KPLO (Korean Pathfinder Lunar Orbiter)
Non-EO
KARI
Exploration
Quick facts
Overview
Mission type | Non-EO |
Agency | KARI |
Launch date | 04 Aug 2022 |
KPLO (Korean Pathfinder Lunar Orbiter) Mission
Spacecraft Launch Sensor Complement Ground Segment References
KPLO will be the first Korean satellite to fly beyond Earth’s orbit and is expected to be launched in the middle of 2022. The KPLO program started in 2016 and is led by the Korea Aerospace Research Institute (KARI). KARI launched its first multipurpose satellite, called Korea Multi-Purpose Satellite (KOMPSAT)-1, in 1999, and now operates more than 15 Earth-orbiting KOMPSAT series, including low Earth orbit (LEO) and geostationary orbit (GEO) satellites. Starting from the KPLO, Korea’s space exploration activities are expected to be more accelerated, as Korea plans to focus on lunar surface investigations using landers and rovers by the end of 2030, and landing on asteroids is seriously considered by the end of 2035. Currently, Korea has just begun to consider another new deep space program, visiting the Apophis during its close approach to Earth in 2029. 1)
The mission objectives of the KPLO have been set up as follows. The first is to secure critical technologies for lunar explorations, the second is to investigate the environment, which includes the establishment of a lunar topographic map to support future lunar landing sites selection, a lunar resources survey, and an investigation on the radiation environment, as well as the surface environment of the Moon. The final goal is to demonstrate and validate space internet technology. To accomplish the above objectives, the KPLO will carry six payloads to the Moon.
Spacecraft
The overall launch mass of the KPLO spscecraft is expected to be approximately 678 kg, including the six payloads. The original transfer method to reach the Moon was to use a 3.5 phasing loop orbit around the Earth; however, the WSB/BLT (Weak Stability Boundary/Ballistic Lunar Transfer ) method was recently selected to save ΔV, which ultimately secure more fuel for KPLO. Actually, it is well known that an average of about 160 m/s of ΔV savings can be achieved by selecting the WSB/BLT method compared to the conventional transfer method to the Moon, and the KPLO indeed saved about 165 m/s of ΔV.
With the WSB/BLT method, the current baseline launch period for the KPLO is approximately 40 days (from late July to early September 2022). After several months of transfer, the KPLO will arrive at the Moon in mid-December 2022 and will begin the Lunar Orbit Acquisition (LOA) phase to be inserted into the Moon. During the LOA phase, which is expected to take approximately 15 days, the KPLO will conduct a total of five Lunar Orbit Insertion (LOI) burns to achieve its final mission orbit. Immediately after the LOA phase, the commissioning phase will be initiated for an approximately one-month period. During the commissioning phase, not only the KPLO bus system, but also all of the onboard instruments will be calibrated and validated to conduct the upcoming one-year nominal mission around the Moon.
To successfully deliver KPLO to the Moon, the spacecraft is designed to have a four Orbit Maneuver Thruster (OMT), with approximately 31.8 N of thrust with 227 s of Isp (specific impulse) for large burn execution, and eight Attitude Control Thruster (ACT) with approximately 3.48 N with 218 s of Isp for small burn execution. All of these engines will be clustered for burn executions. Four OMT engines will be clustered to be used for the main thruster set for large burns during the transfer and LOA phase, i.e., LOIs as well as large-sized Trajectory Correction Maneuvers (TCMs) that require more than 10 m/s of ΔVs. For small burns, such as for TCMs less than 10 m/s, momentum dumping, and orbit maintenance during the nominal mission phase, eight individual ACT engines will be clustered into sets of two ACT and will be used. These two ACT sets will also be used for liquid setting purposes as well as attitude maintenance before and during large burns.
The stowed and in-flight configuration of the KPLO spacecraft is shown in Figure 1, and the WSB/BLT transfer trajectory during the launch period and the orbit of the LOA phase are shown in Figure 2. On the left side of Figure 2, the WSB/BLT transfer trajectory with respect to the Earth–Sun rotating frame is depicted, and the LOA phase in the Moon Inertial frame is shown on the right side of Figure 2.
Spacecraft and Subsystems
The spacecraft has a cubic shape with two solar panel wings and a parabolic antenna mounted on a boom. The total mass is 550 kg. Communications are via S-band (telemetry and command) and X-band (payload data downlink). Power (760 W at 28 V) is provided through the solar panel arrays and rechargeable batteries. A monopropulsion system is used, with four 30 N orbital maneuver thrusters and four 5 N attitude control thrusters. KPLO is equipped with five science instruments and a Disruption Tolerant Network experiment.
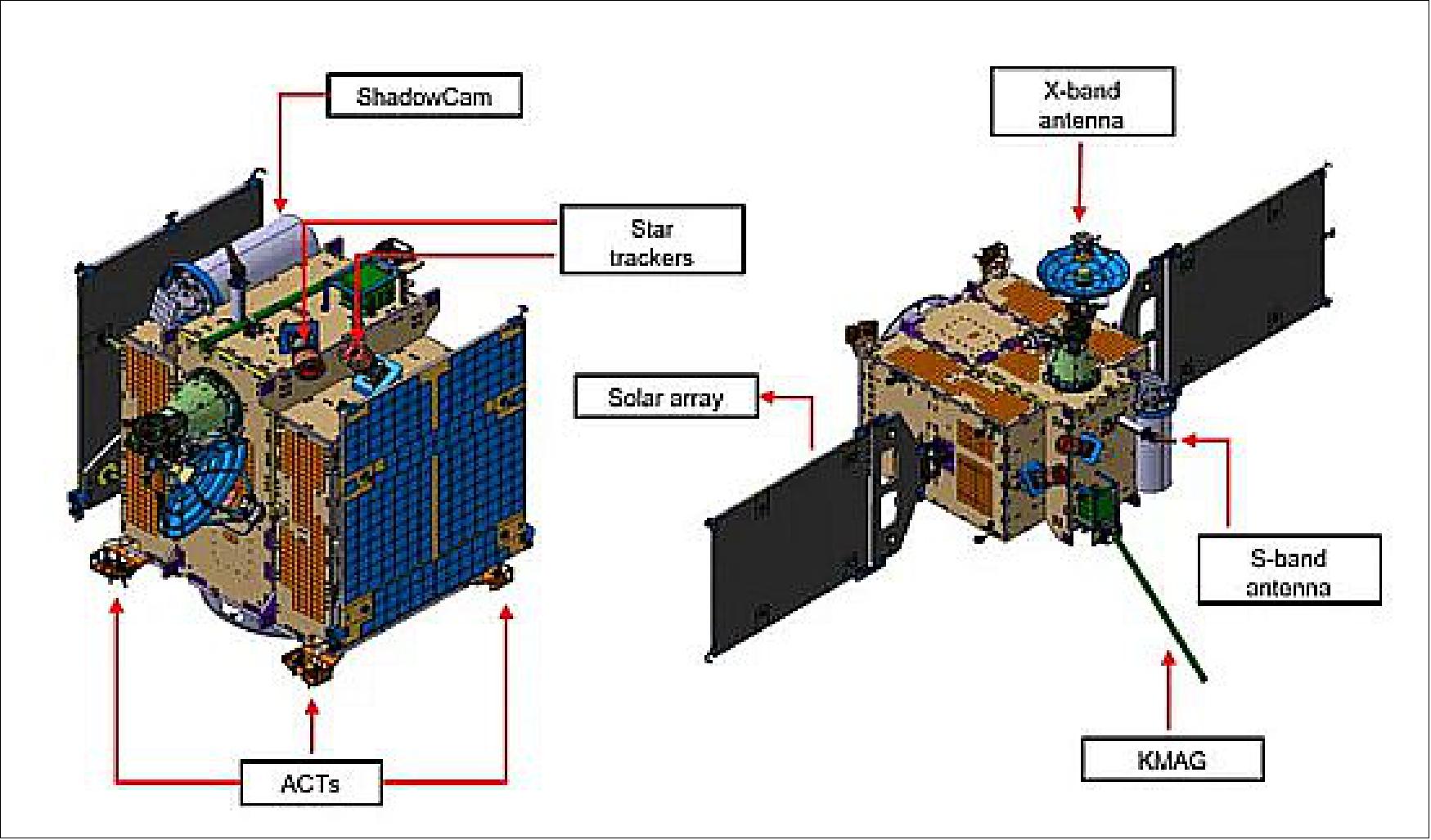
Launch
A launch of KPLO occured on August 4, 2022 on a SpaceX Falcon 9 Block 5 vehicle from Cape Canaveral, Florida into a 300 km Earth orbit, followed by a translunar injection burn and a one month lunar transfer phase. With the use of the Weak Stability Boundary (WSB)/Ballistic Lunar Transfer (BLT) method, the KPLO will be delivered to the final mission orbit around the Moon that has a 90 º inclination with respect to the lunar equator. After capture into an elliptical lunar orbit, it will circularize to a 100 km nominal polar orbit (±30 km), from which it will conduct science operations for approximately one year. If the mission has an extended phase, it will descend to a 70 km orbit or lower.
