CXBN-2 (Cosmic X-ray Background Nanosatellite-2)
Non-EO
NASA
Astronomy and Telescopes
Quick facts
Overview
Mission type | Non-EO |
Agency | NASA |
Launch date | 18 Apr 2017 |
End of life date | 01 Mar 2019 |
CXBN-2 (Cosmic X-ray Background Nanosatellite-2)
Background Spacecraft Launch Mission Status Sensor Complement References
CXBN-2 is under development by MSU (Morehead State University) of Morehead, KY, USA) as a follow-on mission to CXBN, a successful 2U CubeSat that was launched on September 13, 2012 as a secondary payload on the NASA ELaNa-6 (Educational Launch of Nanosatellites-6) Launch Services Program with the primary OUTSat (Operationally Unique Technologies Satellite) mission of NRO. 1)
The goal of the CXBN-2 science mission is to significantly increase the X-ray measurement precision in the 30-50 keV range, thereby constraining models that attempt to explain the relative contribution of X-ray sources. Results from the mission will lend insight into the underlying physics of this phenomenon.
Background: The mission addresses a fundamental science question that is clearly central to our understanding of the structure, origin, and evolution of the universe by potentially lending insight into both the high energy background radiation and into the evolution of primordial galaxies. CXBN-2 will map the extragalactic diffuse CXB (Cosmic X-ray Background) with a new breed of CZT (Cadmium Zinc Telluride) detector (first flown on CXBN) but with twice the detector array area of its precursor and with careful characterization and calibration. The CXB is a powerful tool for understanding the early universe and provides a window to the most energetic objects in the far-away universe. Although studied previously, existing measurements disagree by about 20%. With the novel CZT detector aboard CXBN-2 and an improved array configuration, a new, high precision measurement is possible. In ~1 year of operation the experiment will have collected 3 million seconds of good data, reaching a broadband S/N ~250.
The isotropy of the Cosmic X-ray Background greater than 3 keV strongly suggests that it is extragalactic. The CXB in this range is thought to be composed of a very large number of discrete sources. At soft X-ray energies, the nature of these sources is known, namely faint AGN (Active Galactic Nuclei). However, theoretical attempts to synthesize the CXB spectrum at hard X-ray energies from an AGN population have not been very successful. Part of the difficulty is that the existing measurements are inconsistent with each other, and the one widely considered the "best" [made by the A4 instrument on HEAO-1 (High Energy Astronomy Observatory) of NASA with a launch on Aug. 12, 1977] does not match other measurements. While the NuSTAR (Nuclear Spectroscopic Telescope Array) mission of NASA (launch June 13, 2012) operates in approximately the same energy range, it is not intended to make this specific measurement and would suffer from the systematic effect of secondary particles created by cosmic ray particles interacting with the substantial spacecraft structure, leading to a less precise measurement.
The hard x-ray band is key to understanding the CXB because the bulk of the energy is found over this energy range. The CXB flux peaks at ~30 keV, so the CXBN-2 measurements in the 30-50 keV range cover the peak of the power spectrum. At these energies, genuinely diffuse Galactic emission is small but bright point sources in our galaxy and in the Magellanic Clouds are potential contaminators. The best-measured component of the X-ray Background spectrum is the 3-300 keV band (i.e. 4-0.04 Å). The HEAO-1 A2 experiment provided the best measurements to date of the X-rayBackground spectrum from 3 to about 45 keV.
There is a significant conflict in the nominalization measurements of the background in the 30-50 keV range, leading to a large uncertainty (i.e. 20-30%) in the reported values. These uncertainties do not allow meaningful constraints between various models to explain the underlying physics. The CXBN-2 mission will lead to significantly increased precision in the CXB flux in this range with a goal of measuring this flux with a precision of <5% thereby constraining the existing models and lending insight into the physics of the early universe.
Spacecraft
Fortunately, the science mission requirements fit within the nanosatellite class (2U CubeSat) spacecraft. The science measurements that will be performed with CXBN-2 represent an improvement over the initial CXBN mission owing to four enhancements: 2)
1) The addition of a second CZT array doubles the array area representing a √2 increase in statistics and increased reliability through redundancy.
2) Careful characterization of the CZT arrays on the ground in pre-flight. Hot pixels will be eliminated and the energy response of the array will be mapped allowing more effective interpretation of the science data.
3) Careful calibration of the array for both flux and gain using calibrated Americium 241 sources on the ground and on-orbit.
