LADEE (Lunar Atmosphere and Dust Environment Explorer)
Non-EO
NASA
Science
Quick facts
Overview
Mission type | Non-EO |
Agency | NASA |
Launch date | 07 Sep 2013 |
End of life date | 18 Apr 2014 |
LADEE (Lunar Atmosphere and Dust Environment Explorer)
Spacecraft Launch Mission Status Sensor Complement Ground Segment References
LADEE is a lunar science orbiter mission under development at NASA to address the goals of the NRC (National Research Council) decadal surveys and the SCEM (Scientific Context for Exploration of the Moon) report to study the pristine state of the lunar atmosphere and dust environment prior to significant human activities.
The goal of the LADEE mission is to determine the composition of the lunar atmosphere and investigate the processes that control its distribution and variability, including sources, sinks, and surface interactions. LADEE will also determine whether dust is present in the lunar exosphere, and reveal the processes that contribute to its sources and variability. These investigations are relevant in the understanding of surface boundary exospheres and dust processes throughout the solar system, address questions regarding the origin and evolution of lunar volatiles, and have potential implications for future exploration activities. 1) 2) 3) 4) 5) 6) 7) 8) 9) 10)
The top-level programmatic and science requirements for the LADEE project are designed to accomplish the following mission objectives:
• Determine the composition of the lunar atmosphere and investigate the processes that control its distribution and variability, including sources, sinks, and surface interactions.
• Characterize the lunar exospheric dust environment and measure any spatial and temporal variability and impacts on the lunar atmosphere
• Demonstrate that Lunar Laser Com Demonstration (LLCD) can operate at high data rates from lunar distances
• Create a low-cost reusable spacecraft architecture that can meet the needs of certain planetary science missions
• Demonstrate the capability of the Minotaur V as a launch vehicle for planetary missions.
Background
The moon is surrounded by the thinnest of possible atmospheres, which planetary scientists call an exosphere. Exosphere's are so thin that their atmospheric pressure is lower than what we can achieve in our hardest laboratory vacuums. Unlike the air in a dense atmosphere, the gas molecules almost never interact, so that exospheres can neither trap nor conduct heat, meaning that there is no weather or climate. It also means that aerosols like dust and smoke cannot float, but rather fall to the ground like rocks. 11)
This leads to one of the strangest discoveries made by those early Lunar missions: Dust levitates on the Moon. When the Sun rises, individual microscopic particles of dust rise up from the surface and form a thin haze of material kilometers up from the surface. When night falls, so does the dust. The most logical explanation is that individual high-energy photons of sunlight strike the dust particles and knock off a few electrons, leaving a positive charge on the dust. Positive repels positive, and the dust is thrown up into the air until it is high enough that the electrostatic repulsion is weak enough to be balanced by gravity. But without proper close-up observations and data, this theory remains merely a hypothesis.
A thorough understanding of the characteristics of our lunar neighbor will help researchers understand other small bodies in the solar system, such as asteroids, Mercury, and the moons of outer planets. This is because we now know that nearly all so-called airless bodies in the Solar System do actually possess exospheres of their own, which have an important effect on the long-term behavior of an object.
Since astronomers became aware of the Moon's exosphere, some have begun to worry about the effect we're having on the lunar environment. While there is no life on the Moon, and therefore no ecosystem to damage, the exosphere is still quite vulnerable. After all, while the emissions of a few rocket landings might not seem significant in the context of an entire world, there is so little gas in the exosphere that those few landings can significantly alter its composition - a fact confirmed when the Chinese Chang'e-3 mission landed in December 2013 and LADEE was able to detect the change in exosphere composition and dust volumes.
Because the exosphere is so thin, individual gas molecules hardly ever interact with each other, meaning that there is no atmospheric chemistry to speak of: chemical compounds do not break down, but last forever. This makes LADEE one of the most important active missions today, and the only one with an unmovable deadline: we need to study the exosphere right now, while we still can, before we change it forever.
Project status:
- In June 2013, the LADEE spacecraft arrived at NASA's Wallops Flight Facility to begin final processing for its trip to the moon. 12)
- In November 2012, NASA/ARC completed the initial electromagnetic interference tests of LADEE. 13)
- On August 2, 2011, the LADEE project passed the MCDR (Mission Critical Design Review). 14)
Spacecraft
The LADEE spacecraft bus design was derived from the MCSB (Modular Common Spacecraft Bus), architecture developed at NASA/ARC (Ames Research Center) from 2006-2008. The MCSB is a small, low-cost spacecraft designed to deliver scientifically and technically useful payloads to a variety of locations, including LEO (Low Earth Orbit), lunar orbit and lunar surface, Earth-Moon Lagrange points, and NEOs (Near Earth Objects). 15)
The spacecraft bus is a lightweight carbon composite structure designed to accommodate launch loads and provide attenuation of impact loads. It is also designed for ease of manufacturing and assembly. The modularity of the design is intended not only for multiple mission configurations but also parallelism in development and assembly. The system-level components were drawn from low-cost flight-proven product lines.
For LADEE, the spacecraft bus modules consist of (Figure 1): (1) the Radiator Module, which carries the avionics, electrical system, and attitude sensors, (2) the Bus Module, (3) the Payload Module, which carries the two largest instruments, (4) the Extension Module, which houses the propulsion system, and (5) the Propulsion Module.
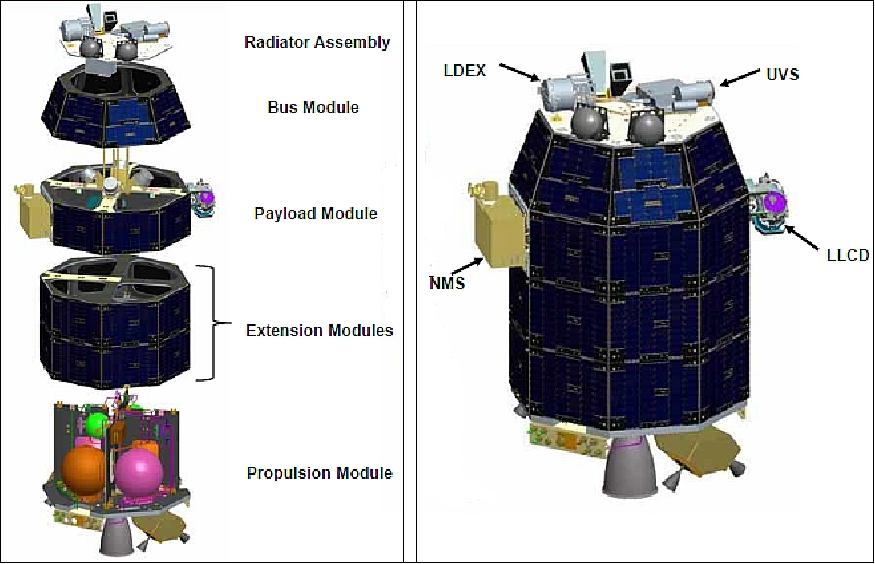
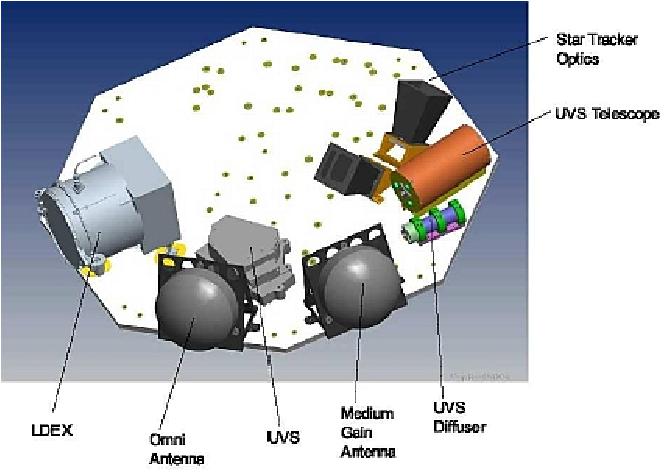
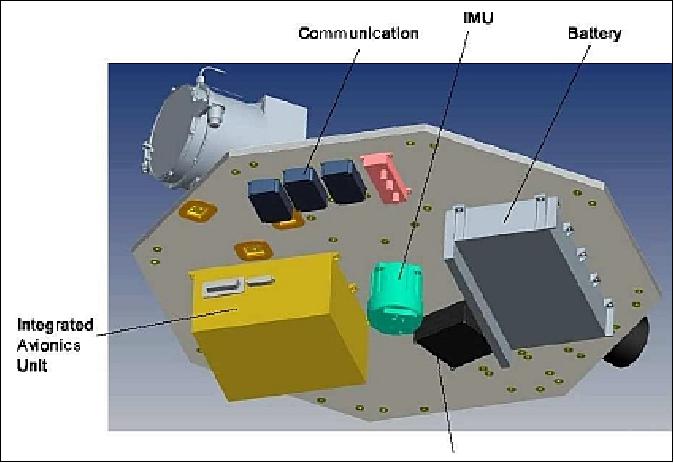
One prominent characteristic of the bus design is that the solar arrays are body-mounted and fixed. While this arrangement reduces the available power, it eliminates deployment and articulation mechanisms, which eliminates several failure modes. It also ensures power production in almost any attitude, which enables very robust safe modes. This process also allows spacecraft attitude to be used for thermal control, and eliminates a hot and a cold side, which minimizes the need for heater power. The spacecraft can be flown in either a spinner or 3-axis control mode.
Figure 4 shows the LADEE system block diagram. The flight avionics packages consists of a commercially available 8-slot 3U cPCI (Compact Peripheral Component Interface) integrated avionics system providing the following functions:
• Command & Data Handling avionics
• Power distribution
• Solar array and battery charge management
• Pyrotechnic actuation.
Propulsion system: The bipropellant propulsion system consists of a main thruster, six attitude control thrusters, two fuel tanks, two oxidizer tanks, two pressurant tanks, an ordnance valve driver box, and associated tubing and cabling. A separate electronics box in the Propulsion Module handles the valve driver actuation. - The propulsion system is being developed at SS/L (Space Systems/Loral) of Palo Alto, CA. NASA awarded a contract to SS/L in December 2009. 16)
The ACS (Attitude Control Subsystem) includes six 22 N thrusters using MMH (Monomethylhydrazine) propellant, with MON-3 oxidizer. The 6 thrusters are mounted in two canted pairs beneath the spacecraft's lower deck.