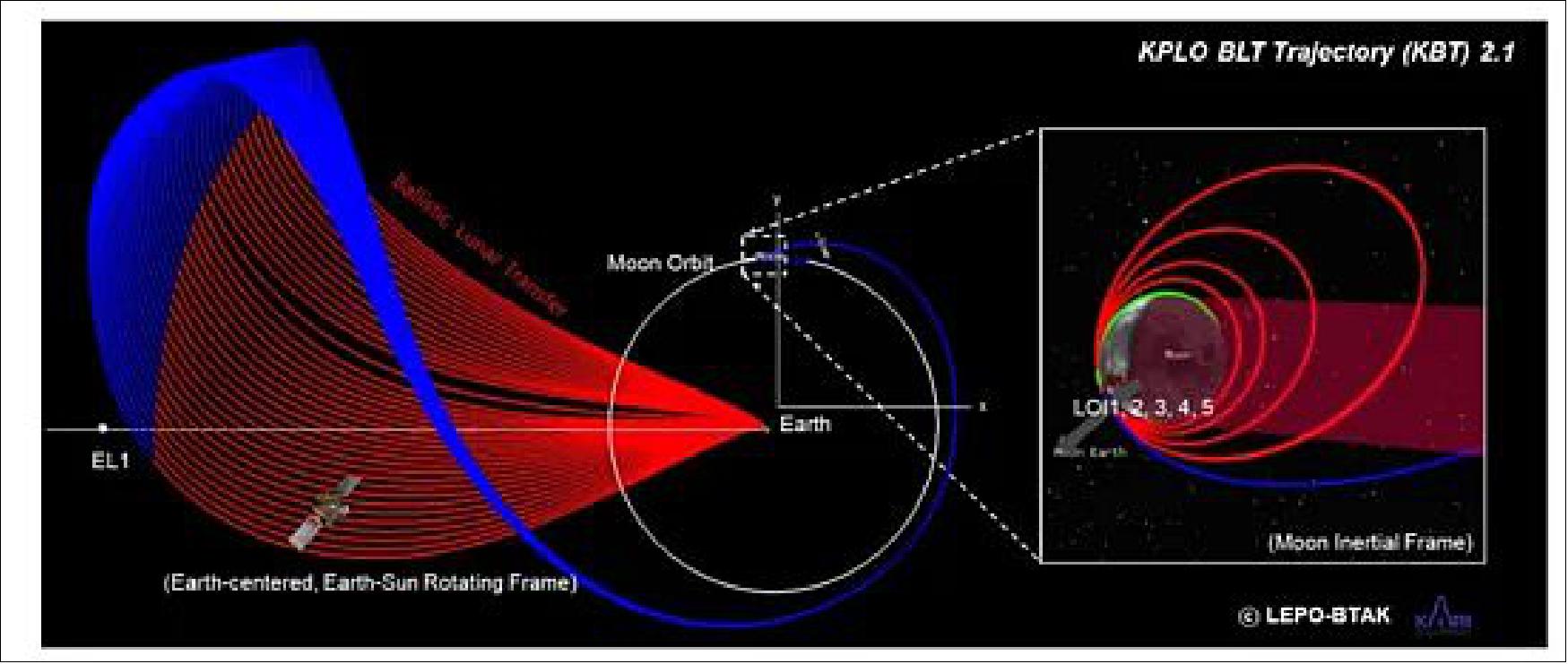
The Lunar exploration project is to develop the first lunar probe in Korea and secure the necessary basic technology for lunar exploration, such as an orbiter, a landing module, science payload, and deep space communication. 2)
The development of the Pathfinder lunar orbiter will be carried out based on international collaboration, securing core technology for space exploration, establishing a deep space telecommunication network, and developing technology payload in 1st phase.
In the following second phase led by Korea, the unmanned lunar orbiter and lunar lander will be developed and launched as a Korean launch vehicle.
The Pathfinder lunar orbiter is equipped with five payloads developed in Korea and one payload to be developed by NASA in the United States.
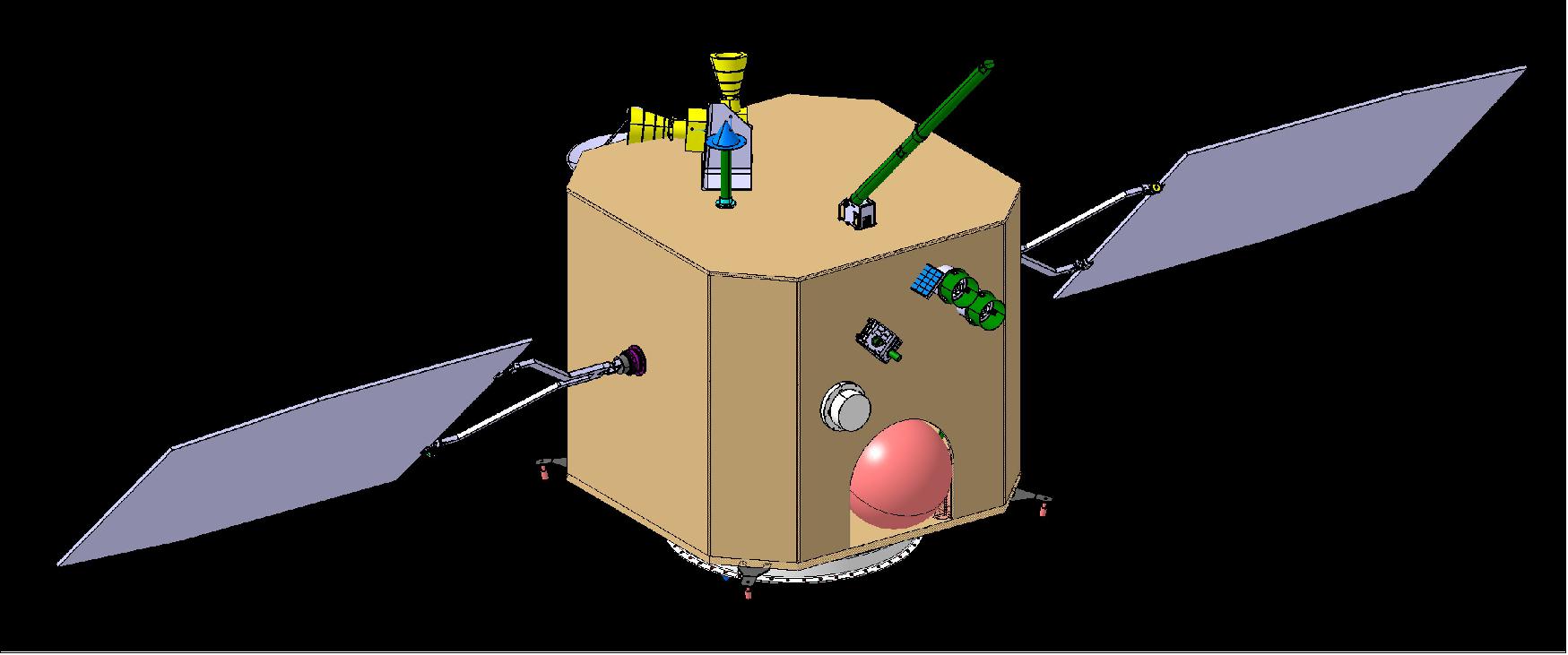
NASA has selected nine scientists to join the upcoming Korea Pathfinder Lunar Orbiter (KPLO) mission. Set to launch in August 2022 on a SpaceX Falcon 9 and orbit the Moon for about a year, KPLO is the first space exploration mission of the Republic of Korea (ROK) that will travel beyond Earth orbit. 3)
“The KPLO Participating Scientist Program is an example of how international collaborations can leverage the talents of two space agencies, to achieve greater science and exploration success than individual missions,” says Dr. Sang-Ryool Lee, the KPLO Project Manager, “It’s fantastic that the Korea Aerospace Research Institute (KARI) lunar mission has NASA as a partner in space exploration—we’re excited to see the new knowledge and opportunities that will arise from the KPLO mission as well as from future joint KARI–NASA activities.”
The three overarching goals of the KPLO mission are realizing the first space exploration mission by ROK, developing and verifying space technologies suitable for deep-space exploration on future missions, and investigating the physical characteristics of the lunar surface to aid future robotic landing missions to the Moon.
To meet these objectives, the spacecraft will carry a payload of five scientific instruments to include three cameras, a magnetometer, and a gamma-ray spectrometer. NASA is contributing one of the cameras, known as ShadowCam, which will be used to obtain optical images at high-resolution of the permanently shadowed regions at the lunar poles of the Moon that are thought to contain ice. ShadowCam’s principal investigator is Mark Robinson, Professor of Geological Sciences at Arizona State University’s School of Earth and Space Exploration.
“It is important that the participating scientists are fully embedded in the existing KARI and NASA teams well before the mission is due to launch,” said Shoshana Weider from NASA’s Planetary Science Division who leads the KPLO Participating Scientist Program. “This means they will have plenty of time to collaborate with their KARI colleagues during the pre-launch mission-planning phase, which will help ensure that the science return of their projects, and the mission as a whole, is maximized.”
Each of the nine participating scientists will join the KPLO science team for at least one of the five KPLO instruments beginning later this year and will be funded for three years. The selected scientists and the associated KPLO instrument(s) are:
• William Farrand, Space Science Institute, Boulder, CO: Using KPLO multispectral polarimetric data to distinguish and characterize known and suspected lunar pyroclastic deposits (PolCam)
• Caleb Fassett, NASA’s Marshall Space Flight Center, Huntsville, AL: Evolution of lunar topography and regolith as constrained by polarimetry (PolCam)
• Ian Garrick-Bethell, University of California, Santa Cruz: The lithospheric magnetic field of the Moon at 100 km (KMAG)
• Rachel Klima, Johns Hopkins University Applied Physics Laboratory, Laurel, MD: Lunar mineralogical prospecting through integrated spectral analysis (KGRS/LUTI)
• Mikhail Kreslavsky, University of California, Santa Cruz: Study of lunar polarimetric anomalies with PolCam
• Shuai Li, University of Hawaii, Honolulu: Surface reflectance quantification to enhance performance of predictive modeling of ice in lunar regolith (ShadowCam)
• Gorden Videen, Space Science Institute, Boulder, CO: Polarized light scattering from lunar regolith simulants in support of PolCam
• Jean-Pierre Williams, University of California, Los Angeles: Local and seasonal temperatures of KPLO polar targets (ShadowCam)
• Naoyuki Yamashita, Planetary Science Institute, Tucson, AZ: Lunar geochemistry and prospecting with the KPLO Gamma-Ray Spectrometer (KGRS)
The newly appointed scientists were selected through the competed KPLO Participating Scientist Program, which is run jointly by NASA’s Science Mission Directorate (SMD) Planetary Science Division (PSD) and Human Exploration and Operations Directorate (HEOMD) Advanced Exploration Systems Division (AES). The addition of the scientists to KPLO’s existing science team will serve to expand the scientific return of the KPLO mission.
The Moon will be the focus of many robotic and human exploration missions in the coming years, including those under the agency’s Artemis program. Beginning later this year, NASA will send science instruments and technology experiments on two separate American robotic landers to the lunar surface. Follow-on commercial deliveries for NASA are slated about twice per year, including flights to the lunar South Pole. The KPLO lunar mission will provide scientific data to better understand the lunar poles and assist planning for some Artemis activities.