4) Extensive GEANT (GEometry ANd Tracking) simulation of the contribution of secondary particles from cosmic ray protons interacting with the spacecraft structures that will be reduced from the data to improve the accuracy of the measurements.
All of the major subsystems comprising the satellite are highly evolved and have flight heritage—having been developed by the team for other missions, and having flown on the first CXBN mission. CXBN-2 is a 2U CubeSat that can share space with a separate 1U CubeSat in a standard 3U CalPoly P-POD (Picosat Orbital Deployer). CXBN-2 has a total mass of 2.6 kg. Innovative systems include upgraded versions of the CXBN PMD (Power Management and Distribution) subsystem, C&DH (Command and Data Handling) subsystem — now based on a Cortex M4 processor, and an innovative ADCS (Attitude Determination and Control Subsystem).

The CXBN-2 subsystems have been flight proven on the CXBN OUTSat mission. The heritage systems include: EPS (Electrical Power Subsystem), RF communications, ADCS , and C&DH. The combination of flight qualified hardware along with improvements to systems based on the CXBN mission experience significantly reduces the risk associated with the CXBN-2 mission.
Concept of Operations
CXBN-2 is a spin-stabilized, sun-pointing 2U CubeSat with deployable solar panels producing 15 W of continuous power. The required orbit is a relatively high inclination (>35°) orbital plane at approximately 700 km altitude. Four contacts with the MSU Earth station are expected per day, allowing 40 MB of data to be downlinked per day at S-band. A link margin of greater than 10 dB is anticipated for 10 º elevation passes. Satellite Tool Kit (AGI) was used to develop initial dynamical models including all orbit parameters, communication link budgets, and spacecraft dynamics.
CXBN-2 will have several different modes of spacecraft operation. They include failure recovery, standby/uplink, downlink, and full science modes. The failure recovery mode will be a most conservative mode which attempts to minimize power usage by emitting a low power CW beacon while waiting for ground communications to perform debugging. The standby/uplink mode will broadcast a beacon with a complete telemetry set and CW padding to better accommodate tracking. In downlink mode the spacecraft will send current science data and historical data to the primary ground station for evaluation.
Spacecraft structure: The CXBN-2 bus structure is based on a robust 2-wall structure matched to a central mounting block. Subsystem enclosures and braces reinforce the structure throughout the length of the body, allowing the frame to hold very tight tolerances better than ±0.1 mm. The design also compromises between maintaining rigidity during vibration and ease of access during subsystem integration. The in-house manufacturing and rapid-prototyping capabilities facilitate spacecraft structure assembly and test within a schedule measured in months rather than years.
ADCS (Attitude Determination and Control Subsystem): The pointing requirement to satisfy the science mission conditions are minimal — the spacecraft is required to point the -Z axis at the Sun and spin around it. The spacecraft will maintain a slowly precessing spin about the Z-axis that will be controlled with magnetic torque coils. The -Z face will include sun sensors. With careful calibration, magnetometers should be adequate to determine the spin phase. A bright star sensor (Canopus Pipper) coaligned with the instrument axis will also be included in the ADCS. The spacecraft also deploys four solar panels at a 90° angle relative to the X-axis of the spacecraft; the weighted ends of the panels will provide additional spin stabilization. 3)
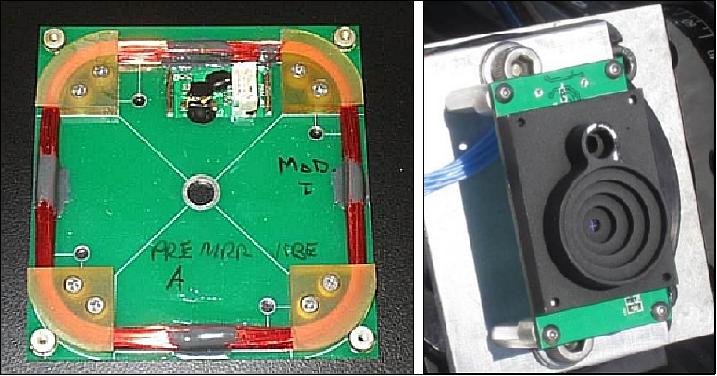
EPS (Electrical Power Subsystem): Attached to the aft end of the satellite structure are four hinged, doublesided, solar panels on which are mounted a total of 48 triple-junction 30% efficient solar cells. These panels are deployed at 90° to maximize the incident solar flux and to provide spin stabilization. The engineering state of the solar panel array (Figure 3) and deployment mechanism is highly evolved, being based on the first CXBN design. The system will produce 15 W of continuous on-orbit prime power providing a significantly positive power budget. The PMD (Power Management and Distribution) subsystem is a direct energy transfer system that has its own MSP-430 microprocessor allowing on-orbit programming. It also complies with the CubeSat standards having integrated remove before flight and deployment switch circuitry. The Li-ion 18650 batteries selected have been shown to have a self-discharge rate of 3.3% per month which will reduce risk associated with the potential on-the-shelf storage time often associated with NASA ELaNa secondary payload missions.