EPS (Electrical Power Subsystem): The EPS consists of an array of body-fixed solar panels, connected to batteries through the solar array control card within the avionics chassis. The body-fixed array design minimizes articulation on the spacecraft bus.
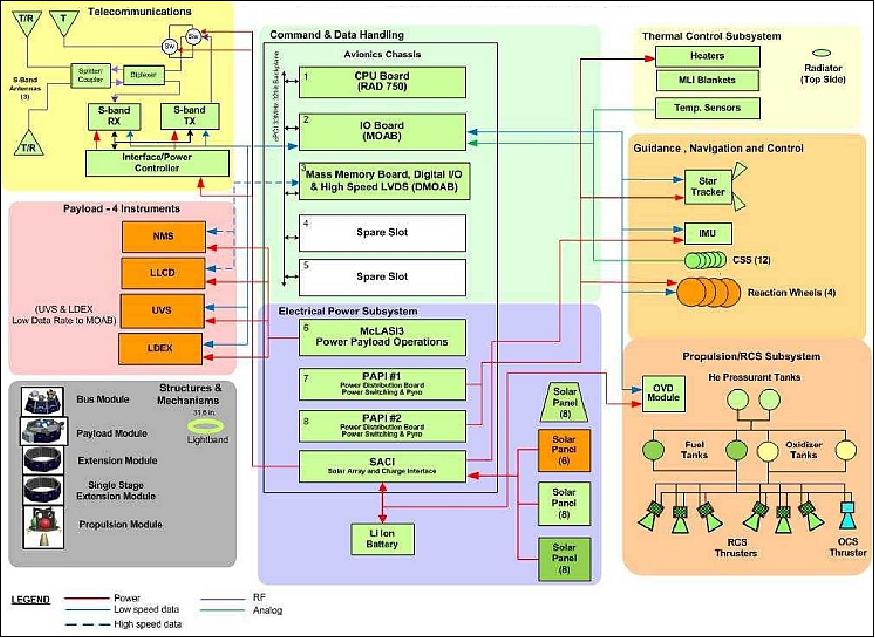

RF Communications
Use of a modular design. The radio has separate receiver, transmitter and IHPA modules and produces 5 W of RF transmitter power with flexible transmission power modes. An omnidirectional/medium gain antenna design of ARC is used to achieve omni-directional coverage with a smaller area of medium gain response.
LADEE makes use of the Space Micro µSTDN (San Diego, CA), a digitally intensive S-band transponder. The effects of received radio frequency power, downlink data rate and temperature on transponder's ranging accuracy were investigated. The LADEE flight ranging data is corrected using calibration data taken during thermal vacuum testing of the flight transponder. 17) 18)
The LADEE spacecraft's communication subsystem consists of two omnidirectional antennas, a medium-gain antenna and a single-string transponder, namely Space Micro's integrated µSTDN transponder (Figure 6). The integrated µSTDN transponder consists of a core transponder containing all the electronics, a diplexer to allow concurrent transmit and receive, an RF switch to select between the omnidirectional antennas and a medium gain antenna, and test couplers. The spacecraft's communication subsystem is compatible with NASA's NEN (Near Earth Network), DSN (Deep Space Network) and SN (Space Network).
The performance of the ranging system that is part of LADEE's µSTDN transponder is one of the reasons the mission could be extended for 28 days. This extension, representing a 15% lengthening greatly increases the science data return from the mission. The transponder combines analog coherence with digital ranging. This best-of-both-worlds approach achieves the accuracy of digital ranging without sacrificing the precise integer ratio of transmit-to-receive frequency that heritage STDN (Standard Tracking and Data Network) radios have used. Moreover, it does so without breaking the mass, power or cost budget of a small satellite while using exclusively EEE-INST-002 Level 2 EEE parts.
The PCM/PSK/PM modulation scheme is used for the low data rate uplink. The command (uplink) data is first phase-shift keyed onto a subcarrier which is then phasemodulated onto the uplink carrier. Similarly, the low data rate telemetry (downlink) data is phase-shift keyed onto a subcarrier which is then phase-modulated onto the telemetry carrier. When ranging mode is selected, the commanding signal is combined with the ranging channel and then phase-modulated onto the carrier. When coherent mode is selected, the telemetry carrier is frequency locked to the command carrier, thereby allowing Doppler measurements to be performed. The transponder is also capable of directly PSK-modulating the carrier for higher data rates.
The transponder's receiver locks to the residual carrier, subsequently to the command subcarrier and then demodulates the command data. When ranging mode is selected, the transponder strips the ranging channel from the received signal and filters it. The ranging channel is subsequently combined with the telemetry signal and phase modulated onto the telemetry carrier. The relative amount of power in each signal component is determined by the command and ranging (for uplink) or telemetry and ranging (for downlink) modulation indices.

Architecture of the Space Micro µSTDN: A basic block diagram of the µSTDN is shown in Figure 7. The RF subsystem amplifies and downconverts the uplink signal to a medium frequency intermediate frequency (IF), which is subsampled by the ADC (Analog Digital Converter). Digital filtering, downconversion and downsampling are performed in an FPGA. The carrier and subcarrier are recovered, and command data is demodulated and output. The ranging channel is stripped off and sent to the transmit modulator when turnaround ranging is selected. Because the ranging channel is digitized, it could readily be demodulated to perform regenerative ranging if that is desired. The digital receiver provides uplink RF power, AGC (Automatic Gain Control) and lock detect functions. Telemetry data is encoded, modulated, combined with the ranging channel and upconverted to a medium IF. The signal is then converted to the analog domain, upconverted to RF and amplified. A digitally intensive architecture with medium frequency IF was chosen to make the system highly reconfigurable with FPGA code changes, but without significant redesign of the hardware. This design can address all the common waveforms over the entire SGLS (Space-to-Ground Link Subsystem) and STDN frequency bands. The chosen architecture has the additional benefit that it does not significantly increase power consumption over a traditional analog design.
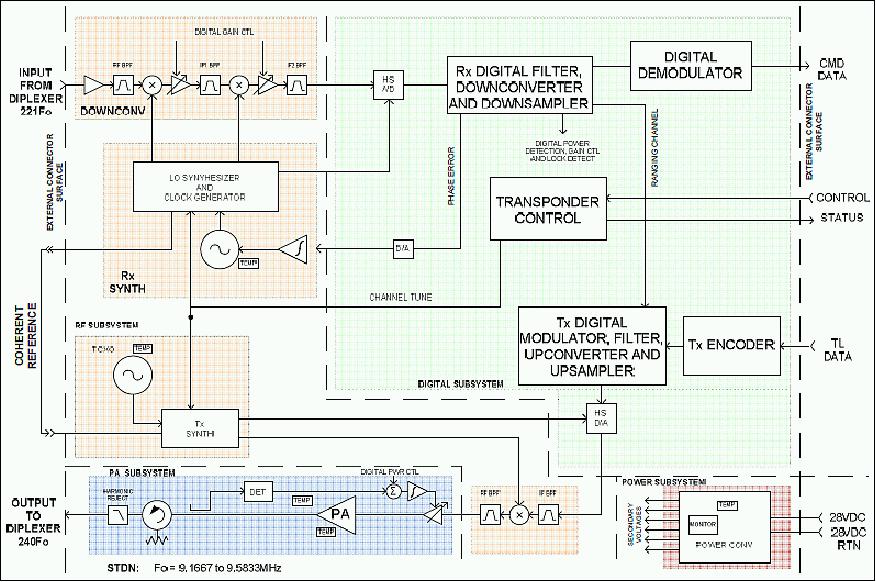
Ranging channel calibration: The ranging channel is digitally filtered to be approximately 800 kHz wide. The ranging turnaround function modulates the entire ranging channel, including noise onto the downlink. Ranging channel gain control is achieved by apportioning the ranging channel the correct predetermined modulation index in the transmit modulator.
The absolute delay of the ranging channel through the transponder is the compound delay of the transponder's RF front end, digital processing and the RF back end as well as the delay in the various passive elements in the RF chain such as the diplexer. The delay varies slightly with RF uplink power and temperature. During thermal vacuum (TVAC) testing, the range delay is measured over temperature and uplink power (Figure 8). A calibration model can be derived from this data.
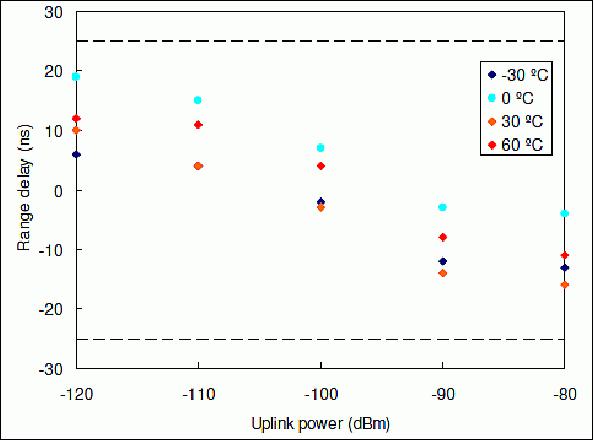
LADEE mission ranging data: During the LADEE mission, transponder turnaround ranging was used to update the orbital estimates. Uncalibrated range bias data for a 10 day period of September 2013, during the transfer orbit phase of the mission is shown in Figure 9. With the exception of some points which occur during spacecraft maneuvers, the range bias remains within a ±30 ns band. Nevertheless, there are clear patterns that can be discerned in the data.

As noted, the transponder's internal status indicators measure temperature and RF uplink power. These values for the same time period are shown in Figure 10. When the transponder is on the sun side of the spacecraft it heats up, and when it is on the other side it cools down. The AGC (Automatic Gain Control) reading shows how the uplink power varies as the antenna pattern moves relative to the ground receiver.
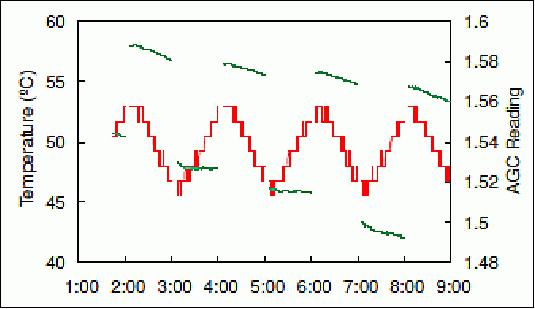
The two antennas have a known range offset of 16 ns which is mainly the result of the difference in cable length between the antenna and the transponder. The temperature and RF uplink power have been corrected using a simple linear model valid over the temperature and uplink power levels that are observed during flight.