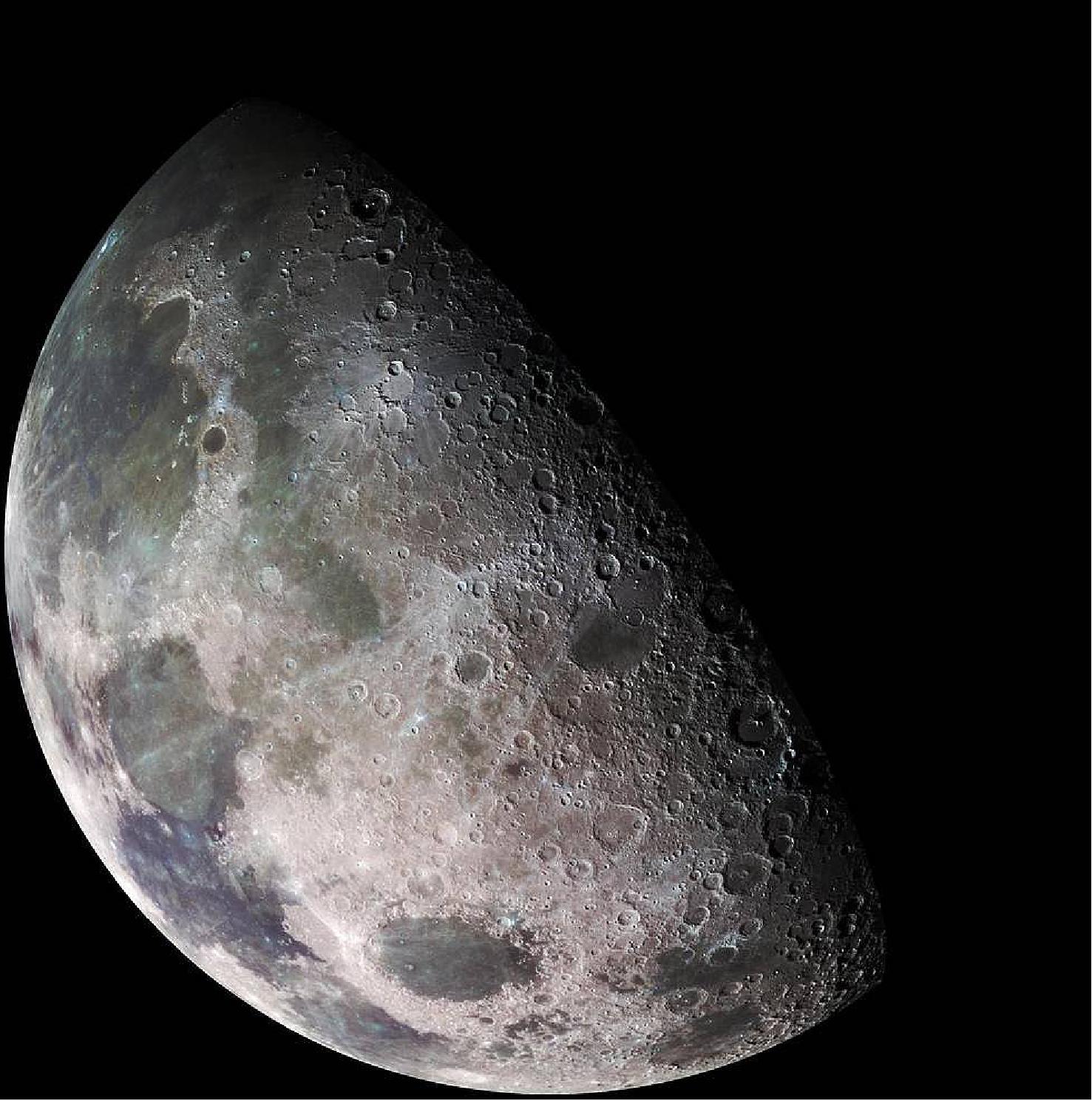
KPLO is South Korea's first lunar mission. It is developed and managed by the Korea Aerospace Research Institute (KARI) and is scheduled to launch in July 2022 to orbit the Moon for 1 year carrying an array of South Korean experiments and one U.S. built instrument. The objectives are to develop indigenous lunar exploration technologies, demonstrate a "space internet", and conduct scientific investigations of the lunar environment, topography, and resources, as well as identify potential landing sites for future missions. 4)
Sensor Compliment
The six experiments are a Lunar Terrain Imager (LUTI), a Wide-Angle Polarimetric Camera (PolCam), a Magnetometer (KMAG), a Gamma-Ray Spectrometer (KGRS), DTNPL (Disruption Tolerant Network Experiment Payload) and a high-sensitivity camera developed and funded by NASA (ShadowCam). The total scientific payload mass is about 40 kg.
KPLO spacecraft nominal mission orbit will be nadir pointing with lunar polar orbit. KPLO spacecraft has several mission phases which are pre-launch, launch, early cruise, phasing-loop transfer, late cruise, lunar orbit acquisition, commissioning, nominal mission, extended mission, end of mission. Operational circular orbit with an altitude of 100 km will be achieved by several consecutive lunar orbit insertions (LOI). The nominal mission orbit will be selected for the commissioning orbit. At the end of commissioning, science mission will be performed at the nominal mission orbit. The moon-centered view is as below. Also, the spacecraft has sun-safe mode,
LUTI (Lunar Terrain Imager)
LUTI is the primary payload on KPLO it will provide high resolution images of the lunar surface. 5) LUTI will take images of the lunar surface with a high spatial resolution (less than 5 m) to investigate a candidate landing site to further prepare for Korea’s second-stage lunar explorations. 6)
System overview
- The LUTI instrument is light-weighted and features a high dynamic range electro-optical camera system.
- The pushbroom camera features linear CCD sensors
- LUTI provides digitized and compressed imagery.
System configuration
- Two identical independent cameras
- CCD detectors with optical filters
- Camera electronics
- Power module.
LUTI Operational Modes
LUTI will provide high-resolution images with four operational modes.
- Survival mode — when the S/C bus is in abnormal conditions, or from the moment of the launch vehicle separation until the moment of normal operation initiation in lunar orbit.
- Standby mode — when LUTI is in the operational temperature range and camera electronics is in off state
- Ready mode — when LUTI is in preparation of imaging operations
- Imaging mode — LUTI takes images in pushbroom mode and transmits the data to a mass memory
- Diagnostic mode — LUTI transmits predefined pattern images to the mass memory.
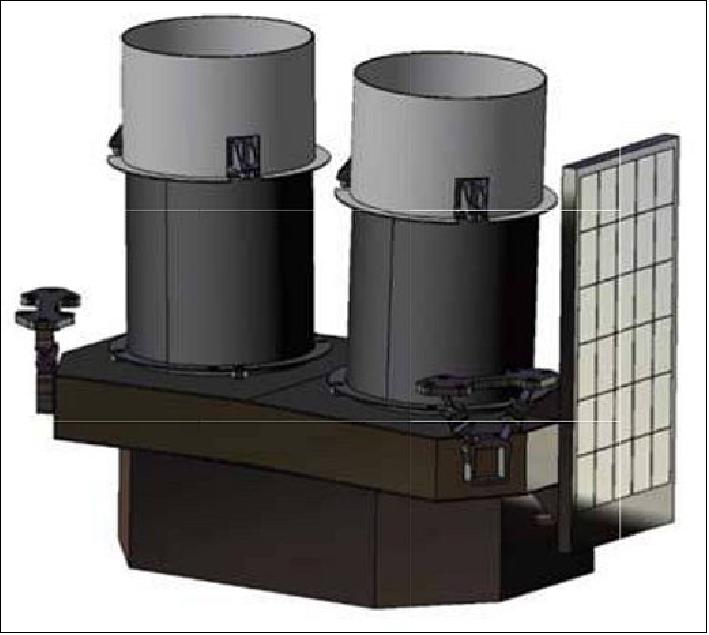
LUTI data will be processed by the ICAS (Image Calibration and Analysis Subsystem) in KDGS (KPLO Deep-space Ground System), and the SDMS (Science Data Management Subsystem) will handle other science payload data and preprocess them to deliver payloads and developers for further scientific research. The science data products will comply with the PDS4 standard established by NASA.
PolCam (Wide-Angle Polarimetric Camera)
PolCam is being developed by KASI (Korea Astronomy and SpaceScience Institute) for KPLO. The objectives are to understand the space weathering process, to investigate the internal evolution of the moon and to support the lunar landing search. To investigate the characteristics of the lunar regolith, the PolCam will take polarimetric images of the entire lunar surface. 7)
PolCam will construct a global lunar map of polarimetric parameters, such as maximal polarization (Pmax) and titanium distribution using three color bands centered at 320, 430, and 750 nm. PolCam's twin cameras are mounted at 45º tilt angles from the nadir across the orbital track in opposite directions. PolCam will obtain polarimetric measurements of sunlight scattered by the lunar surface at various phase angles up to ~140º to achieve the scientific goals during the one-year mission.
Since the degree of linear polarization is a function of the phase angle, it is essential to perform several measurements at various phase angles to properly retrieve, e.g., Pmax, Pmin, and the inversion angle, αinv.
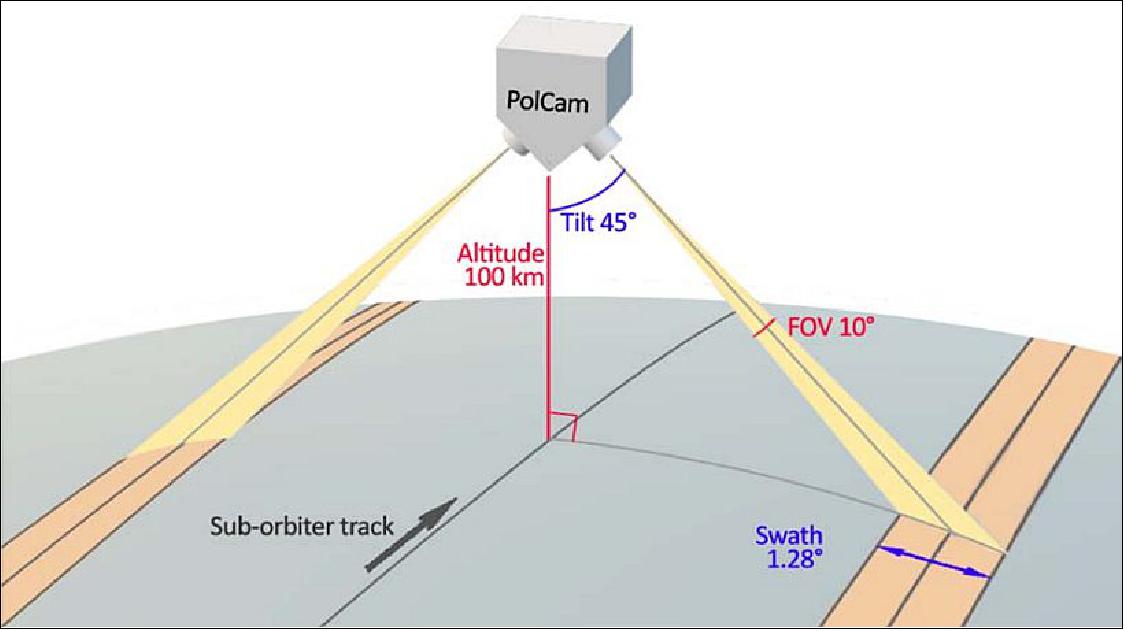
The KPLO/PolCam will measure the polarimetric properties of the surface of the Moon from lunar orbit for the time. The two identical cameras of PolCam have a 10° FOV and view opposite directions across the track at a tilt angle of 45° from the nadir direction. The observational data from the tilted cameras will provide the degree of linear polarization at phase angles up to 140°, whereas a nadir-viewing camera with the same FOV can cover phase angles only up to 95°. With a tilt angle of 45°, PolCam can collect a number of measurements at several different phase angles, enabling estimation of the polarization maximum for the lunar surface at latitudes up to ±70° during the nominal one-year mission duration. When the tilt angle is decreased to 25°, there is a large observational gap at high phase angles. A semi-annual yaw-flip only interchanges the observing sites of the two cameras of PolCam, maintaining the total coverage across longitude, latitude, and phase angle.
