space Research Organization) with a launch mass of 960 kg. 10) 11)
Orbit: Sun-synchronous near circular orbit, altitude = 720 km, inclination = 98.28º, period = 99.31 min, the local equatorial crossing time is at 12:00 hours.
Mission Status
• In 2012, the BeeSat -1 spacecraft and its payload are operating nominally. While the nominal mission was successfully completed after one year of on-orbit life, the satellite is still being operated for technology tests; in addition, the operational service environment offers an excellent training platform for the student body (Ref.13).
• On July 22, 2011, the project detected telemetry data anomalies. BeeSat had entered safe mode of operation automatically and was still functional in this mode. After examination of the fault scenario, which was cross-checked with our engineering model a couple of days, the project decided to activate the cold redundant on-board computer. The controlled switching took place on July 29, 2011. Although all telemetry data are plausible again, the project continues to investigate the cause of the malfunction. 12)
• In 2011, BeeSat is operating nominally. The spacecraft telemetry is being acquired almost daily (on weekdays). So far, there were no events of failures. The reaction wheels are being tested periodically. No performance degradations of subsystems can be detected. The spacecraft is on orbit since Sept. 23, 2009, well beyond the mission design life of 1 year. 13)
• In early June 2010, BeeSat has been on orbit for more than eight months and is operating nominally. After successfully completing a large number of reaction wheel tests and numerous experiments with BeeSat's hard- and software during normal daily satellite operations, time has come to test on-board autonomy. The satellite spent on orbit four weeks without any ground control operations. It was important for the project to execute and demonstrate the system stability and overall survival capability of BeeSat.
Now that daily ground station contact has been re-established and no damage whatsoever was observed, it has been verified that BeeSat is quite capable of handling longer periods without any intervention from the ground. The on-board autonomy was demonstrated successfully. 14)
• As of late April 2010, the RW-1 (RW-B), the world`s smallest reaction wheel assembly, has seen ~ seven months of successful operation in space on BeeSat. Astrofein provides with RW-1 the first spaceborne proven precise actuator system for picosatellites. 15)
• On Nov. 16, 2009, BeeSat is now 55 days in orbit and has been contacted daily by TU Berlin's ground station. The miniaturized reaction wheel system is being tested regularly (Ref. 14).
• Telemetry of BeeSat was received on the first pass over Berlin with a maximum elevation of 7.8º.
Sensor Complement
Micro-camera
The payload consists of a micro-camera providing VGA (Video Graphics Adapter) snapshot imagery of the Earth's surface in the visible range. The CMOS detector has a size of 1600 x 1200 pixels. Implemented as SoC (System-on-Chip), it provides windowing and JPEG compression. The sensor itself is complemented by an 8 bit microcontroller equipped with 1 MB of SRAM for configuration and time critical operations of the detector.
The imagery is being transferred and stored in a non-volatile memory of the PDH (Payload Data Handling) system and transmitted to the ground during station passes. Hence the PDH is nearly identical to BeeSat's on-board computer (OBC) architecture, it is able to store multiple images, provide housekeepings, and perform limited data processing.
RW-1 (Reaction Wheel-1)
AFW (Astro- und Feinwerktechnik GmbH) of Berlin Adlershof (also referred to as Astrofein) designed and developed in cooperation with TUB and Magson GmbH a tiny reaction wheel - a precise actuator assembly to solve the problem of CubeSat and nanosatellite stabilization. Conceptually, this had to be the smallest RW anywhere to fit into the AOCS of a 1U CubeSat. AFW and Magson started work on the challenging actuator project in 2006 with the idea of creating a COTS (Commercial off-the-Shelf) product, referred to as RW-1, for the CubeSat market. The project was sponsored by the German Aerospace Center, DLR and funded by BMBF (Ref. 15). 16)
RW-1 is a highly integrated micro-reaction wheel assembly with an external WDE (Wheel Drive Electronics) device for controlling up to four wheels. The first implementation (prototype) and spaceborne functional demonstration/qualification of RW-1 is RW-B (Reaction Wheel BeeSat). The RW-B EM (Engineering Model) was delivered to TUB in January 2007 (Figure 5).
The RW-1 is able to control speed and acceleration of the connected wheels in a closed loop PI (Proportional Integrator) control circuit (PID control loop is available). It is an adaptable reaction wheel system with different options for the technical details. Depending on customer requirements it can be equipped with two different rotation masses (type A and type B).