The resulting range bias that has been fully calibrated for antenna, temperature and RF uplink power offsets is shown in Figure 11. The fit line shows the best fit to a second order polynomial. The root mean square estimate of the residue is just 2.2 ns. The residual range bias is the result of systematic drift in ground station time base and orbital motion.
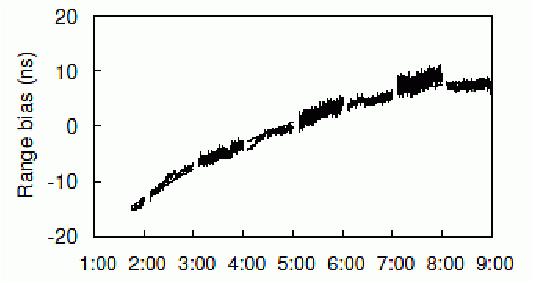
Correcting the range data for systematic transponder offsets aids interpretation of the flight data. Systematic range offsets are separated from residual random errors. Systematic range offset sources resulting from spacecraft orientation, data rate and ground station time base offset are identified and quantified.
Flight system performance: The LADEE communications subsystem performed reliably and consistently throughout all phases of the mission; over all modes of mission power, mechanical, and thermal environments experienced and data rates attempted, without hardware or firmware incident or significant performance anomaly (Ref. 18).
The communications subsystem - through a combination of factors - including overall, aggregated, subsystem performance, and primary use of more powerful and more sensitive DSN 34 m stations for telemetry and commanding than the designed-for NEN 18 m station, performed flawlessly with significant uplink/commanding and downlink/telemetry margins as was anticipated by the equipment and signal conditions. Downlink data margins were consistently much higher than minimum requirements, even at the maximum 128 kbit/s (unencoded)data rate licensed. Uplink data margins were similarly high, especially when maximum station uplink power was used to assure the command path.
There were no operationally significant unanticipated or unforeseen behaviors of the communication subsystem hardware and firmware. However, there were in-flight signal path behaviors attributable to known and empirically quantified RF phenomenon such as increased solar flux and anticipated multipath RF signal phenomenon near acquisition- and loss-of-earth views. These factors had to be tracked and qualified dynamically for the specific operating conditions and orientations the spacecraft was flown in to aid the Real Time Operations team in real-time activity execution planning.
In light of and learning from the Lessons Learned, the LADEE communications subsystem operated successfully with minor issues and significant margin during the full duration of the LADEE mission: September 2013 through April 2014. As the subsystem contained many new and/or never before-flown elements, the LADEE communication subsystem paved the way for future small satellite communication subsystems in terms of newer, more powerful, flexible, shorter lead time, and lower cost transponder and antenna system designs and technologies suitable for the new generation of lower cost, shorter lead time, higher risk, small spacecraft missions.
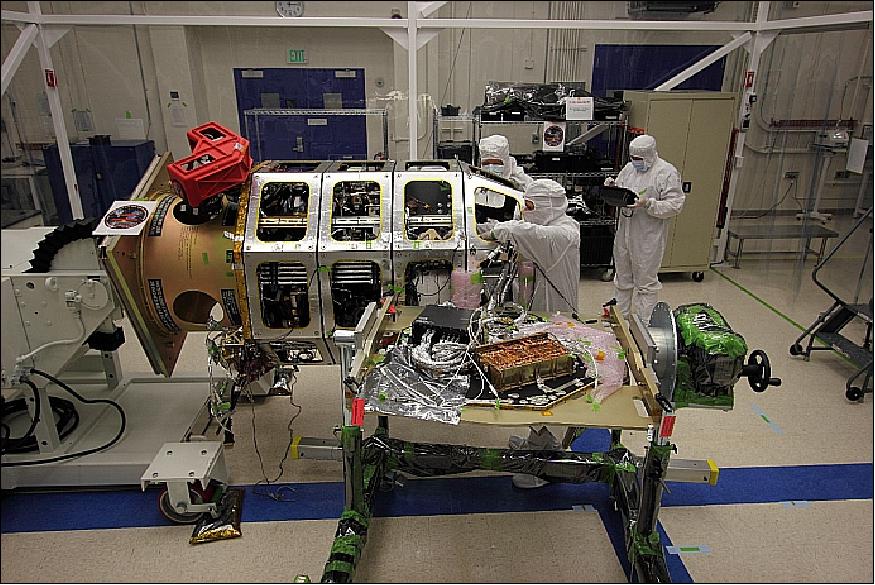
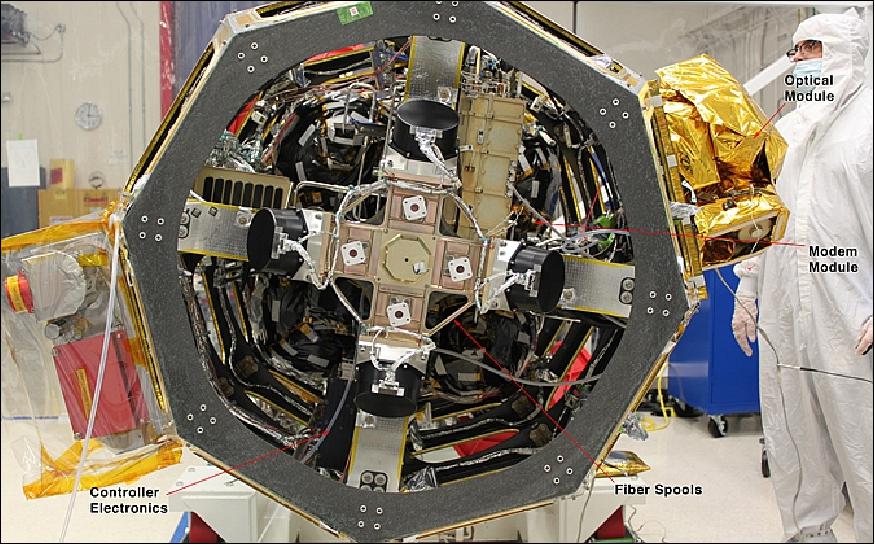
The LADEE spacecraft has a launch mass of ~ 383 kg, the bus diameter is 117 cm, power of ~ 295 W and a mission duration of ~160 days (30 days to travel to the moon, 30 days for checkout and 100 days for science operations).
Launch
The LADEE spacecraft was launched on September 7, 2013 (UTC 3:37:00) on a Minotaur-5 vehicle of OSC (a 5-stage converted Peacekeeper missile). This represented the maiden flight of the Minotaur-V rocket. The launch site was the commercial MARS (Mid-Atlantic Regional Spaceport) facility on Wallops Island, VA. 20) 21) 22)
Lunar orbit: Low-altitude (50 km) retrograde equatorial orbit.
LADEE will spend ~60 days reaching nominal lunar orbit and checking out systems before its 100 day science mission starts (it will take 30 days to enter lunar orbit). The nominal science orbit will be a near-circular (about 50 km) retrograde equatorial orbit with a period of 113 minutes. The periselene will be over the sunrise terminator. 23)
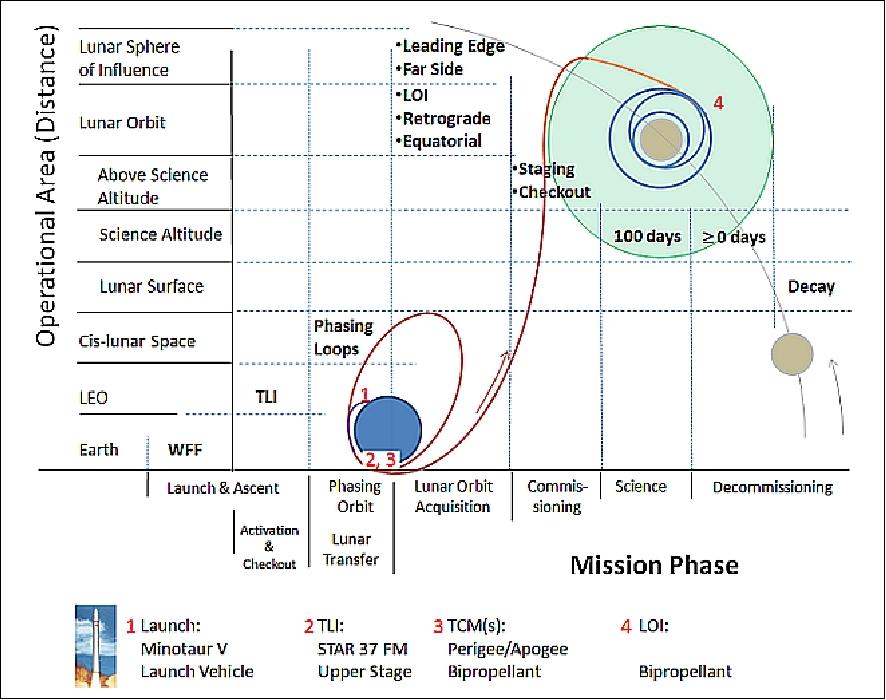

Mission Status
• April 15, 2019: Researchers from NASA and JHU/APL ( Johns Hopkins University /Applied Physics Laboratory) in Laurel, Maryland, report that streams of meteoroids striking the Moon infuse the thin lunar atmosphere with a short-lived water vapor. 24)
- The findings will help scientists understand the history of lunar water — a potential resource for sustaining long term operations on the Moon and human exploration of deep space. Models had predicted that meteoroid impacts could release water from the Moon as a vapor, but scientists hadn't yet observed the phenomenon.
- Now, the team has found dozens of these events in data collected by NASA's LADEE (Lunar Atmosphere and Dust Environment Explorer) was a robotic mission that orbited the Moon to gather detailed information about the structure and composition of the thin lunar atmosphere, and determine whether dust is lofted into the lunar sky.
- "We traced most of these events to known meteoroid streams, but the really surprising part is that we also found evidence of four meteoroid streams that were previously undiscovered," said Mehdi Benna of NASA's Goddard Space Flight Center in Greenbelt, Maryland, and the University of Maryland Baltimore County. Benna is the lead author of the study, published in Nature Geosciences. 25)
- The newly identified meteoroid streams, observed by LADEE, occurred on Jan. 9, April 2, April 5 and April 9, 2014.