On the basis of polarimetric and photometric methods, PolCam will construct a global lunar map of maximum polarization and other polarimetric parameters, regolith particle size, and titanium distribution using three colored bands and five polarization filters, increasing knowledge of the scattering properties of the lunar surface. The observational data will also be helpful in the landing-site selection for a future mission to the Moon.
KMAG (KPLO Magnetometer)
Mission objective: Localized magnetic fields (magnetic anomaly) of the lunar surface have been examined by previous lunar exploration missions such as Lunar Prospector (LP) and KAGUYA (SELENE). Even though past lunar missions have derived reasonable results for the lunar magnetism, many puzzles still remain. Magnetic field maps have already been obtained but more data are required to understand the origins of lunar magnetism and swirl formation. — The main objective of the KMAG instrument is to provide measurement data of the magnetic field of the Moon using three fluxgate sensors. 8)
KMAG consists of the Magnetometer (MAG) unit and the Fluxgate magnetometer Control Electronics (FCE) unit (Figure 7). The MAG unit is composed of the boom Assembly, actuator Assembly and hinge Assembly. The three MAGs are located inside a 1.2 m-long tube of the Boom Assembly and the tube is made from CFRP (Carbon Fiber Reinforced Plastic), which has high strength to weight ratio. The FCE is composed of a housing structure and four electrical boards: Low Voltage Power Supply (LVPS), On-Board Computer (OBC), Digital board and Analog board. The OBC communicates with Spacecraft BUS Management Unit (SBMU). The housekeeping and science data will be generated at about 295.31 Mbit/day. KMAG has a mass of 3.5 kg and power consumption of 4.6 W.
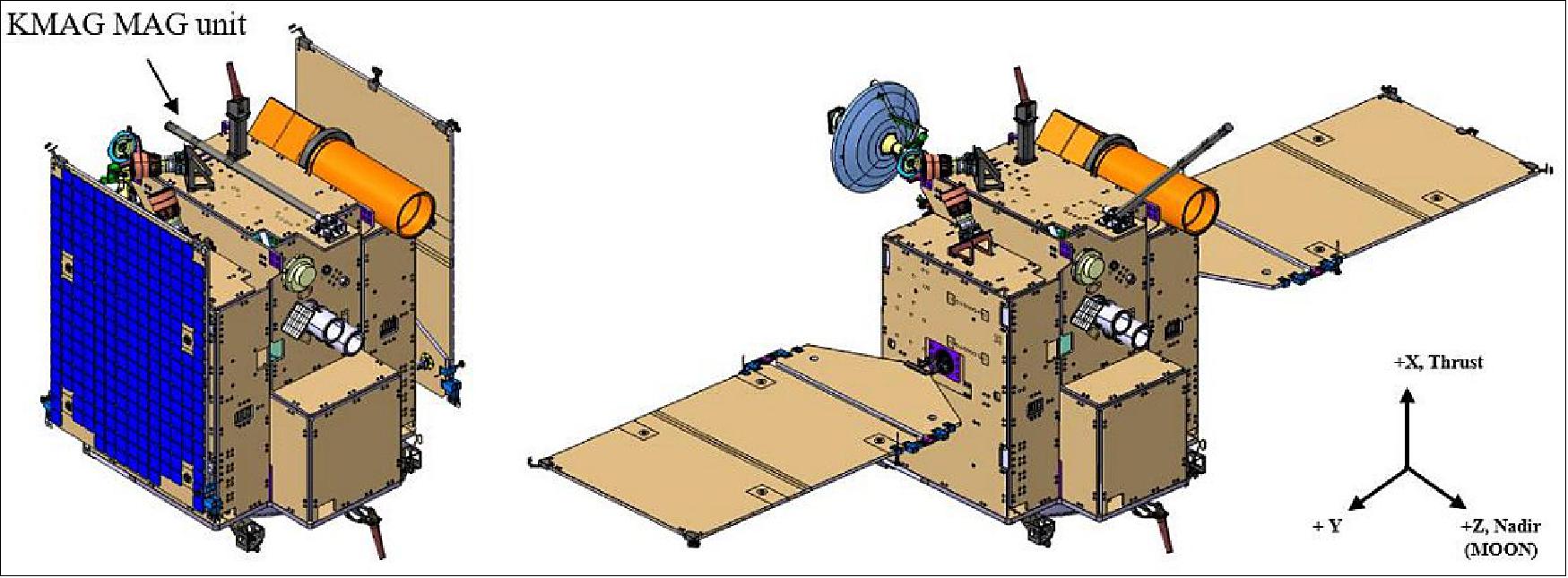
Magnetometer: The magnetometer of the KMAG is a tri-axial fluxgate designed to measure ±1000 nT range of DC magnetic fields with lower than 0.2 nT resolution. The magnetometer has a temperature coefficient of less than 0.1%/°C and noise of less than 50 pT/√Hz. The dimensions of fluxgate magnetometer are ∅45 x 45 mm and it has a 3-axis configuration. The mass is 0.15 kg and power consumption is about 0.28 W. The MAG unit is designed to deploy with a 135° angle on the central top floor of spacecraft (S/C) to minimize an effect of magnetic disturbance from the S/C. For this deployment mechanism, a non-explosive actuator is equipped on the MAG unit with torsion springs. The three fluxgate magnetometers are installed between the edge and middle of the boom to eliminate the S/C's induced fields effectively. In addition, a three-axis magnetoresistive (MR) sensor is located inside the FCE unit to detect the internal magnetic condition of S/C. The MR sensor will also provide useful information for in-flight calibration and data processing.
Operation Scenario: KMAG has two operation categories. Deployment mode: MAG unit will be deployed right after the S/C deployment process of solar panels and antennas. Normal operation mode: KMAG will operate on a 100 % duty cycle in nominal phase and extended phase. Measurements will continue and datawill be transferred to the Earth until KPLO impacts on the surface of the Moon.
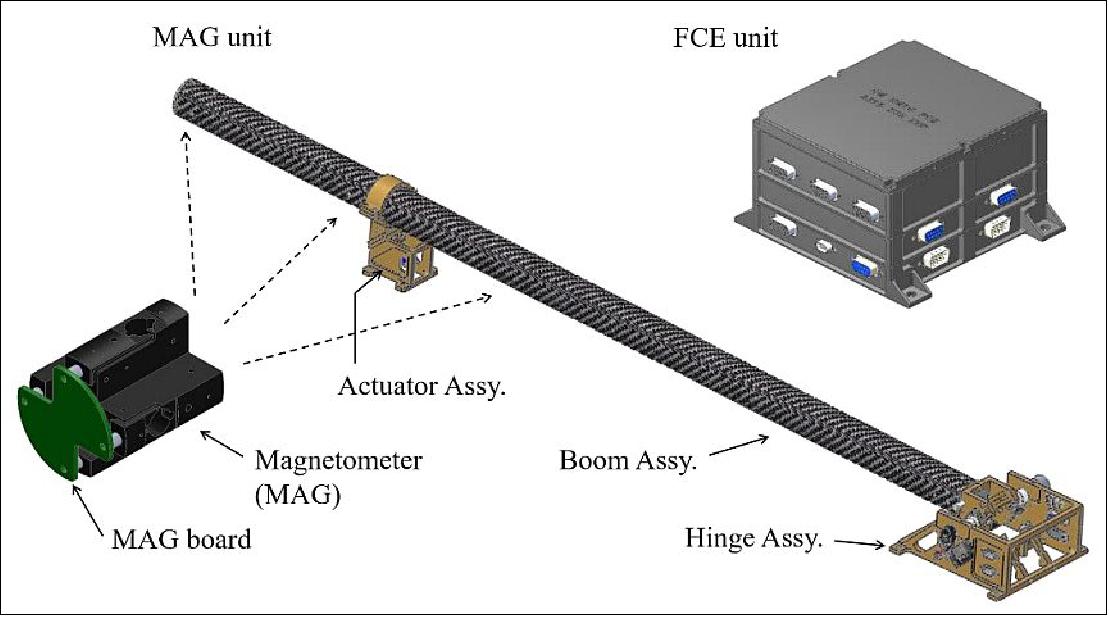
KGRS (KPLO Gamma-Ray Spectrometer)
The KGRS will investigate the chemical composition of the lunar surface materials by mapping the spatial distribution of gamma-ray energy, which ranges from 10 keV to 10 MeV. The KGRS will collect low energy gamma-ray signals by either X-ray fluorescence or by natural radioactive decay in the low energy regions of spectrum and higher-energy gamma rays due to either neutron capture or inelastic scatter. The GRS system will determine the elemental compositions of the near surface of the Moon for investigation of the lunar geology and resources. 9)
The main detector of KGRS is surrounded by an anti-coincidence counting module of a boron-loaded plastic scintillator to reduce both low energy gamma-ray background from the spacecraft and housing materials and high energy gamma-ray background from cosmic rays. The main detector has high and low gain settings for gamma-ray energy ranges up to 3 and 10 MeV, respectively.