Type A is able to store an angular momentum of 5.8 x 10-4 Nms. It is ideal for pico- and nanosatellites. Type B can store only an angular momentum which is six times smaller; it is designed for precise pointing of picosatellites. To support different types of data interfaces of pico- and nanosatellites, the customer can choose between four different standards (CAN2.0, SPI, RS232 and RS485).
A typical RW-1 reaction wheel system consists of one to four wheels (mostly used in a tetrahedron configuration as shown in Figure 10) and an external WDE.

Parameter | Type A | Type B |
Angular momentum | 5.8 x 10-4 Nms | 1.0 x 10-4 Nms |
Nominal rotation speed | 8,000 rpm | |
Maximum rotation speed | > 16,000 rpm | |
Resolution | 0.25 rpm (1 LSB) | |
Speed deviation (rms) | 5 rpm | 8.5 rpm |
Nominal torque | 23 x 10-6 Nm | 4 x 10-6 Nm |
Torque deviation (rms) | < 2.2 x 10-6 Nm | < 0.8 x 10-6 Nm |
Mass (wheel) | ≤ 24 g | ≤ 12 g |
Mass WDE (Wheel Drive Electronics) | ≤ 40 g | |
Wheel size | Ø 21 mm x 12 mm | |
WDE size | 95 mm x 50 mm x 15 mm | |
Moment of inertia | 694.5 g mm2 | 119.4 g mm2 |
Voltage | 5 V | |
Power (single wheel nominal speed) | < 0.62 W | |
Power consumption (standby) | < 0.4 W | |
Power consumption max (single wheel) | < 0.72 W | |
Data interfaces | CAN2.0, SPI, RS232, RS485 | |
Operations temperature | -20 to +50ºC |
A schematic illustration of the wheel is shown in Figure 11. The wheel is driven by a brushless DC motor, designed as a flat runner. The stator coils are part of a PCB (Printed Circuit Board) which also contains the feedback sensors. The permanent magnet of the rotor is included into the flywheel mass. The motor and the flywheel mass are surrounded by a housing which contains the bearings, which are specially designed for operation under the extreme conditions of the space environment.

The functional block diagram of the WDE is shown in Figure 12. The main component of the WDE is the FPGA, which contains the control core (the intelligence) of the reaction wheel. It is a standard PI (or PID) controller which is able to control speed and acceleration of the wheels. The PI controller drives the power electronics, another main component of the WDE, which supplies voltage and current to the wheels. The measured wheel speed signals will be lead to the WDE. Furthermore there is a signal processing unit which pre-processes the speed signals and transmits them to the FPGA. The speed data is being interpreted in the speed measurement module of the FPGA. Additionally, there are other peripherals like transceivers and an EEPROM on the WDE.

The initial flight of the RW-1 (RW-B) was on BeeSat (launch Sept. 23, 2009). The AOCS of BeeSat was comprised of the following components: 6 sun sensors, 2 magnetometers and 3 gyros for attitude determination. Actuation was provided by a 3 reaction wheel configuration (RW-B implementation) and 6 magnetic coils. The reaction wheels were arranged perpendicular to each other. The RW-B assembly with WDE has a mass of 150 g.
The first wheel operation was carried out on Sept. 30, 2009. Because of the system layout, the BeeSat reaction wheels were initially operated only when in contact with the ground station. A speed and acceleration profile for a standard wheel test is integrated into the onboard data system. The test was activated via ground station command.
For the checkout of the wheels, a speed and acceleration profile was commanded to the wheels and compared with the reaction of the satellite. The housekeeping data of RW-B and the data of the attitude sensors of BeeSat were analyzed and compared.
In late April 2010, after more ~ seven months of spacecraft operations, the RW-B was performing nominally. Although COTS components were integrated into the WDE, no degradation or any interference of the operations has been observed so far. All performance parameters (power consumption, torque level, speed deviation, etc.) displayed nominal behavior and were within their boundary conditions. A typical RW-B performance curve is shown in Figure 13.

Aside from the qualification aspects of the RW-B assembly, the instrument was already used to de-spin the initial BeeSat rotation after deployment. BeeSat was separated on orbit with an angular rotation of ~20º/s. This rotation was decreased to < 2º/s in late January 2010, using the magnetic coils as well as the support of RW-B.