Retooling Space Solar Cell System CIC (Solar Cell Interconnects Coverglass) for CXBN-2. The objective is to satisfy the small satellite system requirements while having the most effective surface area and providing the necessary wattage. 4)

C&DH (Command and Data Handling) subsystem: The C&DH architecture is distributed to some extent. The science payload, PMD (Power Management and Distribution) subsystem and the radios each have their own microcontroller. The "flight computer" C&DH system comprises the main processor which collects and reduces data from the flight subsystems. The payload interface is a combination of analog to digital and digital capture circuitry to support the science goals of the mission. The main processor is an ARM Cortex M4 architecture which consumes minimal resources in standby power modes while having significant processing capacity during full operation.
The advantage of the C&DH system is its capability to operate a full operating system which limits the amount of embedded programming required for the full spacecraft mission. All drivers are written in high level C and C++ and we have extensive libraries available for supporting complex math, I/O stacks, and data transfer. Additionally, a BSL (Bootstrap Loader) allows new code to be uploaded to the processor, the payload, and the PMD while on orbit. The latter represents a significant improvement in CubeSat technology and operations concepts where the spacecraft becomes an extensible platform.
RF communications: CXBN-2 has two transceivers (UHF and S-band) that provide high throughput downlink utilizing the Morehead State 21 m antenna and low bandwidth uplink and downlink that can utilize amateur radio equipment and take advantage of the world-wide amateur radio operators' network. The two on-board radios are AstroDev Lithium-1 and Berylium-1 radios.
The UHF system contains all of the necessary processing to act as a TNC (Terminal Node Controller) for decoding AX.25 amateur packet protocol for uplink and downlink. The S-band system is a customized protocol with larger packet sizes and reduced packet overhead in comparison to AX.25.
The CXBN-2 UHF antenna is a quadrature array system is comprised of traditional spring steel and stowed by the deployable solar panel system, which is driven by a phase matching circuit that provides circular polarization. This design overcomes a major challenge of CubeSat developers related to the small electrical surface (ground plane) of the satellite bus relative to lower frequency communications. This system represents a significant improvement in CubeSat communications systems compared to dipole or monopole systems.
With a mass of about 2.6 kg, the CXBN-2 CubeSat has dimensions of 10 x 10 x 20 cm and is fitted with four deployable solar arrays capable of generating up to 15 W of power. The satellite incorporates a power distribution and handling system known as PMD, a command and data handling system (C&DH) based on a Cortex Arm processor, and an innovative ADCS (Attitude Determination and Control Subsystem) developed at MSU.
In comparison with the first CXBN mission which was sent into space in September 2012, the CXBN-2 CubeSat has two 256 pixel arrays instead of one. Moreover, it features an innovative 3-D printed Tungsten collimator, a series of improvements to the spacecraft bus, and an innovative conops characterized by a free flying minimally spinning spacecraft.

The satellite's flight and engineering models were completed in the Fall of 2016 and passed flight qualification testing. The flight unit was delivered to the launch integrator, NanoRacks, in December 2016 and was subsequently shipped to the Kennedy Space Center in Florida.

Launch
The CXBN-2 CubeSat was launched as a secondary payload on the Orbital ATK Cygnus CRS (Commercial Resupply Service) OA-7 mission on April 18, 2017 on an ULA Atlas-5 401 vehicle from SLC-41 (Launch Complex-14) at the Cape Canaveral Air Force Station in Florida. 6)
Orbit: Near-circular orbit, altitude of ~ 400 km, inclination of 51.6º (β angle variation: 0-75º).
Secondary payloads (CubeSats): 7)
• Altair-1, a 6U CubeSat technology demonstration mission of Millennium Space Systems, El Segundo, CA, USA.