One month of calibration and 11 months of a nominal mission period are planned with a minimum required GRS background measurement at anti-nadir direction. The planned altitude of KPLO in orbit is 100 ± 40 km, and KGRS data will be collected every 10 sec. The KGRS investigation will help to assess open questions related to the spatial distribution and origin of the elements on the lunar surface and will contribute to unraveling geological surface evolution and elemental distributions of potential lunar resources. This talk introduces the current development of KGRS and prospective scientific outcomes by KGRS data.
DTNPL (Disruption Tolerant Network Experiment Payload)
DTNPL will conduct an interplanetary internet communication experiment on disruption tolerant networking.
ShadowCam
NASA has selected an instrument developed by investigators at Arizona State University (ASU) and Malin Space Science Systems (MSSS) as a U.S. contribution to the Korea Aerospace Research Institute’s (KARI) first lunar exploration mission, Korea Pathfinder Lunar Orbiter (KPLO). ShadowCam will address Strategic Knowledge Gaps, or lack of information required to reduce risk, increase effectiveness, and improve the designs of future human and robotic missions. ShadowCam joins four KARI-developed instruments on KPLO. 10)
KARI, headquartered in Daejeon, South Korea, provided NASA with about 15 kg of payload mass aboard the KPLO. In September 2016, NASA issued a solicitation seeking science instruments that could increase our understanding of the distribution of volatiles, such as water, including the movement of such resources toward permanently shadowed regions and how they become trapped there.
“Permanently shadowed regions have been a mystery because the perpetually dark interiors are difficult to image and existing research offers varying interpretations regarding the distribution of volatiles within these cold regions,” says Jason Crusan, Director of NASA’s Advanced Exploration Systems Division at NASA Headquarters in Washington, D.C. “Future missions in deep space will be safer and more affordable if we have the capability to harvest lunar resources, and ShadowCam has the potential to greatly increase our understanding of the quality and abundance of those resources in these regions.”
ShadowCam will map the reflectance within the permanently shadowed regions to search for evidence of frost or ice deposits. The instrument’s optical camera is based on the LRO (Lunar Reconnaissance Orbiter) Narrow Angle Camera, but is 800 times more sensitive, allowing it to obtain high-resolution, high signal-to-noise imaging of the moon’s permanently shadowed regions. ShadowCam will observe the PSRs (Permanently Shadowed Regions) monthly to detect seasonal changes and measure the terrain inside the craters, including the distribution of boulders.
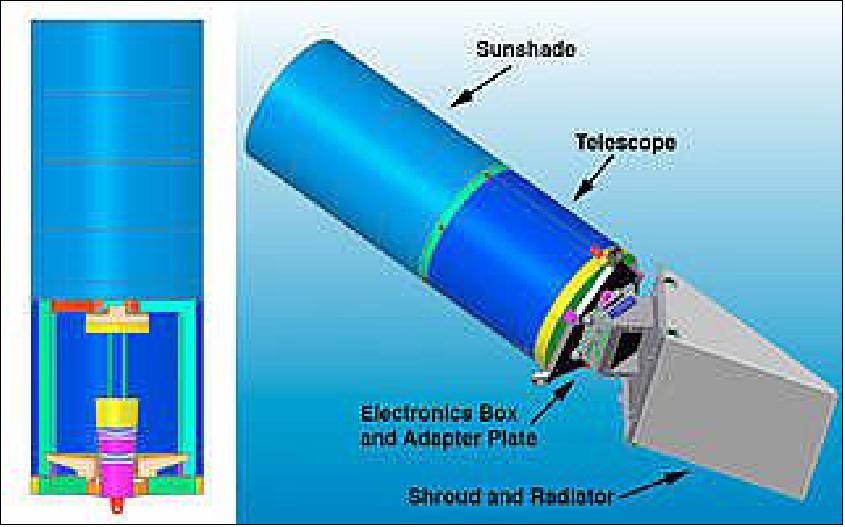
“We are pleased with the addition of NASA’s ShadowCam instrument,” says Dr. Seok Weon Choi, Director of the Lunar Exploration Program Office at KARI. “KPLO is an exciting mission and will reveal much about the moon for us and our spacefaring partners.”