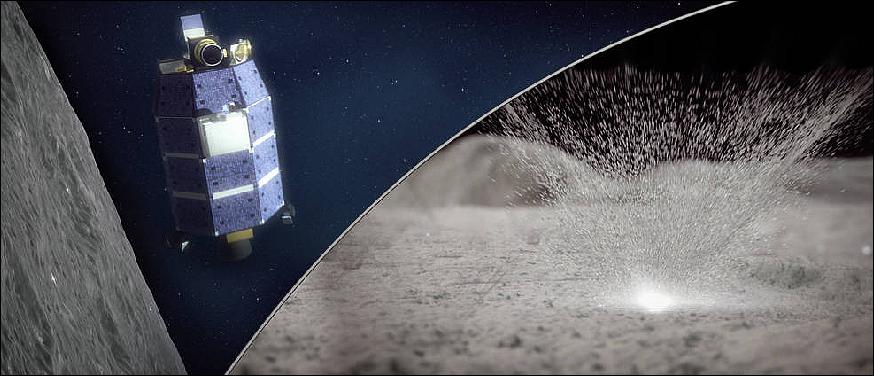
- There's evidence that the Moon has water (H2O) and hydroxyl (OH), a more reactive relative of H2O. But debates continue about the origins of the water, whether it is widely distributed and how much might be present.
- "The Moon doesn't have significant amounts of H2O or OH in its atmosphere most of the time," said Richard Elphic, the LADEE project scientist at NASA's Ames Research Center in California's Silicon Valley. "But when the Moon passed through one of these meteoroid streams, enough vapor was ejected for us to detect it. And then, when the event was over, the H2O or OH went away."
- Lunar scientists often use the term "water" to refer to both H2O and OH. Figuring out how much H2O and how much OH are present is something future Moon missions might address.
- LADEE, which was built and managed by NASA's Ames Research Center in California's Silicon Valley, detected the vapor using its Neutral Mass Spectrometer, an instrument built by Goddard. The mission orbited the Moon from October 2013 to April 2014 and gathered detailed information about the structure and composition of the lunar atmosphere, or more correctly, the "exosphere" – a faint envelope of gases around the Moon.

- From the measurements of water in the exosphere, the researchers calculated that the hydrated layer has a water concentration of about 200 to 500 parts per million, or about 0.02 to 0.05 percent by weight. This concentration is much drier than the driest terrestrial soil, and is consistent with earlier studies. It is so dry that one would need to process more than a metric ton of regolith in order to collect 16 ounces of water.
- Because the material on the lunar surface is fluffy, even a meteoroid that's a fraction of an inch (5 mm) across can penetrate far enough to release a puff of vapor. With each impact, a small shock wave fans out and ejects water from the surrounding area.
- When a stream of meteoroids rains down on the lunar surface, the liberated water will enter the exosphere and spread through it. About two-thirds of that vapor escapes into space, but about one-third lands back on the surface of the Moon.
- These findings could help explain the deposits of ice in cold traps in the dark reaches of craters near the poles. Most of the known water on the Moon is located in cold traps, where temperatures are so low that water vapor and other volatiles that encounter the surface will remain stable for a very long time, perhaps up to several billion years. Meteoroid strikes can transport water both into and out of cold traps.
- The team ruled out the possibility that all of the water detected came from the meteoroids themselves.
- "We know that some of the water must be coming from the Moon, because the mass of water being released is greater than the water mass within the meteoroids coming in," said the second author of the paper, Dana Hurley of the Johns Hopkins University Applied Physics Laboratory.
- The analysis indicates that meteoroid impacts release water faster than it can be produced from reactions that occur when the solar wind hits the lunar surface.
- "The water being lost is likely ancient, either dating back to the formation of the Moon or deposited early in its history," said Benna.
- NASA is leading a sustainable return to the Moon with commercial and international partners to expand human presence in space and bring back new knowledge and opportunities.
• December 17, 2015: NASA scientists have released new findings about the moon's tenuous exosphere – the thin layer of gas surrounding the moon that's one 25-trillionth the density of Earth's atmosphere. The data reveal, for the first time, that meteoroid strikes cause a predictable increase in the abundance of two key elements within the lunar exosphere. 26)
- Physical processes such as meteoroid stream impacts, the bombardment of helium and hydrogen particles from the sun, thermal absorption, and space weathering constantly modify the moon's surface as they work within the lunar exosphere. NASA's LADEE spacecraft observed an increase in exospheric gases when the rain of meteoroid impacts increases during a stream. These interplanetary grains can hit the lunar surface at speeds exceeding 34 km/s, releasing immense heat, and vaporizing part of the soil and meteoroids themselves.
- Within this vapor are sodium and potassium gases. LADEE's UVS (Ultraviolet Visible Spectrometer ) instrument measured levels of sodium and potassium around the moon every 12 hours for more than five months. These frequent readings revealed a dynamic rise of gas levels in the exosphere as meteor streams bombarded the moon, with the concentrations of both elements returning to normal background levels after the stream passed. Interestingly, the time it took to return to "normal" was dramatically different for the two gases, with potassium returning to its pre-shower state within days, while sodium took several months.
- Researchers will incorporate these observations into exosphere models of the Moon and similar bodies to help NASA unravel the mysteries of how our solar system originated and is changing over time. "To understand the Moon's exosphere requires insight into the processes controlling it, including the interaction of meteoroid showers as well as solar wind bombardment and ultraviolet radiation of the surface," said Anthony Colaprete, researcher at NASA/ARC (Ames Research Center) in Moffett Field, California, and principal investigator of the UVS instrument. "Understanding how these processes modify the exosphere allows researchers to infer its original state. Since these processes are ubiquitous across the solar system, knowledge gained by examining the Moon's exosphere can be applied to a range of other bodies, granting us greater insight into their evolution through time."
• March 16, 2015: Post mission analysis. A major goal of the mission was to characterize the dust exosphere prior to future lunar exploration activities, which may alter the lunar environment. The UVS (Ultraviolet/Visible Spectrometer) onboard LADEE addressed this goal, utilizing two sets of optics: a limb-viewing telescope, and a solar-viewing telescope. 27)
- The project examined two tail observation activities – one in January 2014, immediately after the Quantrantid (QUA) meteoroid stream encounter, and one in April 2014, during an extended hiatus in meteoroid stream activity. Just after the Quadrantids, the UVS spectral data show a "blue slope" at 230-475 nm. This blue slope fades away as the spacecraft's line-ofsight moves out of the tail. The 230 -475 nm region blue-sloped spectra are well-modeled by the backscattering of sunlight from nanoparticles with radii less than 20 or 30 nm.
- Spectral data from mission times coinciding with low meteoroid stream activity (e.g. the April 2014 dataset) still indicate the presence of dust, but at lower concentrations than seen after the Quadrantids.
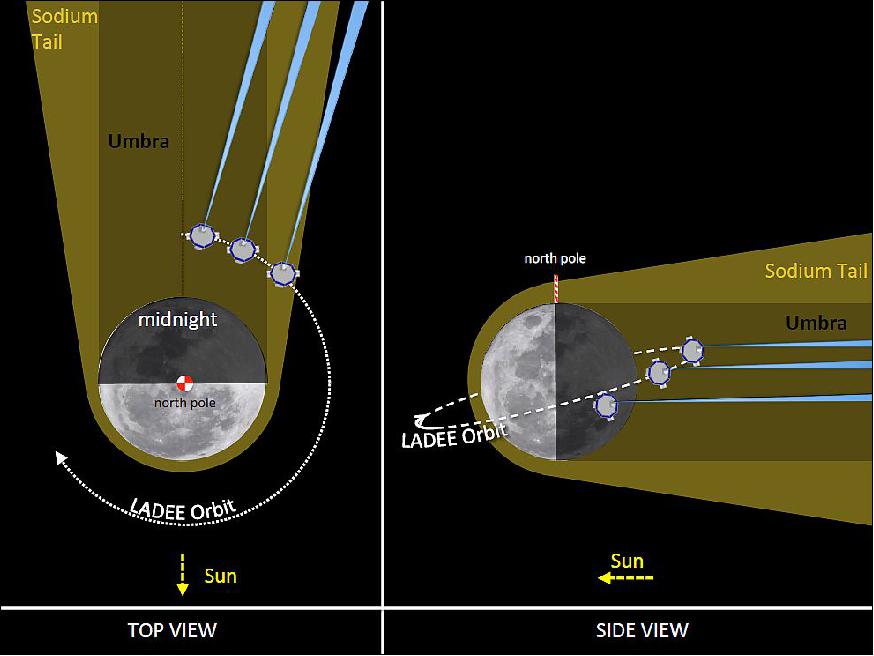
• October 2014: NASA'S LRO (Lunar Reconnaissance Orbiter) spacecraft has spied a new crater on the lunar surface; one made from the impact of NASA's LADEE mission. The LROC (Lunar Reconnaissance Orbiter Camera) team developed a new computer tool to search the NAC (Narrow Angle Camera) before and after image pairs for new craters. 28)
- LADEE's engines fired April 11, 2014, to perform a final orbital maintenance maneuver and adjust to guarantee it would impact on the farside of the moon and away from the Apollo landing sites. Over a seven-day period, LADEE's orbit decreased and the spacecraft orbited very low to the surface and close to the walls of lunar craters and mountain ridges to give the team a chance to collect valuable science data. Finally, LADEE impacted the eastern rim of Sundman V crater on April 18. The impact site is about 780 m from the crater rim with an altitude of about 2,590 m and was only about 300 m north of the location mission controllers predicted based on tracking data.
- The impact crater is small, less than 3 m in diameter, barely resolvable by the LROC NAC. The crater is small because the spacecraft — compared to most celestial impacts — was not traveling very fast, approximately 1,699 m/s and had a low mass and a low density. The size of the impact crater made it hard to identify among the myriad of small fresh craters on the lunar surface. Images acquired of the impact region before the impact, were compared with images obtained after the impact to identify the crater.
- Since the NAC images are so large (250 Mpixels) and the new crater is so small, the LROC team co-registered the before and after images (called a temporal pair) and then divided the before image by the after image. By doing this, changes to the surface become evident.