• IceCube, a NASA/GSFC 3U CubeSat technology demonstration mission.
• HARP (HyperAngular Rainbow Polarimeter), a 3U CubeSat of UMBC (University of Mayland, Baltimore County)
• CSUNSat-1, a 2U CSUN (CubeSat of California State University Northridge).
• CXBN-2 (Cosmic X-Ray Background-2), a 2U CubeSat of Morehead State University, Morehead, Kentucky.
• OPEN (Open Prototype for Educational NanoSats), a 1U CubeSat of UND (University of North Dakota).
• Violet, a 1U CubeSat of Cornell University, Ithaca, N.Y.
• Biarri-Point, a 3U CubeSat technology mission, a four nation defence related project involving Australia, the US, the UK and Canada. Biaari is an RF signal collection mission that can be related to the spot beam mapping mission through mutual use of GPS signals. 8) 9)
• QB50 x 28. Twentyeight CubeSats of the international QB50 constellation, a European FP7 (7th Framework Program) Project for Facilitating Access to Space and managed by the Von Karman Institute for Fluid Dynamics in Brussels, were flown to the ISS for subsequent deployment (atmospheric research).The 28 CubeSats of the QB50 constellation were integrated into 11 NanoRacks 6U deployers. 10) 11)
In addition, four Lemur-2 satellites, operated by Spire Global Inc. of San Francisco, were launched aboard the Cygnus OA-7 cargo craft to replenish and expand the company's constellation dedicated to obtaining global atmospheric measurements for operational meteorology and tracking ship traffic across the planet for various commercial applications. The four Lemur-2 CubeSats are mounted externally to the cargo ship. After Cygnus departs the station in July, it will climb to a higher altitude, around 500 km, and eject them into space.
Mission Status
• On May 16-17, 2017, NanoRacks began the first of two airlock cycles for the 11th and 12th NanoRacks CubeSat Deployer Missions (NRCSD-11, NRCSD-12). NRCSD-11 and NRCSD-12 were brought to the International Space Station on the Orbital ATK-7 mission, which launched on April 18, 2017 from the Kennedy Space Center in Cape Canaveral, Florida. This launch was the largest CubeSat mission of NanoRacks to date, bringing 34 satellites into the Space Station, plus four CubeSats mounted on externally on the Cygnus spacecraft. 12)
A total of 17 CubeSats were deployed on NRCSD-11 by the NanoRacks Team:
- 11 CubeSats of the QB50 mission were deployed in this first airlock cycle:
SOMP2 – TU Dresden, Germany
HAVELSAT – Havelsan, Turkey
Columbia – University of Michigan, USA
PHOENIX – National Cheng Kung University, Taiwan
X-CubeSat – Ècole Polytechnique, France
QBEE – Open Cosmos Ltd. & University of Lulea, Sweden
ZA-AEROSAT – Stellenbosch University, South Africa
LINK – Korea Advanced Institute of Science and Technology, South Korea
UPSat – University of Patras and Libre Space Foundation, Greece
SpaceCube – Ècole des Mines Paristech, France
Hoopoe – Herzliya Science Center, Israel
- 3 CubeSats were deployed of the NASA ELaNa XVII Sponsored CubeSats:
CXBN-2 of Morehead State University, Morehead, KY
IceCube of NASA/GSFC
CSUNSat-1 of California State University Northridge, NASA/JPL
- Additionally, 3 CubeSats were deployed:
Altair-1 of Millennium Space Systems
SHARC of AFRL (Air Force Research Laboratory)
SG-Sat (Stellar Gyroscope Satellite) of the University of Kentucky.