NASA’s Advanced Exploration Systems Division (AES) led the payload solicitation and selection for the NASA instrument on KPLO. A division of the Human Exploration and Operations Mission Directorate, AES uses innovative approaches and public-private partnerships to rapidly develop prototype systems, advance key capabilities, and validate operational concepts for future human missions beyond Earth orbit. Through this partnership opportunity with KARI, AES is addressing key lunar SKGs while complementing KARI’s primary mission objectives and instruments.
• The ShadowCam instrument was stowed inside its shipping container on 12 August 2021, starting the first leg of a long journey that will eventually place it into lunar orbit. The camera, which is based on the successful Lunar Reconnaissance Orbiter Camera Narrow Angle Camera, left Malin Space Science Systems (MSSS) in San Diego and departed the United States for South Korea on 13 August 2021. During its time at the Korean Aerospace Research Institute (KARI), the Korean Pathfinder Lunar Orbiter (KPLO) engineering team will mount the ShadowCam instrument to the satellite, along with four other Korean-built science instruments, before shipping the completed satellite to the United States for launch from Cape Canaveral. 11)
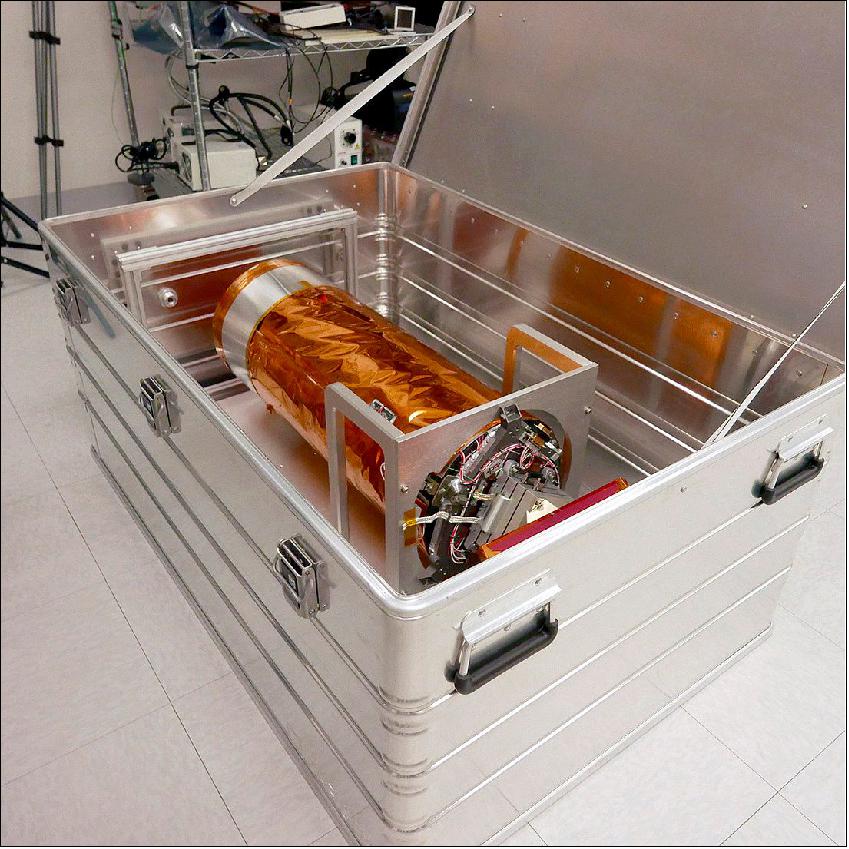
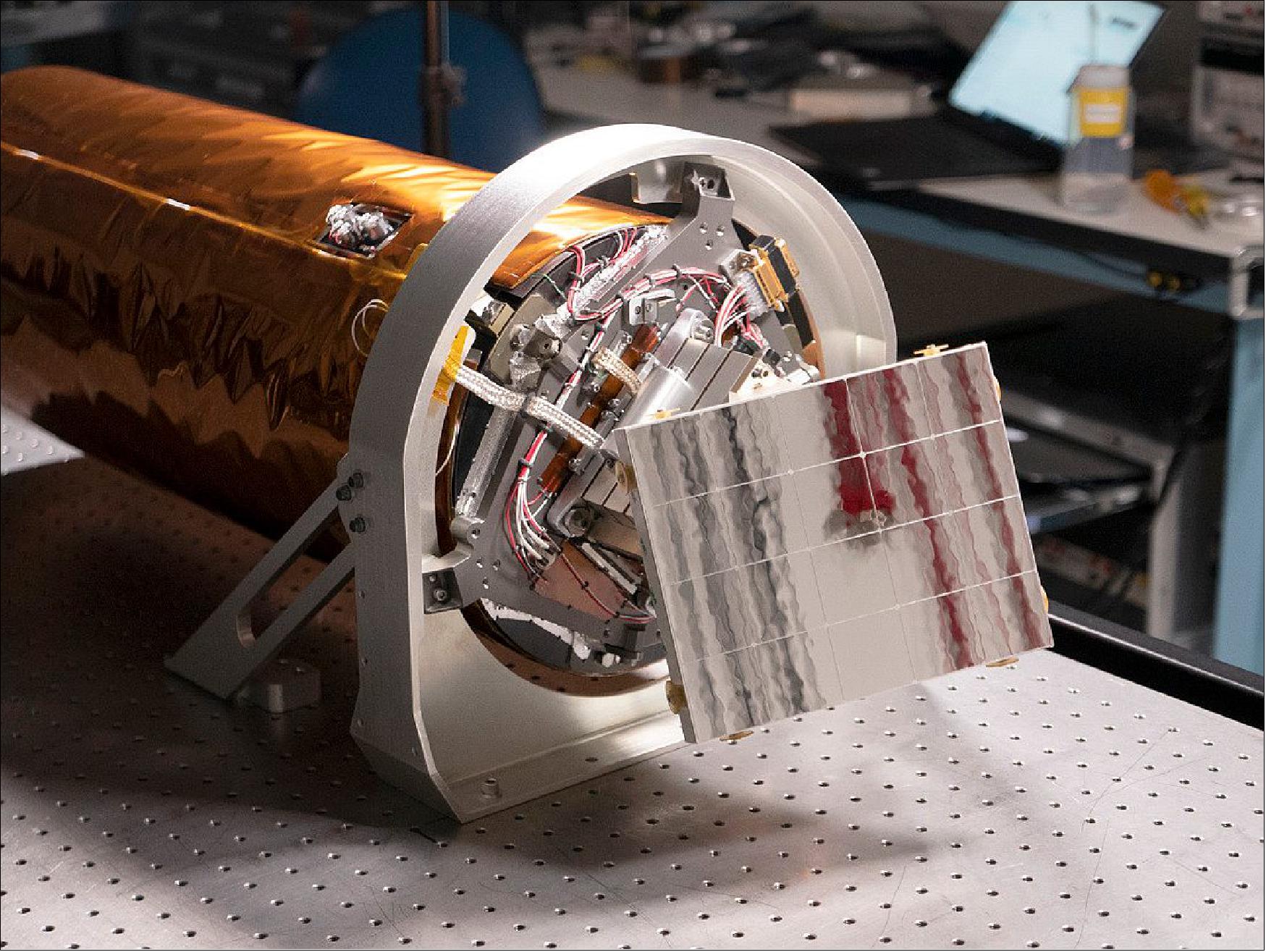
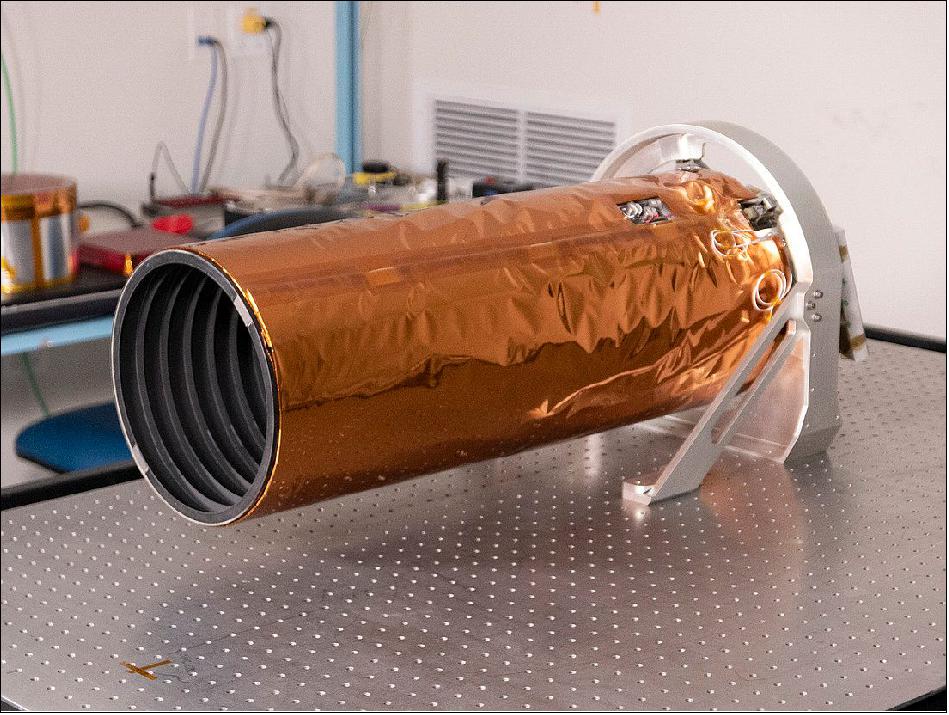
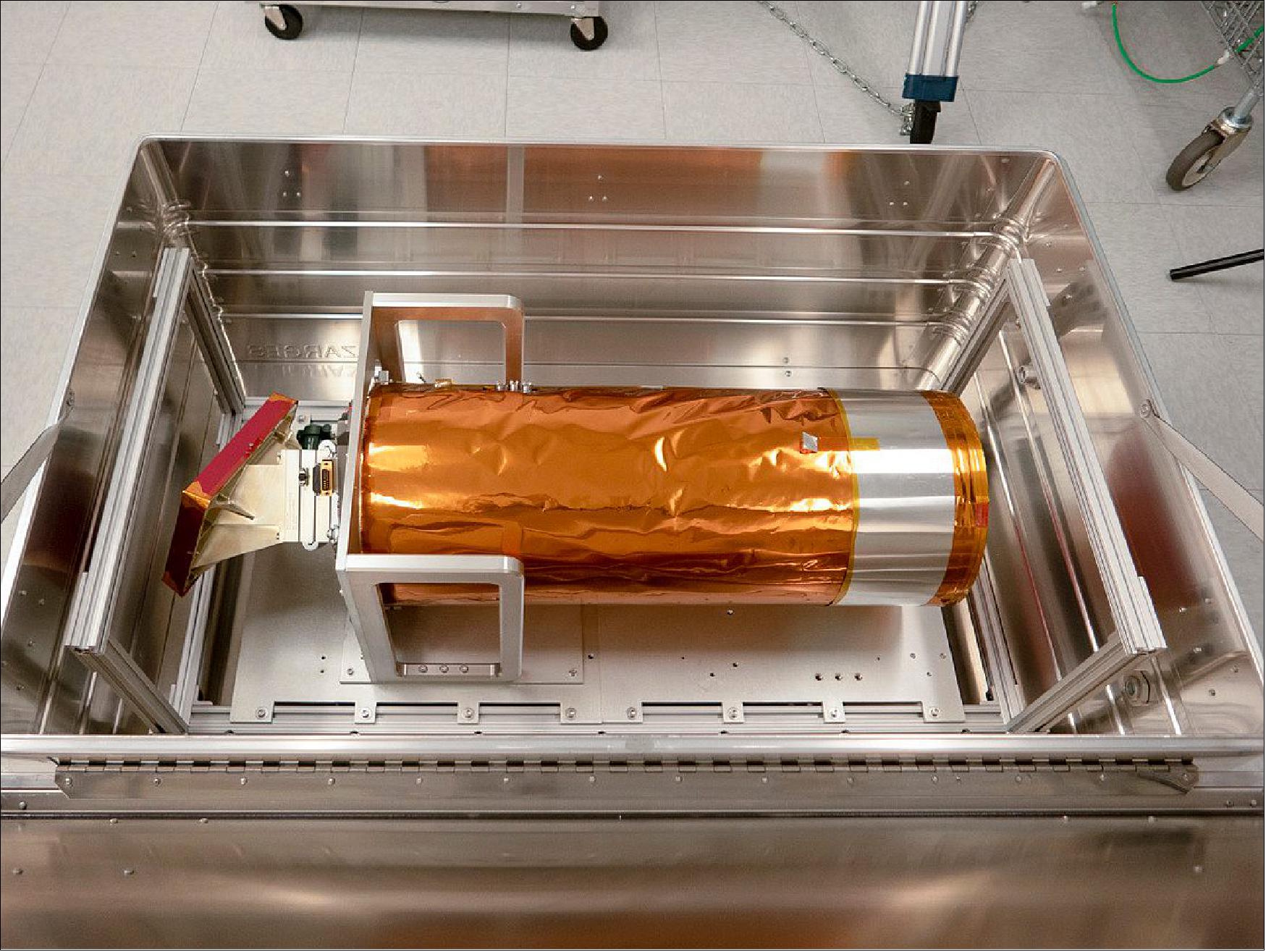
Ground Segment
An important segment of the KPLO mission is the ground segment. For the success of the KPLO mission, KARI has put much effort into developing, verifying, and validating the ground segment of the KPLO mission. The KDGS (KPLO Deep-space Ground System) is one of the components that completes the KPLO ground segment. The KDGS will be implemented at the KARI site with subsystems, including the KSDA (Korea Deep-Space Antenna). The top-level architecture of KDGS is shown in Figure 14 (Ref. 1).
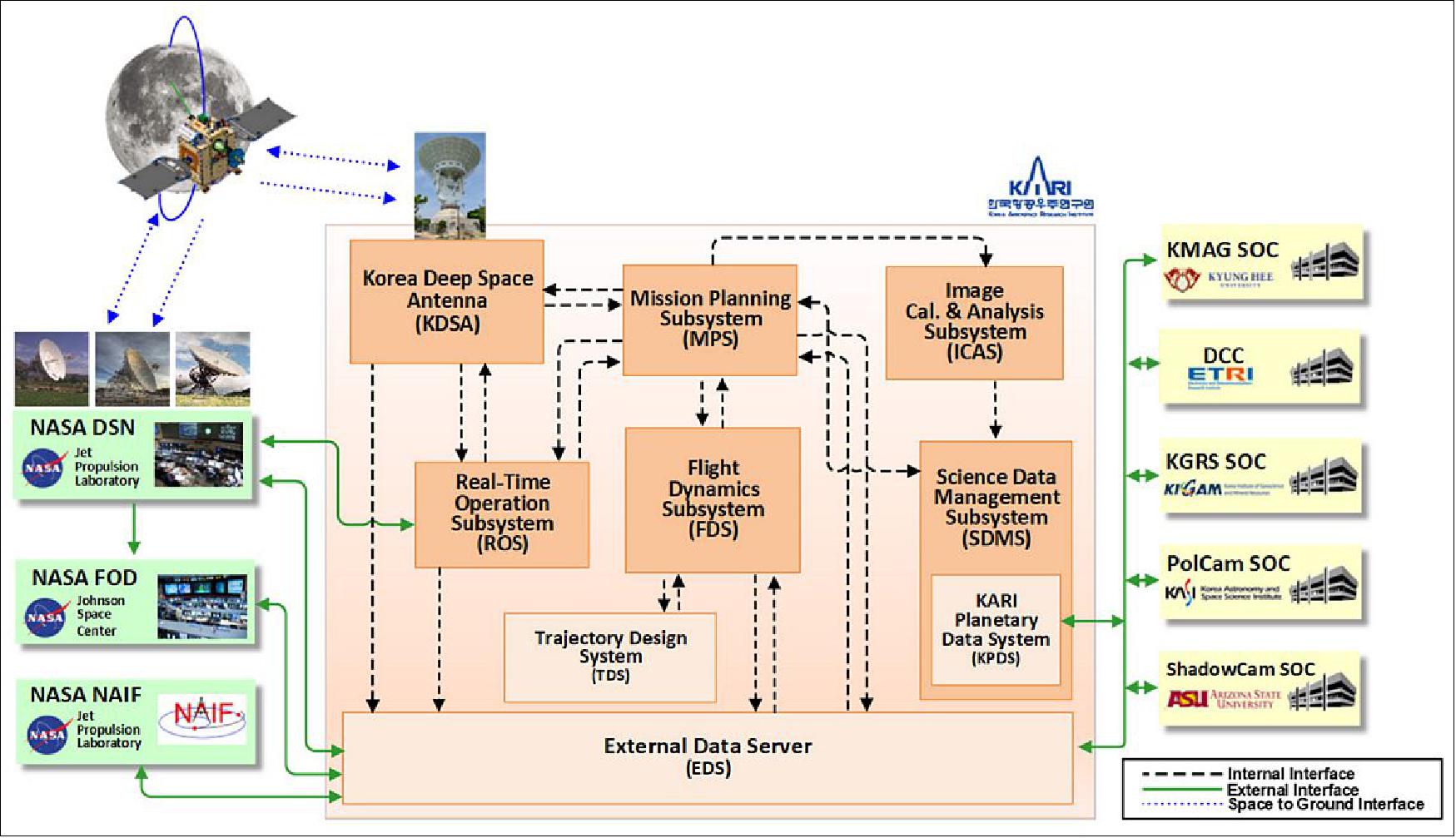
KDGS completes the KPLO ground segment with other entities, such as DCC (Disruption tolerant network Control Center) and the external Science Operation Center (SOC), through EGNS. The functionalities of major KDGS subsystems are as follows. KDSA is a 35 m aperture deep-space antenna with a high-power transmitter that is capable of tracking and communicating with KPLO during the mission. Figure 4 shows the state of KSDA, which is currently under construction. A real-time operation subsystem (ROS) will generate, send, and receive telecommands (TCs) and telemetry (TM), and will monitor the spacecraft health status in real time. The Mission Planning Subsystem (MPS) will generate mission timelines and commands for both the bus system and payloads. The MPS will also manage payload data gathering schedules to maximize data gatherings within a limited mission lifetime, while maintaining resource allocation constraints. LUTI data will be processed by the ICAS (Image Calibration and Analysis Subsystem) in KDGS, and the SDMS (Science Data Management Subsystem) will handle other science payload data and preprocess them to deliver payloads and developers for further scientific research. The science data products will comply with the PDS4 standard established by NASA.