• May 2014: NASA considers the LLCD (Lunar Laser Communication Demonstration) mission to have been a great success. All the functions operated as predicted or better. The pointing, acquisition and tracking systems were robust and instantaneous. Useful data services were demonstrated and found to be dependable. A rudimentary multi-site ground terminal network was developed and demonstrated. Operations with the NASA spacecraft were made routine. It was demonstrated that optical links could be set up without special hands-on interactions, and a ground station handover was demonstrated. Many lasercom system design approaches were validated, including specifying and validating the spacecraft-terminal interface, building the lasercom links to work through turbulence, operating lasercom as part of an ongoing science mission, employing multi-mode photon-counting receivers, using ground telescope arrays on both the uplink and downlink, employing an inertially-stabilized space telescope, including high-accuracy ranging as a by-product of the lasercom links [Ref. 71) and 72)]. 29)
- NASA plans to follow LLCD with the LCRD (Laser Communications Relay Demonstration) mission. LCRD leverages the knowledge and experience gained from LLCD, and it will consist of two optical communications terminals to be launched to GEO in 2018 as a hosted payload on a commercial communications satellite. It will also have two ground stations in the United States that will support bi-directional communications at 1.25 Gbit/s. In addition, NASA is currently studying the concept of putting an optical communications terminal on the ISS (International Space Station) to communicate with LCRD to enhance the demonstration. LCRD is seen as a pathfinder for NASA's Next Generation TDRS (Tracking and Data Relay Satellite) series. 30)
• April 25, 2014: The OGS (Optical Ground Station) of ESA (European Space Agency) on Tenerife Island (Canary Islands, Spain) received laser signals from the LADEE spacecraft just a few days prior to LADEE's mission end - achieving data rates of 80 Mbit/s. This speed is high enough to transmit an entire movie DVD in about eight minutes and is many times faster than provided by traditional radio links used by today's spacecraft. 31)
- In the first round of laser communication demonstrations with LADEE in October 2013, data rates of 40 Mbit/s were obtained. The ESA team also demonstrated at the time, that it could transmit laser signals to LADEE and even obtain highly accurate range data, just like the traditional but much larger radio tracking stations can. Overall, the test series had been a big success.
- ESA will now refine the European laser communication technology to support live tests in the near future with other missions. These include NASA's OPALS (Optical PAyload for Lasercomm Science) system, delivered to the International Space Station on April 20, 2014 (SpaceX-3 mission), and Japan's SOTA (Small Optical Transponder) on the SOCRATES (Space Optical Communications Research Advanced Technology Satellite) microsatellite mission of NICT (National Institute of Information and Communications Technology). SOCRATES is a secondary payload on the ALOS-2 mission, scheduled for launch in May 2014.
• April 22, 2014: LADEE ran its science instruments almost non-stop right up to impact the evening of April 17, 2014, in an effort to gather as much low-altitude data as possible. Further study of the returned data will reveal what the instruments saw at these amazingly low orbits, just a few kilometers above the surface. Early results suggest that LADEE was low enough to see some new things, including increased dust density and possibly new atmospheric species. In an incredible race with time, LADEE's Real Time Operations team queued and downloaded all science files just minutes prior to LADEE's impact. 32) 33)
- As the clock was running out on the LADEE mission, the project team took advantage of an opportunity to replicate observations by the Apollo astronauts more than 40 years ago — using one of the star tracker cameras to gaze out over the Moon's horizon, while LADEE was in the deep darkness of the lunar night and over the far side where no Earthshine can reach.
- In the minutes before orbital sunrise, when LADEE emerged from shadow into sunlight, the team commanded the spacecraft to take a series of images. The goals was to see the same scene the astronauts saw, with the sun just below the horizon. In this configuration, one was able to view anything that might scatter sunlight. On Earth, "rosy-fingered dawn" paints the sky prior to sunrise because aerosols and dust particles suspended in the atmosphere scatter the sunlight. With the sun below the horizon, the reflected and scattered light makes the sunrise glow for an observer to see in the dark shadow beyond. However, the very low dust densities that LADEE/LDEX (Lunar Dust Experiment) measured should not produce such a sunrise glow – there were just too few particles along the line of sight to scatter measurable light. Yet some Apollo astronauts reported a pre–sunrise glow and even rays of light, as if the sun was shining through notches of the lunar mountains, and the light was scattered by .... something. Could LADEE spot this?

Legend to Figure 21: The image is one of the last frames in a series of images taken on April 12. — The series begins with LADEE viewing the lunar horizon ahead, a few minutes before orbital sunrise. At this position, there is already a glow in the sky above the completely dark surface of the moon, though the sun is many degrees below the horizon. LADEE's orbital motion makes the stars appear to move to the left. The same motion brings the sun closer to the horizon ahead and the glow gets brighter. In fact, the glow becomes so bright, parts of the image are saturated. Finally, sunrise fully saturates the camera image. This sequence is the closest thing to the astronaut's orbital viewpoint that LADEE could provide!
• On April 18, 2014, ground controllers at NASA/ARC confirmed that the LADEE spacecraft impacted the surface of the moon, as planned, on April 17. LADEE lacked fuel to maintain a long-term lunar orbit or continue science operations and was intentionally sent into the lunar surface. The spacecraft's orbit naturally decayed following the mission's final low-altitude science phase. 34) 35)
- On April 11, LADEE performed a final maneuver to ensure a trajectory that caused the spacecraft to impact the far side of the moon, which is not in view of Earth or near any previous lunar mission landings. LADEE also survived the total lunar eclipse on April 14 to 15, 2014. This demonstrated the spacecraft's ability to endure low temperatures and a drain on batteries as it, and the moon, passed through Earth's deep shadow.
- LADEE gathered detailed information about the structure and composition of the thin lunar atmosphere. In addition, scientists hope to use the data to address a long-standing question: Was lunar dust, electrically charged by sunlight, responsible for the pre-sunrise glow seen above the lunar horizon during several Apollo missions?
• April 3, 2014: The LADEE spacecraft is gradually lowering its orbital altitude to continue making science observations prior to its planned impact on the moon's surface on or before April 21. Ground controllers at NASA/ARC (Ames Research Center) in Moffett Field, CA, are maneuvering the spacecraft to fly approximately 2 -3 km above the lunar surface to gather science measurements at the lowest altitude possible. 36)
- A final maneuver will ensure LADEE's trajectory will impact the far side of the moon, which is not in view of Earth or near any previous lunar mission landings. Ground controllers have little room for error with LADEE's navigation system, and at these low orbital altitudes, a small error could mean the difference between continuing to orbit above the lunar surface and impacting it. Because of this, the team does not intend to target a specific impact location on the moon's surface.
- Until mid-April, ground controllers will continue to fire the LADEE altitude control thrusters once a week to keep the observatory in its target orbit. On April 11, LADEE will perform its final orbital maintenance maneuver before the total lunar eclipse on April 15, when Earth's shadow passes over the Moon. This eclipse, which will last approximately four hours, exposes the spacecraft to conditions at the limits of what it was designed to withstand.
- After the eclipse, ground controllers will determine how well the spacecraft is functioning. If it is healthy, LADEE will continue to acquire and transmit science data, as longs as its altitude and contact with ground controllers allow.
- LADEE's three science payload instruments have been working to unravel the mysteries of the moon's atmosphere, acquiring more than 700,000 measurements. In its previous orbit, LADEE's closest approach to the lunar surface was between 20 and 50 km, and its farthest was between 75 and 150 km — a unique position that allows the spacecraft to frequently pass from lunar day to lunar night every two hours. This vantage provides data on the full range of changes and processes occurring within the moon's tenuous atmosphere.
- Scientists hope this data will help answer a long-standing question: Was the lunar dust, electrically charged by sunlight, responsible for the pre-sunrise glow detected during several Apollo missions above the lunar horizon? LADEE also is gathering detailed information about the structure and composition of the thin lunar atmosphere. A thorough understanding of these characteristics of our nearest celestial neighbor will help researchers understand other bodies in the solar system, such as large asteroids, Mercury, and the moons of the outer planets.
• April 1, 2014: LADEE successfully completed in March its 100-day prime science phase and is operating in an extended science phase. Instruments aboard the spacecraft will continue to make science observations and acquire data at progressively lower altitudes until the planned impact.
• Feb. 13, 2014: The LADEE observatory successfully downlinked images of the moon and stars taken by onboard camera systems, known as star trackers. This is the first time the LADEE team commanded the spacecraft to send these pictures back to Earth. The main job of a star tracker is to snap images of the surrounding star field so that the spacecraft can internally calculate its orientation in space. It completes this task many times per minute. The accuracy of each of LADEE's instruments' measurements depends on the star tracker calculating the precise orientation of the spacecraft. 37)
• January 31, 2014: NASA's LADEE observatory has been approved for a 28-day mission extension. The spacecraft is now expected to impact the lunar surface on or around April 21, 2014, depending on the final trajectory. The extension provides an opportunity for the satellite to gather an additional full lunar cycle worth of very low-altitude (12-60 km) data to help scientists unravel the mysteries of the moon's atmosphere. 38)
• On January 15, LROC (Lunar Reconnaissance Orbiter Camera) of orbital colleague LRO (Lunar Reconnaissance Orbiter) snapped a picture of LADEE. LADEE is in an equatorial orbit (east-to-west) while LRO is in a polar orbit (south-to-north). The two spacecraft are occasionally very close and on Jan. 15, 2014, the two came within 9 km of each other. As LROC is a pushbroom imager, it builds up an image one line at a time, so catching a target as small and fast as LADEE is tricky. 39)
• On January 5, 2014, the LADEE spacecraft completed 1000 lunar orbits. LADEE continues to perform splendidly. In the evening of Jan. 19, LADEE executed a near-perfect periapsis-raising maneuver, one of a series of maneuvers designed to maintain LADEE's science orbit within the desired altitude range. Without these maneuvers, LADEE's orbit would decay in a matter of days, resulting in premature impact on the lunar surface. 40)
• Dec. 27, 2013: The completion of the 30-day LLCD (Lunar Laser Communication Demonstration) mission has revealed that the possibility of expanding broadband capabilities in space using laser communications is as bright as expected. 41)
- For example, LLCD demonstrated error-free communications during broad daylight, including operating when the moon was to within three degrees of the sun as seen from Earth. LLCD also demonstrated error-free communications when the moon was low on the horizon, less than 4 degrees, as seen from the ground station, which also demonstrated that wind and atmospheric turbulence did not significantly impact the system. LLCD was even able to communicate through thin clouds, an unexpected bonus.
- Operationally, LLCD demonstrated the ability to download data from the LADEE spacecraft itself. The project was able to download LADEE's entire stored science and spacecraft data (1 GB) in less than five minutes, which was only limited to the 40 Mbit/s connection to that data within LADEE. Using LADEE's onboard radio system would take several days to complete a download of the same stored data. Additionally, LLCD was to prove the integrity of laser technology to send not only error-free data but also uncorrupted commands and telemetry or monitoring messages to and from the spacecraft over the laser link.