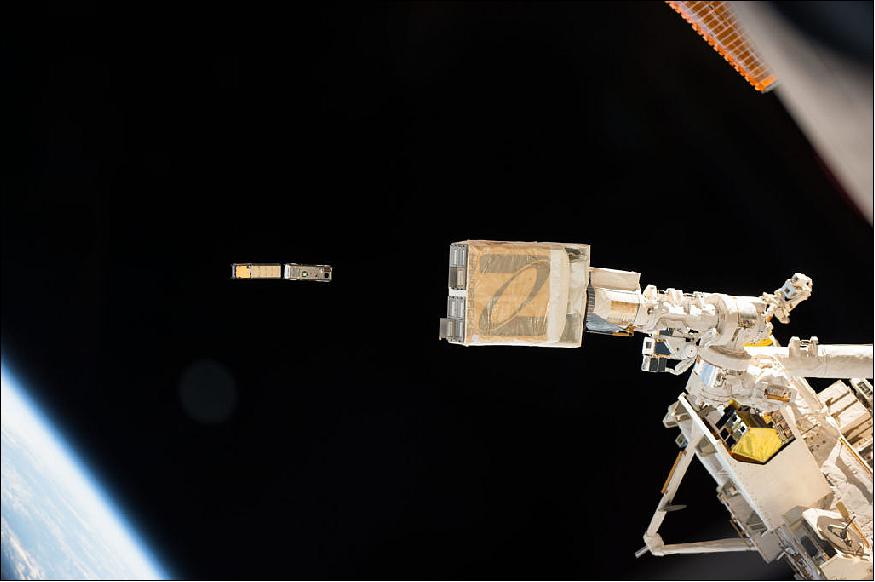
Sensor Complement
CZT (Cadmium Zinc Telluride) Array
The CZT array is the CXBN science instrument (or science array), optimized for response in the 30-50 keV energy range where the diffuse cosmic X-ray background peaks. "Though originally intended for the detection of X-ray and gamma-ray photons while operating at room temperature and for applications in medical physics and security imaging, we found that the CZT detectors possessed the desired energy resolution and photon efficiency over the energy range of interest for the mission." Thomas Pannuti, CXBN-2 Science Principal Investigator at MSU (Ref. 5).
The CZT detectors were developed by Redlen Technologies, a leading manufacturer of high-resolution semiconductor radiation detectors. The company has produced extremely uniform crystalline structure CZT material though the manufacturing process known as the Traveling Heater Method (THM). This allows uniformity in the semiconductor material so that charge is evenly distributed, allowing greater energy resolution and detection by bleeding off impurities.
The CZT detectors form the Redlen M1770 CZT Array, an imaging module onboard the CXBN-2 CubeSat. This module is a 256 pixel radiation detector that is configured in a 16 x 16 matrix with a 2.46 mm pixel pitch. It consists of a 2 x 2 array of 64 pixel CZT detectors with thicknesses of 5 mm and bonded to a common cathode plate.
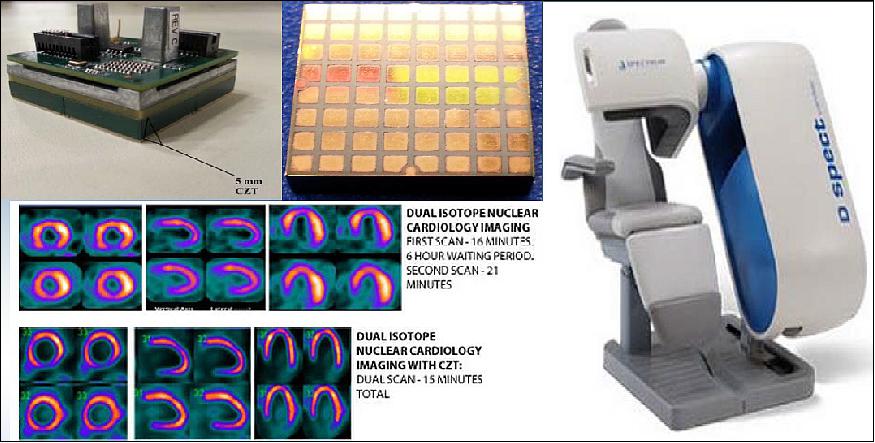
In this configuration, the CXBN-2 satellite has the potential to advance our understanding about the diffuse X-ray background in particular and the temporal evolution of supermassive black holes at the centers of galaxies in general. Malphrus and his colleagues are convinced that their CubeSat will provide measurements of the CXB with high precision, thus constraining models that address the relative contribution of the proposed dominant emitting source population (namely heavily absorbed active galactic nuclei).
Ben Malphrus and his colleagues are convinced that their CubeSat will provide measurements of the CXB with high precision, thus constraining models that address the relative contribution of the proposed dominant emitting source population (namely heavily absorbed active galactic nuclei). "Such a high precision measurement of the CXB will provide insight into the underlying physics of the early universe and provide a window on the most energetic objects in the distant universe," Malphrus explained.