References
1) K. Kayal, K. Briess, “Pico and Nanosatellite Technologies at the Technical University of Berlin,” Proceedings of the 56th IAC 2005, Fukuoda, Japan, Oct. 17-21, 2005, IAC-05-B5.6.B.03
2) https://www.raumfahrttechnik.tu-berlin.de/menue/forschung/aktuelle_projekte/beesat/v_menue2/project_overview/
3) H. Kayal, K. Brieß, U. Renner, “Small Satellites from Berlin,” Proceedings of the Asian Space Conference 2007, Nanyang Technological University (NTU), Singapore, March 21-23, 2007
4) M. Herfort, M. Berlin, H.-P. Geile, Z. Yoon, “BeeSat Attitude Determination and Control System,” Proceedings of the 6th IAA Symposium on Small Satellites for Earth Observation, Berlin, Germany, April 23 - 26, 2007
5) Hakan Kayal, Frank Baumann, Klaus Briess, “BeeSat - A pico satellite of TU Berlin for the in-orbit verification of miniaturized wheels,” Proceedings of the 59th IAC (International Astronautical Congress), Glasgow, Scotland, UK, Sept. 29 to Oct. 3, 2008, IAC-08.B4.4.B10
6) Frank Baumann, Klaus Briess, Hakan Kayal, “BEESAT - A Fault-tolerant Picosatellite Approach,” Proceedings of the 7th IAA Symposium on Small Satellites for Earth Observation, Berlin, Germany, May 4-7, 2009, URL of presentation: http://media.dlr.de:8080/erez4/erez...
7) H. Kayal , F. Baumann, K. Briess, M. Herfort, “Verification of Miniaturized Reaction Wheels for Pico and Nano Satellites,” 6th ESA Round Table on Micro & Nano Technologies for Space Applications, October 8-12, 2008, URL: https://escies.org/download/webDocumentFile?id=7219
8) S. Trowitzsch, F. Baumann, H. Birke, R. Grewe, H. Kayal, D. Richter, “TUPEX - An Experimental Sounding Rocket Payload for Evaluation of Pico Satellite Technologies,” Space Technology Education Conference, May 9-12, 2006, Braunschweig, Germany
9) Sergio Montenegro, Klaus Briess, Hakan Kayal, “Dependable Software (BOSS) for the BeeSat Picosatellite,” 2006, URL: http://montenegros.de/sergio/public/beesat_dasia2006_final.pdf
10) “PSLV-C14 Successfully Launches Oceansat-2 Satellite,” ISRO, Sept. 23, 2009, URL: http://www.isro.org/pressrelease/scripts/pressreleasein.aspx?Sep23_2009
11) “ISRO's PSLV launches Oceansat-2, 6 nano satellites,” Sept. 23, 2009, URL: http://netindian.in/news/2009/09/23/0003458/i...
12) “Activation of Redundant On-board Computer,” Aug. 1, 2011, URL: https://www.raumfahrttechnik.tu-berlin.de/menue/forschung/aktuelle_projekte/beesat/v_menue2/beesat_1/news/?
13) Information provided by Klaus Briess of TU Berlin.
14) “Eight months on orbit - Test of resilience,” June 1, 2010, URL: http://www.raumfahrttechnik.tu-berlin.de/beesat/v-menue2/beesat-1/news/
15) S. Roemer, S. Stoltz, F. Baumann, H. Kayal, “RW-1 the Worldwide Smallest Commercial, Flight Proven Reaction Wheel for Pico- and Nanosatellites - Fields of Operation and Flight Results,” Proceedings of the Symposium on Small Satellite Systems and Services (4S), Funchal, Madeira, Portugal, May 31-June 4, 2010
16) Karsten Großekatthöfer, Gudrun Wahnschaffe, Zizung Yoon, Christian Raschke, “Modellgestützte Entwicklung eines Lageregelungssystems,” Munich, Oct. 7, 2009, URL: http://www.dglr.de/fileadmin/inhalte/...
The information compiled and edited in this article was provided by Herbert J. Kramer from his documentation of: ”Observation of the Earth and Its Environment: Survey of Missions and Sensors” (Springer Verlag) as well as many other sources after the publication of the 4th edition in 2002. - Comments and corrections to this article are always welcome for further updates (eoportal@symbios.space).