- LLCD also demonstrated the ability to "hand-off" the laser connection from one ground station to another, just as a cellphone does a hand-off from one cell tower to another. An additional achievement was the ability to operate LLCD without using LADEE's radio at all. The project was able to program LADEE to awaken the LLCD space terminal and have it automatically point and communicate to the ground station at a specific time without radio commands. This demonstrates that this technology could serve as the primary communications system for future NASA missions.
• Dec. 11, 2013: LADEE completed its OLM-3 (Orbit Lowering Maneuver-3) on Nov. 10, and its OLM-4 on Nov. 20, 2013. During the commissioning phase when ground controllers were checking out the instruments and spacecraft, LADEE was orbiting the moon 250 km above the surface. Now LADEE is in its science operations orbit. All three science instruments are taking data in their planned sequence. LADEE's new orbit makes its closest approach to the moon's surface (or periapsis altitude) between 20 and 50 km, and farthest point (or apoapsis altitude) between 75 and 150 km. 42)
- The payload instrument measurements changed when LADEE swooped down to 50 km above the moon's surface after OLM-3 on Nov. 10. At that low vantage point, NMS (Neutral Mass Spectrometer) was able to detect Argon-40 for the first time, and see its distinctive variation across the lunar dawn. Argon-40, a noble gas, has an atomic mass 10 times that of helium, and tends to stay closer to the lunar surface. The rate that LDEX (Lunar Dust Experiment) was sensing lunar dust at high altitudes (approximately one dust grain per minute) suddenly increased several-fold at 50 km. And things got even better after OLM-4 brought LADEE into its science orbit.
• Nov. 22, 2013: LADEE has completed the commissioning phase, and now is ready to begin the mission's primary science phase. 43)
After the successful OLM-3 (Orbit Lowering Maneuver) on Nov. 10, LADEE was in an elliptic pre-science orbit. The first six days in this orbit were dedicated to completing the science instrument commissioning, doing opportunistic science measurements in coordination with NASA's LRO (Lunar Reconnaissance Orbiter) spacecraft, and taking measurements of the impact of the Leonids meteor shower on the lunar environment.
• On Nov. 20, 2013, the LADEE spacecraft successfully entered its planned orbit around the moon's equator — a unique position allowing the small probe to make frequent passes from lunar day to lunar night. This will provide a full scope of the changes and processes occurring within the moon's tenuous atmosphere. 44)
- LADEE now orbits the moon about every two hours in the altitude range of 12-60 km above the moon's surface. For about 100 days, the spacecraft will gather detailed information about the structure and composition of the thin lunar atmosphere and determine whether dust is being lofted into the lunar sky.
• On Oct. 26, 2013, ESA's OGS (Optical Ground Station) on the island of Tenerife has received laser signals of LLCD over a distance of ~400, 000 km from NASA's latest Moon orbiter, LADEE. The data were delivered many times faster (40 Mbit/s) than possible with traditional radio waves, marking a significant breakthrough in space communications. With the first two communication passes with LADEE on 26 October and six more to 29 October, the ESA team on Tenerife are tweaking the station hardware – especially for the uplink – and improving procedures. 45)
- The contact with Tenerife came just days after LADEE made history on October 18 in the first-ever laser transmission from lunar orbit, picked up by a NASA station at White Sands, New Mexico, USA. The craft is also transmitting to a third station, at NASA's Jet Propulsion Laboratory in California. - During the coming weeks, ESA engineers will test uplink communications at 20 Mbit/s and obtain accurate ‘time-of-travel' measurements to be used for calculating the spacecraft's orbit.
• On October 18, 2013, the LLCD (Lunar Laser Communication Demonstration) has made history using a pulsed laser beam to transmit data from lunar orbit to Earth at a record-breaking download rate of 622 Mbit/s. LLCD is NASA's first system for two-way communication using a laser instead of radio waves. It also has demonstrated an error-free data upload rate of 20 Mbit/s transmitted from the primary ground station in New Mexico to the spacecraft currently orbiting the moon. 46) 47) 48)
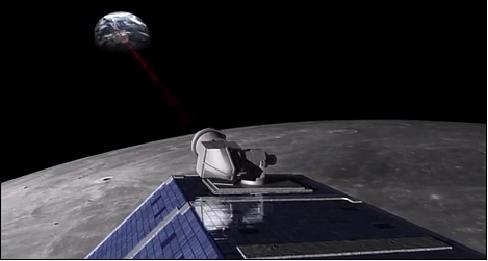

• Oct. 17, 2013: During the NASA shutdown period (general government shutdown Oct. 1-17, 2013), the LADEE mission continued to perform its critical maneuvers and capture into the commissioning orbit around the moon. The trajectory correction maneuver (TCM-1) was completed on Oct. 1, and set the spacecraft to rendezvous with the moon on Oct. 6. The Neutral mass Spectrometer (NMS) cap ejection on Oct. 3 was successful. The first LOI-1 (Lunar Orbit Insertion) maneuver (lasting ~4 minutes) on Oct. 6 was very accurate, and required no course adjustments afterward. This is an impressive performance of the propulsion system, given the size of the LOI-1 burn. The maneuver put the spacecraft into a 24 hour elliptical lunar orbit. 50)
- The LOI-2 maneuver on Oct. 9,2013 also was very accurate, putting LADEE into a 4-hour elliptic lunar orbit.
- The third and final LOI-3 burn occurred on Oct. 12, and put the spacecraft into the 2 hour commissioning orbit (roughly 235 km x 250 km). The LADEE spacecraft commissioning activities are now complete, and the instrument commissioning activities have begun.
- The LDEX (Lunar Dust Expreriment) and UVS (Ultraviolet Spectrometer) aliveness activities were completed successfully on Oct. 16, 2013 with both instrument covers deployed. These instrument cover deployments were the last remaining planned critical events for the mission. All critical maneuvers and all instrument cover deployments are completed at this point. The science instrument commissioning and lasercom primary experiment will be conducted through mid-November, at which point the spacecraft will start to drop down to the lower lunar science orbit.
• Sept. 25, 2013: The LADEE observatory continues the phase of the mission where it is cruising on its way to the moon. LADEE currently is in its third and final elliptic orbit around Earth – performing phasing loops. After the final perigee pass around Earth on Oct. 1, 2013, LADEE will travel to the point at which it will be captured around the moon using an initial LOI-1 (Lunar Orbit Insertion) burn of the onboard main engine. After that, LADEE will be in lunar orbit. 51)
- The major activities accomplished since the first perigree maneuver (PM-1) on Sept. 13, 2013, involved instrument checkouts with their covers closed. These tests ensure that the instruments are operational, and survived the stresses of launch. All three science instruments, as well as the laser communication experiment, successfully completed their tests and look healthy.
• Sept. 13, 2013: The LADEE observatory has completed the checkout phase of the mission, and is now in the cruise phase on the way to the moon. The project is currently in elliptic orbits around Earth, called phasing loops, and will continue with two more of these elliptical orbits until LADEE is captured around the moon using an initial LOI-1 (Lunar Orbit Insertion-1) burn on Oct. 6, 2013. After that the spacecraft is in lunar orbit. 52)
Sensor Complement
LADEE employs a high-heritage science instrument payload, including a neutral mass spectrometer, an ultraviolet spectrometer, and an in-situ dust sensor. In addition to these science instruments, LADEE will also carry a laser communications system technology demonstration.
NMS (Neutral Mass Spectrometer)
The NMS instrument is based on a similar instrument on NASA's CoNTour (Comet Nucleus Tour) mission with a launch on July 3, 2002 and the SAM (Sample Analysis at Mars) instrument developed for the Mars Science Laboratory (MSL). The NMS uses a high sensitivity quadrupole mass spectrometer with a 150 Dalton range and unit mass resolution. The NMS is a NASA/GSFC instrument. Participating organizations are: the University of Michigan/Space Physics Research Lab, Battel Engineering, AMU Engineering, and Nolan Engineering.
Although to date only He, Ar-40, K, Na and Rn-222 have been firmly identified in the lunar exosphere and arise from the solar wind (He), the lunar regolith (K and Na) and the lunar interior (Ar-40, Rn-222), upper limits have been set for a large number of other species. The LADEE NMS observations will determine the abundance of several species and substantially lower the present upper limits for many others. Additionally, LADEE NMS will observe the spatial distribution and temporal variability of species which condense at nighttime and show peak concentrations at the dawn terminator (e.g. Ar-40), possible episodic release from the lunar interior, and the results of sputtering or desorption processes from the regolith. 53)
Measurement concept:
• High-sensitivity quadrupole mass spectrometer, mass range 1-150 Dalton and unit mass resolution
• At 50 km lunar altitude or lower can detect helium, argon and other species
• UHV (Ultra High Vacuum) materials and processing used in the fabrication of NMS yield a substantial improvement over background instrument noise from Apollo era instruments, corresponding increase in sensitivity of the measurement.
• The sensitivity is necessary to adequately measure the low density atmosphere of the moon.
Performance data:
• Closed source species: He, Ar, non-reactive neutrals
• Open source species: neutrals and ions
• Mass range: 2 - 150 Dalton
• Mass resolution: unit mass resolution over entire range
• Sensitivity: 10-2 (counts per second) / (particles cm-3)
• Instrument mass: 11.3 kg, envelope: 43.2 cm x 24.5 cm x 37.0 cm, power: 34.4 W average, data rate: 3.5 kbit/s.
The elements of the LADEE NMS are shown in Figure 24. 54) Its ion dual ion source, hyperbolic quadrupole rod assembly, and vacuum housing consist of refurbished elements of the engineering unit NGIMS (Neutral Gas and Ion Mass Spectrometer ) developed for the CONTOUR mission. This mass spectrometer is a similar design to the Cassini INMS (Ion and Neutral Gas Mass Spectrometer) designed and developed at NASA Goddard as a facility instrument for this mission to the moons of Saturn.
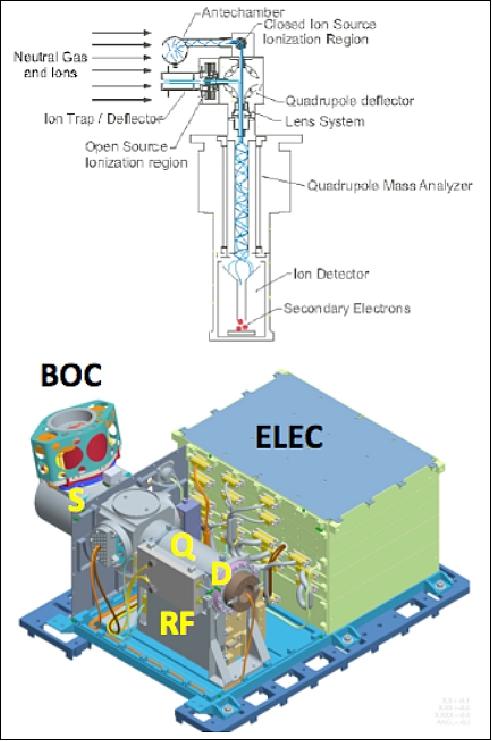
Legend to Figure 24: The top image shows the NMS elements. The bottom model shows BOC (Break-Off Cap), ELEC (Electronics box), S (Ion Source Region), Q (Quadrruple Region), D (Detector), RF (Radio Frequency).