As the KPLO program will utilize the NASA Deep Space Network (DSN), KDGS will have an interface with the NASA DSN. The Goldstone Deep-Space Communication Complex (DSCC), the Madrid DSCC, and the Canberra DSCC will be primary service facilities during the transfer phase. Goldstone and Madrid will still be the primary service facilities, while the Canberra DSCC will serve as the backup for KDSA anomalies during the nominal mission phases. KDGS will also have functions to support the Space Link Extension (SLE) protocol and the DTN node. Finally, the FDS (Flight Dynamics Subsystem) is one of the critical subsystems that is responsible for overall flight dynamics-related operations, which will be introduced in detail through this work.
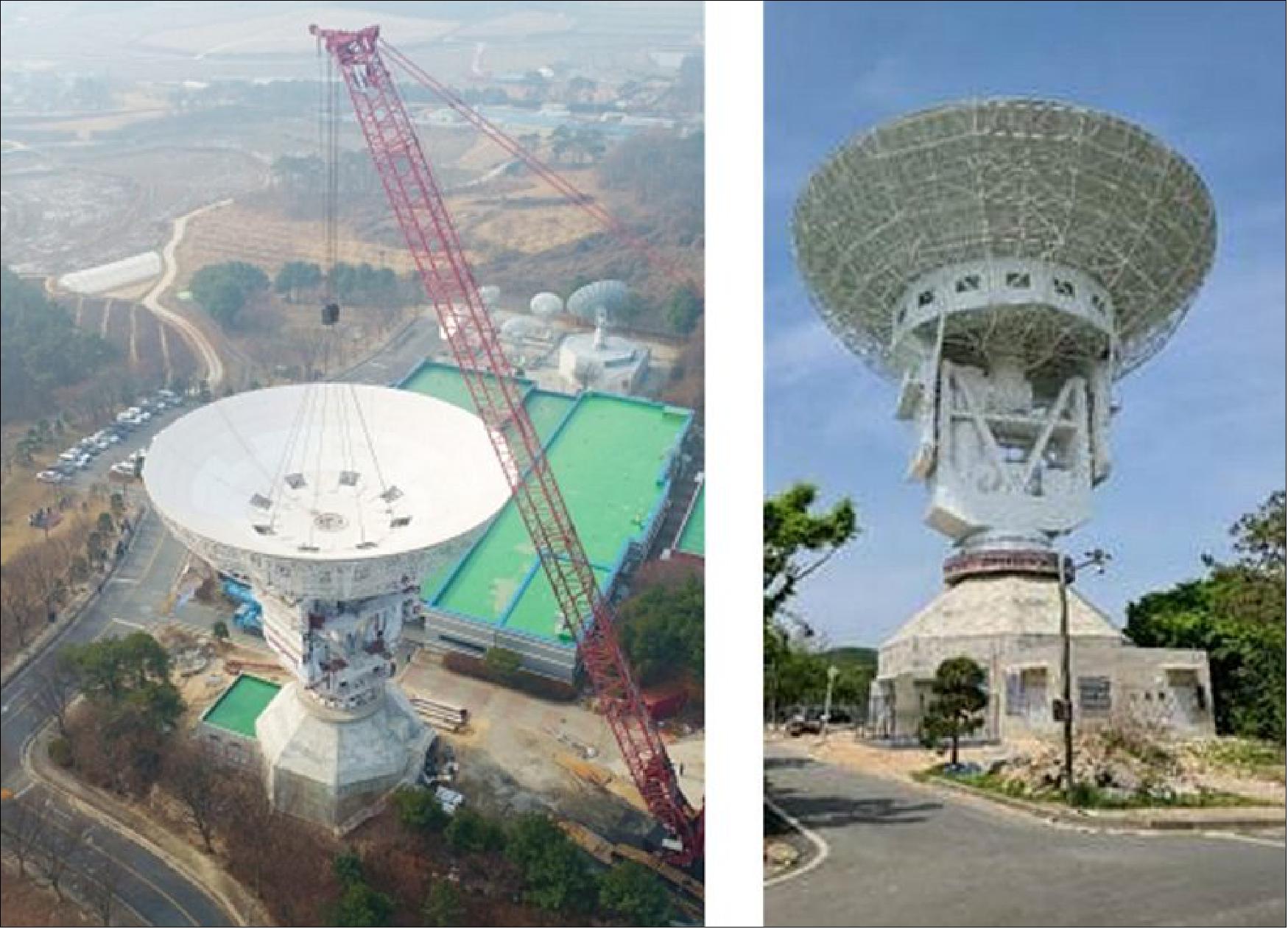
Design Philosophy of the FDS (Flight Dynamics Subsystem)
Despite many real flight dynamics backgrounds on LEO and GEO missions, the decision was made to adopt Commercial-Off-The-Shelf (COTS) software to formulate FDS for the KPLO mission. This decision was made to secure the operational stability related to flight dynamics, considering the characteristics of the KPLO being Korea’s first lunar mission. Among the several COTS software programs, the Orbit Determination Tool Kit (ODTK) and System Tool Kit (STK) with Astrogator (an add-in module of the STK) by Analytical Graphics Inc. (AGI) is selected. The criteria for selecting a COTS were whether there were cases applied to actual flight missions in the past and whether the FDS function could be freely implemented using COTS, that is, its expandability was considered the most. The Astrogator is the third and most recent version of a program originally developed by NASA Goddard Space Flight Center (GSFC) and has been used to design and operate many missions; not just Earth missions, but also missions with a central body other than the Earth, such as Clementine, Wind, SOHO (Solar and Heliospheric Observatory), ACE (Advanced Composition Explorer), Lunar Prospector, MAP, LRO (Lunar Reconnaisance Orbiter), and LADEE (Lunar Atmosphere and Dust Environment Explorer).
At the same time, in-house software, especially the core algorithm related to the flight dynamics of the planetary mission, is now being developed based on KARI’s heritage of past LEO and GEO missions [34–42] to prepare for future space exploration in Korea. During the operation of the COTS-based FDS, the performance of the developed in-house software will also be validated using the real navigation and flight data of the KPLO. After the KPLO mission, the authors strongly expect that the developed in-house software will replace the COTS software and be further used for space exploration missions in Korea after the KPLO mission.
Interface with the Trajectory Design System
While establishing the early FDS design concept, the decision was made not to include the trajectory design function into FDS, and instead prepare another subsystem called the Trajectory Design System (TDS) while maintaining interoperability with FDS. This was intended by regarding the characteristics of interplanetary trajectory design work, which can only be designed by a subject matter expert and that the design itself cannot be automated. Additionally, complex targeting sequences could be broken down many different ways depending on the results of previous execution results. Therefore, the final decision was made to operate standalone TDS by directly using STK/Astrogator.
One of the important features of the TDS is that the operational “template scenario” will be generated inside of the TDS and delivered to the FDS. This design strategy is the result of differentiation from the FDS design and operation of typical LEO and GEO satellite missions, which do not have a high dependence on trajectory or orbit design results during flight operation. More detailed reasons for this special feature will be explained in Section 3.3. During the flight operation of the KPLO, the TDS will maintain close contact with the FDS and will share many products. During KPLO’s operation, TDS will continuously observe whether KPLO follows the designed reference trajectory, i.e., whether the current trajectory information satisfies the predefined error boundary and will update reminders of the reference trajectory. In the process of checking whether KPLO follows the reference trajectory well, the TDS will receive and utilize many products generated by the FDS, including the navigation data, the current fuel mass estimates, the engine’s thrust, and Isp estimates with its efficiencies. During KPLO’s mission operation, the TDS operator will closely monitor the current status of KPLO, together with the FDS operator. In Figure 16, the top-level interoperability architecture between FDS and TDS is shown.