"We are entering a new era of significant science being supported by CubeSats and Morehead State is at the forefront of this enterprise. The opportunity to participate in astrophysics research facilitated by the CubeSat platform as well as to train our students in space systems engineering and observational astrophysics through live space missions like CXBN-2 is invaluable to our research program, our academic programs and to our students," Thomas Pannuti concluded.
References1) K. Z. Brown, B. K. Malphrus, G. Jernigan, "The Cosmic X-Ray Background NanoSat-2 (CXBN-2): An Improved Measurement of the Cosmic X-Ray Background," Proceedings of the 2nd IAA Conference on University Satellite Missions and CubeSat Workshop, IAA Book Series , Vol. 2, No 2, Editors: Filippo Graziani, Chantal Cappelletti, Rome, Italy, Feb. 3-9, 2013, paper: IAA-CU-13-04-01
2) Jordan Healea, Ben Malphrus, Sean Mckneil, Kevin Brown, Jeff Kruth, Bob Twiggs, Kien Dang, Andrew Cavins, Yevgenih Byleborodov, "The Cosmic X-ray Background NanoSat-2 (CXBN-2): An improved measurement of the diffuse X-ray background," 12th Annual CubeSat Developers Workshop, Cal Poly, San Luis Obispo, CA, USA, April 22-24, 2015, URL: https://web.archive.org/web/20171115162009/http://mstl.atl.calpoly.edu:80/~bklofas/Presentations/DevelopersWorkshop2015/Healea_CXBN-2.pdf
3) M. Ovchinnikov, V. Penkov, B. Malphrus, K. Brown, D. Roldugin, "Active magnetic attitude control algorithms for a CubeSat for astrophysics research," Moscow, Russia, 2014, URL: http://keldysh.ru/papers/2014/prep2014_47_eng.pdf
4) Jacob R. Wade, Eric Thomas, " Retooling Space Solar Cell System CIC for CXBN-2; Solar Cell CIC Optimization and Factorization for CXBN-2," Proceedings of the 30th Annual AIAA/USU SmallSat Conference, Logan UT, USA, August 6-11, 2016, URL: http://digitalcommons.usu.edu/cgi/viewcontent.cgi?article=3461&context=smallsat
5) Tomasz Nowakowski, "CXBN-2 CubeSat to embark on an important X-ray astronomy mission," Phys Org, March 6, 2017, URL: https://phys.org/news/2017-03-cxbn-cubesat-embark-important-x-ray.html
6) "NASA Space Station Cargo Launches aboard Orbital ATK Resupply Mission," NASA, Release 17-029, April 18, 2017, URL: https://www.nasa.gov/press-release/nasa-space-station-cargo-
launches-aboard-orbital-atk-resupply-mission
7) "United States Commercial ELV Launch Manifest," Dec. 28, 2016, URL: http://www.sworld.com.au/steven/space/uscom-man.txt
8) Eamonn P. Glennon, Joseph P. Gauthier, Mazher Choudhury, Kevin Parkinson, Andrew G. Dempster, "Project Biarri and the Namuru V3.2 Spaceborne GPS Receiver," IGNSS (International Global Navigation Satellite Systems Society) Symposium 2013, Outrigger Gold Coast, Australia, 16 – 18 July 2013, URL: https://pdfs.semanticscholar.org/3d15/3f47ef0f39f21acb3649ab92afef72b53aea.pdf
9) Jacob A. LaSarge, "A CubeSat mission for mapping spot beams of geostationary communication satellites," Thesis, March 2015, URL: http://www.dtic.mil/get-tr-doc/pdf?AD=ADA617698
10) Davide Masutti, "QB50-ISS CubeSats ready to be launched," Dec. 9, 2016, URL: https://www.qb50.eu/index.php/news/78-qb50-iss-ready-to-be-launched
11) US Commercial ELV Launch Manifest, March 5, 2017, URL: http://www.sworld.com.au/steven/space/uscom-man.txt
12) "NanoRacks CubeSat Deployer Mission 11 Status Update: Good Deploy!," NanoRacks, May 17, 2017, URL: http://nanoracks.com/cubesat-deployer-mission-11-update/
The information compiled and edited in this article was provided by Herbert J. Kramer from his documentation of: "Observation of the Earth and Its Environment: Survey of Missions and Sensors" (Springer Verlag) as well as many other sources after the publication of the 4th edition in 2002. Comments and corrections to this article are always welcome for further updates (eoportal@symbios.space).
Background Spacecraft Launch Mission Status Sensor Complement References Back to Top