With a new ion/electron optical design the sensitivity of the NMS has been improved over the INMS by more than an order of magnitude to optimize measurements in the low density lunar exosphere. The NMS closed source is suitable for species that do not adsorb on ion source surfaces such as He and Ar. A RAM density enhancement is realized in this source and the measurement of these inert noble gas species is not impacted by the multiple wall collisions in this source prior to ionization. On the other hand, the open source is required for a search for species such as metal atoms that are surface reactive. In this source the atomic or molecular species are ionized and focused into the quadrupole analyzer without wall collisions.
NMS calibration approach on the vacuum chamber: The NMS calibration will enable the response of the NMS to lunar gas to be established so that the detector counts measured in lunar orbit can be converted into ion source densities for the open source and into neutral flux into the closed source. The NMS was calibrated on a thermal gas ultra-high vacuum chamber pumped by magnetically levitated turbomolecular pumps. Both the chamber and the mass spectrometer were baked for several days prior to calibration. High purity N2 and the noble gases He, Ne, Ar, Kr, and Xe were utilized either as single gases or as mixtures. The flow out of the vacuum chamber was limited by an iris valve to minimize density gradients within the vacuum chamber. A NIST tracable Bayard Alpert gauge measured pressure. The mass spectrometer was operated with first with GSE (Ground Support Equipment) electronics to optimize lens focusing and to establish the response of the detector signal to variations in lens voltages and then moved to the NMS flight electronics.
NMS calibration after separation from the vacuum chamber: The NMS sensor incorporates a passive chemical getter that can remove the active gases. After the chamber calibration described in the preceding paragraph had been completed a mixture of noble gases He, Ar, Kr, and Xe was prepared and several static mode mass spectrometer scans carried out where the only pump utilized was the getter. The sensitivity of the closed source established for various noble gas isotopes from one of these runs is shown in Figure 25.
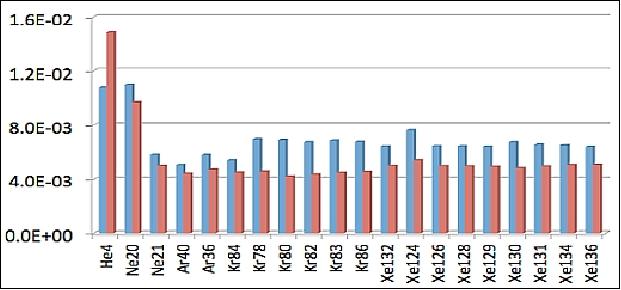
Legend to Figure 25: The sensitivity plotted on the vertical axis is in units of (counts/second)/(particle/cc) after normalization using ionization cross-sections to that of N2 to illustrate the differences between the low (21Ne through136Xe) and high frequency (4He through 20Ne) FR regions.
Early results from NMS observations: 55)
Owing to its very low chemical background at M/Z = 4, the NMS instrument was successful at detecting and map-ping exospheric Helium during the high altitude (250 km) commissioning phase. This early mapping campaign provided the unique opportunity to observe the variation of Helium at a constant altitude and for nearly a full lunation. Figure 26 shows results of Helium spatial and temporal variability as the Moon moves in and out of the Earth's magnetotail.
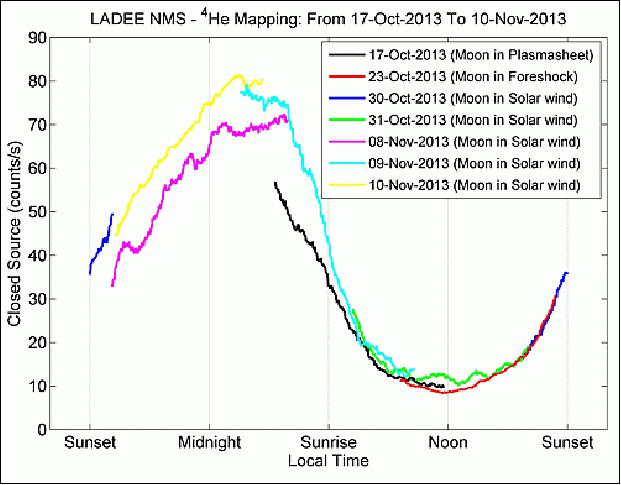
At the end of the commissioning phase and after the orbit's periselene was lowered to ~ 50 km, the NMS instrument was able to detect Argon (M/Z = 40) and Neon (M/Z = 20). Mapping of the temporal and spatial variation of these two species along LADEE's orbit is being conducted systematically.

UVS (Ultraviolet/Visible Spectrometer)
The UVS instrument is a next-generation, high-reliability version of the LCROSS UV-VIS spectrometer, spanning 230-810 nm wavelength, with high (<1 nm) spectral resolution. UVS will also perform dust occultation measurements via a solar viewer optics system. The objective is to examine exospheric emissions and a broader continuum of forward or backward scattered light from dust down to ~10 nm in size. In occultation mode, UVS will study dust distributions down to ~300 m above the surface. The UVS is a NASA/ARC instrument. Participating organizations are: Aurora Design & Technology, and Visioneering, LLC. 56) 57)
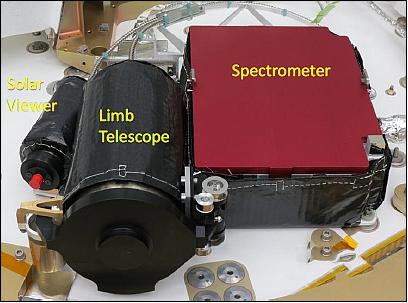
LADEE mission measurement concept:
• UVS includes UV-VIS spectrometer, telescope, solar diffuser, & bifurcated optical fiber
• UVS observations consists of limb and occultation measurements
• Limb observations measure the lunar atmosphere, & also measure limb dust by measuring back- or forward-scattered sunlight
• Solar occultation observations measure lunar atmospheric dust extinction from 0 to 50 km.
Performance data:
• In Limb mode measures atmospheric species including: K, Na, Al, Si, Ca, Li, OH, H2O
• By combining long integration times, UVS measures each specie to < current upper limits
• In limb mode measures dust (via scatter) at concentrations as low as 10-4 per cc for r=100 nm size particles
• In occultation mode, UVS measures dust (via extinction) at concentrations as low as 10-4 per cc for r=100 nm size particles down 300 m in altitude.
• The UVS instrument has a mass of 3.98 kg and an average power consumption of 1$ W.

UVS has two means of observing: a Limb Telescope and a SOV (Solar Occultation Viewer). The Limb Telescope is pointed just above the surface of the moon, at its limb, looking for emission by exosphere gasses and the scattering of sunlight by dust grains. For a typical orbit, limb observation will be centered on the morning and evening terminators and local noon. During each of these activities the LADEE spacecraft will keep the telescope pointed no more than 20 km above the lunar surface. Each limb "stare" is accompanied by a "nod" during which the telescope field of view is nodded down to below the lunar limb,so that the moon is entirely in the FOV (Field of View) of the telescope, and then up to an altitude of 50 km. The "nod" will help to resolve any altitude variations in gasses or dust and provides a calibration point for zodiacal light and light scattered into the telescope from the surface of the moon.
The SOV allows UVS to stare at the sun, monitoring it as it rises or sets across the lunar limb. The advantage of a solar occultation observation is the very high signal-to-noise achieved (SNR>500) in the instrument for very short (<20 ms) integration times. The high SNR and rapid sample rate allows UVS to search for extinction due to dust at very low altitudes above the lunar surface (<1.5 km).
Observations to Date: 58)
UVS has two means of observing: a "Limb Telescope" and a "Solar Occultation Viewer". Limb observations, using the UVS three-inch telescope, have been made on a routine basis, with limb "stares" at 20 km at the terminators, and 40 km at around local noon time. At the terminators the spacecraft "nods" the telescope between the surface and about 50 km. At noon it was found that near-surface scatter precluded observations below about 30 km, so nods are not performed then. There have been a mix of both "backward" looks (stares that point in the anti-velocity direction of the spacecraft), and "forward" looks (which flip the spacecraft to allow UVS to look in the velocity direction). This permits observations both in and away from the direction of the sun.
Occultations have been performed about once every two days, tracking the sun as it sets behind the sunrise terminator between about 35 km and the surface. In this mode UVS has gathered spectra at a rate of either 15 ms or 26 ms, corresponding to a spatial sampling of <1 km with very high SNR (typically >500 for a single scan).
Sodium and potassium are regularly measured in all activities, except for occultations. Trends in these measurements are made both spatially and temporally, and associations are with specific events, such as meteor streams, and surface composition are examined.
During terminator observations the spacecraft observes the illuminated lunar atmosphere while the spacecraft is in lunar umbra. At these times lunar surface and solar scatter is absent, thus very faint emissions or scattering signatures can be searched for in the data.
LDEX (Lunar Dust Experiment)
LDEX is designed to map the spatial and temporal variability of the dust size and density distributions in the lunar environment. LDEX is an impact ionization dust detector, sensing dust impacts in situ at LADEE orbital altitudes of 50 km and below, with a particle size range of between 100 nm and 5 µm. Dust particle impacts on a large hemispherical target create electron and ion pairs. The latter are focused and accelerated in an electric field and detected at a microchannel plate. The detector area of LDEX is ~ 0.01 m2. 59) 60) 61)
LDEX has been designed and developed at LASP (Laboratory for Atmospheric and Space Physics) of the University of Colorado, Boulder, CO. Participating organizations are: Max-Planck-Institute for Nuclear Physics, Heidelberg, Germany; University of Stuttgart, and the Institute for Geosciences, Heidelberg University, Heidelberg, Germany.
• LDEX measures the mass of individual dust grains with m ≥ 1.7 x 10-16 kg (radius rg ≥ 0.3 µm) for impact speeds ~ 1.7 km/s
• Also measures the collective current due to grains below the threshold for individual detection, enabling the search for dust grains with rg ~ 0.1 µm over the terminators.