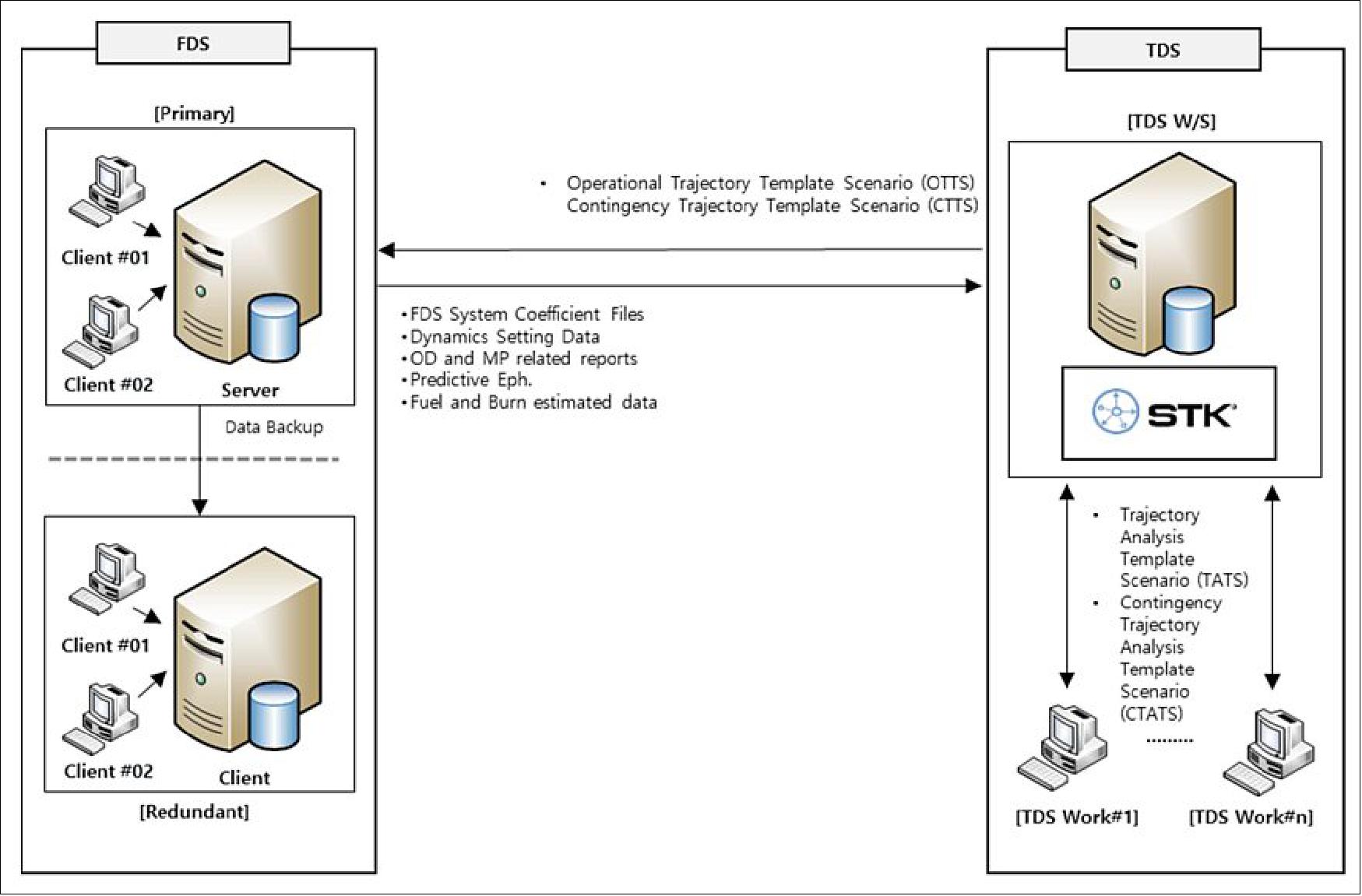
Template Scenario
The key feature of the KPLO FDS is that most of the FDS functional modules will be run by loading the reference “template scenario”. The FDS module executed by loading the template scenario is as follows: ODM, MPM, SPM, and some features of AM. Any existing previously saved sets of STK and ODTK scenario files can be directly used as template scenarios inside of the FDS. When establishing the concept of a “template scenario” loading-based operation, one of the top priorities considered was to automatically detect the activated parameters inside of the template scenario and to only display those parameters in the GUI of the FDS. This strategy will maintain user controllability while minimizing GUI changes, and even replacing the template scenarios. This effort resulted in the maximization of FDS applicability to any situation that may occur during the real-time operation, which could likely happen, namely, replacing the template scenario that has different parameters to achieve each module’s final objectives.
Similar to the KPLO FDS, the FDS for the LADEE mission also utilized COTS software (STK, Astrogator, and ODTK) and used a similar approach called operational “Procedures”. The procedure, in terms of LADEE FDS, is a list of script-based tasks written with the VB.NET language. Inside the procedures, there exists a subfunction called the “load scenario”, which is very similar to those of the KPLO FDS. However, to modify the task of LADEE FDS, the operator needs to know more specifics of the COTS tool’s Application Programming Interface (API) than the current design of KPLO FDS, since KPLO FDS loads the entire template scenario using just one click and automatically displays user-activated parameters. By applying KPLO FDS’s own unique strategy, wide ranges of trajectory options can flexibly consider achieving the final mission goal during real flight operations. Rapidly dealing with contingency situations during actual operation, i.e., unavoidable reference trajectory changes due to burn failure, could be one of the most promising applications as flight dynamics operators can reconstruct a reference trajectory to target a new aim point using any supporting parameters nested.
As an example of an MPM loading operational template scenario that will be constructed from the inside of the TDS, there will be two different sets of template scenarios called the Operational Trajectory Template Scenario (OTTS) and the Contingency Trajectory Template Scenario (CTTS). As their names indicate, the OTTS is constructed for the nominal operation phases of the entire mission, and the CTTS is intended to use when a contingency trajectory situation occurs. When producing OTTS, the most prioritized facts were to follow the designed reference trajectory well and, at the same time, satisfy the ΔV limits given in each of mission phases. The most ideal way is to use the scenario created to generate the reference trajectory in TDS directly as OTTS. Unfortunately, this is impossible, as most of the reference trajectories, especially KPLO, which uses the WSB/BLT trajectory, calculate the reference trajectory with various targeting parameters using forward-backward shooting at the same time. The template scenario in which the forward-backward shooting method is used is not suitable as an OTTS, and it needs to be converted to the template scenario utilizing only the forward sense. While converting into OTTS, various conditions need to be considered. Some important points are listed as follows. First, statistical TCM locations are properly located and nested inside an OTTS by regarding the expected navigation, trajectory, orbit prediction, and spacecraft burn performances. These locations of statistical TCMs are of no major interest when generating the reference trajectory itself inside of the TDS, except the location of the deterministic TCMs. Second, the OTTS should be constructed considering real-flight operation timelines, such as regarding the time zone of the operation center and the operator’s shift work schedule. Finally, and most importantly, the targeting parameter and associated convergence tolerances are set to be different while following the reference trajectory well rather than those of the original set of scenarios that are used for reference trajectory generation. These measures were used to consider the convergence performances as well as their speed, assuming the actual operation situation.
References
1) Young-Joo Song, Young-Rok Kim, Jonghee Bae, Jae-ik Park, Seungbum Hong, Donghun Lee and Dae-Kwan Kim, ”Overview of the Flight Dynamics Subsystem for Korea Pathfinder Lunar Orbiter Mission,” Aerospace 2021, 8, 222,Published: 10 August 2021, https://doi.org/10.3390/aerospace8080222, https://www.mdpi.com/2226-4310/8/8/222/htm
2) ”Korean Lunar Exploration Program,” KARI (Korea Aerospace Research Institute), URL: https://www.kari.re.kr/eng/sub03_04_01.do
3) ”NASA Selects Nine Scientists to Join Korea Pathfinder Lunar Orbiter Mission,” NASA Feature, 30 March 2021, URL: https://www.nasa.gov/feature/
nasa-selects-nine-scientists-to-join-korea-pathfinder-lunar-orbiter-mission
4) ”Korea Pathfinder Lunar Orbiter (KPLO),” NASA, 27 April 2021, URL: https://nssdc.gsfc.nasa.gov/nmc/spacecraft/display.action?id=KPLO
5) Sangyoun Shin, Haeng-Pal Heo, and Seok-Weon Choi, ”The Lunar Terrain Imager Operations Concepts,” SpaceOps conferences, 28 May - 1 June 2018, Marseille, France, URL: https://arc.aiaa.org/doi/pdfplus/10.2514/6.2018-2345
6) Sangyoun Shin, Haeng-Pal Heo, Seok-Weon Choi, Hyoungho Ko, ”The Lunar Terrain Imager Operations Concepts,” SpaceOps Conference, 28 May-1 June 2018, Marseille, France, URL: https://arc.aiaa.org/doi/pdfplus/10.2514/6.2018-2345
7) Chae Kyung Sim, Sungsoo S. Kim, Minsup Jeong, Young-Jun Choi, and Yuriy G. Shkuratov, ”Observational Strategy for KPLO/PolCam Measurements of the Lunar Surface from Orbit,” Publications of the Astronomical Society of the Pacific, Volume 132, Number 1007, Published: 17 December 2019, https://doi.org/10.1088/1538-3873/ab523d, URL: https://iopscience.iop.org
/article/10.1088/1538-3873/ab523d/pdf
8) J. Shin, H. Jin, H. Lee, S. Lee, S. Lee, M. Lee, B. Jeong, J.-K. Lee, D. Lee, D. Son, K-H. Kim, I. Garrick-Bethell and E. Kim, ”KMAG: The Magnetometer of the Korea Pathfinder Lunar Orbiter (KPLO) mission,” 50th Lunar and Planetary Science Conference 2019 (LPSI Contribution No. 2132), The Woodlands, March 18-22, 2019, URL: https://www.hou.usra.edu/meetings/lpsc2019/pdf/2276.pdf
9) Kim, Kyeong; Min, Kyoung Wook; Elphic, Richard; Re Choi, . Yi; Hasebe, Nobuyuki; Hiroshi Nagaoka; Park, Junghun; Yi, Eung Seok; Lee, Sungsoon; Yeon, Young-Kwang; Lee, K. B.; Kim, Yong-Kwon; Park, Kilsoon; In Kang, Kyung; Cho, Jin Yeon; ”Introduction to the lunar gamma-ray spectrometer for Korea Pathfinder Lunar Orbiter,” 42nd COSPAR Scientific Assembly, Held 14-22 July 2018, in Pasadena, California, USA, Abstract id. B3.1-24-18.
10) ”NASA Selects ‘ShadowCam’ to Fly on Korea Pathfinder Lunar Orbiter,” NASA, 28 April 2017, URL: https://www.nasa.gov/feature/nasa-selects-shadowcam-to-fly-on-korea-pathfinder-lunar-orbiter
11) Mark Robinson, ”ShadowCam Begins Long-Awaited Journey,” ASU, 18 August 2021, URL: http://shadowcam.sese.asu.edu/posts/1041
The information compiled and edited in this article was provided by Herbert J. Kramer from his documentation of: ”Observation of the Earth and Its Environment: Survey of Missions and Sensors” (Springer Verlag) as well as many other sources after the publication of the 4th edition in 2002. - Comments and corrections to this article are always welcome for further updates (eoportal@symbios.space).
Spacecraft Launch Sensor Complement Ground Segment References Back to top