LDEX has a mass of 3.6 kg (with margin), size: 15 cm x 15 cm x 20 cm, power of 6.11 W (peak) and 3.8 W in operations, the data rate is 1 kbit/s (64 Mbit/day).

Of particular interest is to verify from orbit the presence of water ice in the permanently shadowed lunar craters.

Legend to Figure 31: The target hemisphere is segmented to accommodate impact detection to determine the mass and speed of dust grains (top segment) and a time-of-flight setup for chemical analysis (bottom segment).
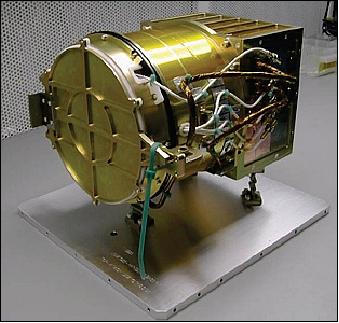
LDEX aboard the LADEE spacecraft can collect a large number of samples from a greater part of the entire surface for analysis. It thus combines the advantages of a remote sensing instrument and a lander. The instrument is especially sensitive to the metallic compounds of minerals and any species which easily form ions (e.g. water).
Initial LDEX observations: 62)
The LDEX cover was deployed on Oct. 16, 2013 after a thorough testing of all functionalities of the instrument. Since the cover deployment, LDEX continues to make dust observations. By the end of 2013 LDEX had recorded about 65,000 MCP and target waveform pairs, indicating potential dust impacts. The unambiguous identification of dust impacts is ongoing, based on the analysis of the individual waveforms. The major milestones of LDEX operations are summarized in Figure 2.

Legend to Figure 33: The number of recorded events (noise and dust impacts) sharply increased following LADEE orbit lowering maneuvers. An unusually large burst of events was observed on Nov. 12, 2013, most likely related to the Taurids meteor shower. The following intense period starting on Dec. 12, 2013 coincides with the Geminids shower and the landing of Chang'e-3.
LDEX was tested and calibrated at the dust accelerator facilities of the University of Colorado, and MPI-K, Heidelberg, Germany. The facilities are capable of providing particles with typical radii of 0.1 to 2 µm with speeds >> 10 km/s.
The LDEX observations are the first to identify the ejecta clouds around the Moon sustained by the continual bombardment of interplanetary dust particles. Ejecta clouds were first observed by the Galileo mission during flybys of the icy moons of Jupiter: Europa, Ganymede, and Callisto. No ejecta clouds were directly identified as of yet by the Cosmic Dust Analyzer onboard Cassini in orbit around Saturn.
However, modeling the observations indicate that ejecta particles have to make a non-negligible contribution to the dust environment, in addition to the active plumes on Enceladus. Most of the dust particles generated in impacts have insufficient energy to escape and follow ballistic orbits, returning to the surface, ‘gardening' the regolith. Ejecta clouds are expected to engulf all airless planetary objects, including the Moon, Mercury, and the moons of Mars: Phobos and Deimos. LDEX is expected to characterize in detail the ejecta cloud surrounding the Moon, including the size, velocity, and angular distribution of the ejecta particles.
LDEX also continues to search for plasma-lofted particles. These particles, with characteristic radii of ~ 0.1 µm, have been suggested to lift off the surface due to charging and intense localized electric fields. The processes involved remain controversial. The combination of the remote sensing optical observation by the LADEE UVS (Ultraviolet and Visible Spectrometer) and the in situ LDEX instruments will establish new strict upper limits for the density of these particles.
The ARTEMIS (Acceleration, Reconnection, Turbulence, & Electrodynamics of Moon's Interaction with the Sun) mission of NASA consists of two probes from the THEMIS mission, repurposed in 2011 to perform plasma science around the Moon. ARTEMIS is able to provide measurements of the lunar charged particle environment as well electric and magnetic fields during the entire LADEE mission and provide some insight into the LDEX current measurements. 63) 64)
ARTEMIS provides a variety of plasma measurements which show a high degree of correlation with LDEX current measurements. There are two regions in the LDEX data that show consistent correlation: 1) the subsolar point and 2) the time LADEE crosses into umbral shadow. Shown in Figure 34 are the average LDEX current for 15 minutes from subsolar and shadow crossing time respectively. Additionally, the component of the convection electric field parallel to the LDEX boresight appears to be significant in LDEX's current measurements.
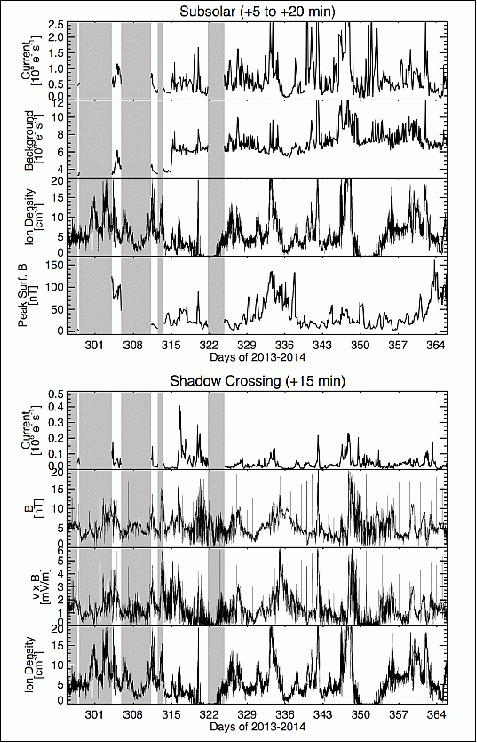
LLCD (Lunar Laser Communications Demonstration)
LLCD is a collaborative project of MIT/LL (Massachusetts Institute of Technology/Lincoln Laboratory) and NASA/GSFC. The goal is to demonstrate and validate duplex optical communications from a lunar orbiting spacecraft to an Earth-based ground receiver. The requirements call for data rates of up to 620 Mbit/s in downlink and up to 20 Mbit/s in uplink. In addition, two-way time-of-flight measurements are needed with the potential to perform ranging with sub-centimeter accuracy. 65) 66) 67) 68) 69) 70) 71) 72) 73) 74)
LLCD is a secondary payload on the LADEE mission. LLCD will demonstrate:
• Photon counting pulse position modulation
• Inertial stabilization
• Integrating an optical communications terminal to a spacecraft
• Link operations from lunar orbit
• Scalable array ground receiver.
Optical communications offers many potential benefits for future deep space missions. Increased aperture gains and reduced diffraction losses at optical wavelengths, as compared to radio-frequency (RF) wavelengths, enable higher data rate forward and return links with reduced size, weight, and power (SWAP) burden on a spacecraft and smaller ground terminals on the Earth. Moreover, the 10's of THz of available spectrum in the optical bands is unregulated, and, commercially-available optical transmitter and receiver technologies provide for efficient utilization of >40 GHz of optical bandwidth, enabling higher data rate links that are much more photon efficient than those that might be achieved using the narrower bandwidths available for RF links. For these reasons, NASA has identified optical communications as an important technology for the future of deep space communications. The LLCD (Lunar Laser Communications Demonstration) on LADEE is intended to be a first step toward developing an operational optical communications capability for future manned and robotic missions.
The LLCD comprises 3-elements:
• LLST (Lunar Lasercom Space Terminal)
• LLGT (Lunar Lasercom Ground Terminal)
• LLOC (Lunar Lasercom Operations Center).
Each of these elements is being designed, built, tested, and operated by MIT Lincoln Laboratory. The LLCD project is being managed by NASA's Goddard Space Flight Center. The LLST payload will be operated for a total of 16 days during the 1 month commissioning phase which precedes the science phase. During the commissioning phase, the spacecraft will be in a ~2 hour orbit at an altitude of approximately 250 km above the lunar surface. Power limitations and thermal considerations on the spacecraft limit the duration of LLST operations to about 15 minutes per orbital pass. Since the ground terminal must be in view for communications operations to take place, each day will provide opportunities for 3-5 laser communications orbits (Ref. 65).
LLST (Lunar Lasercom Space Terminal)
The LLST instrumentation is comprised of three modules (Figure 35): an optical module, a modem module, and a controller electronics module. The optical module is situated on the exterior of the LADEE spacecraft payload module, while the modem and controller electronics modules are mounted on the interior of the spacecraft. The LLST payload has a mass of ~30 kg and operates at a power in the range of ~50-140 W.
The optical module is a Cassegrain telescope with a 10.8 cm aperture on a two-axis gimbal that enables optical link operation over a wide range of spacecraft orientations. The telescope and backend optics are inertially stabilized using MIRU (Magnetohydrodynamic Inertial Reference Unit) that rejects high-frequency disturbances from the spacecraft interface (MIRU was built by the ATA (Applied Technology Associates) Corporation of Albuquerque, NM). A wide field-of-view InGaAs quadrant detector is used for spatial acquisition and coarse tracking of the optical uplink. Transmit and receive signals are coupled to and from the telescope via optical fibers. These fibers are mounted to piezoelectric actuators that are used to provide a point-ahead capability between the transmit and receive fibers and low-bandwidth fine spatial tracking of the optical uplink signal.

The optical transmitter and receiver are contained in the modem module, which is fiber coupled to the optical module. Digital electronics in the modem aggregate the various downlink data sources (including science data from the LADEE spacecraft, high-rate LLST telemetry, and loopback of the optical uplink) and encode the data using a high-efficiency half-rate code. The encoded data are modulated onto an optical carrier using a high-bandwidth pulse-position modulation format (slot rates up to 5 GHz) and amplified to 0.5 W average power in an EDFA (Erbium-Doped Fiber Amplifier) prior to transmission on the downlink. An optically preamplified direct-detection receiver based on a low-noise EDFA is used for the uplink receiver. A near-optimum hard-decision pulse-position modulation demodulator based on a previously demonstrated binary PPM (Pulse Position Modular) demodulator6 demodulates the uplink waveform prior to decoding of the uplink signals in a high-density SRAM-based field programmable gate array.
The LLST is a three wavelength system, operating in the 1550 nm band, consisting of one downlink communications wavelength, the transmit wavelength, and two uplink wavelength, the acquisition wavelength and the receive communications wavelength. The three wavelengths are separated by ~20 nm in total. Dichroic optics are used to route and separate the beams within the SOA (Small Optics Assembly). On the outgoing transmit path, the beam exiting the transmit fiber is collimated, apertured, and folded into the telescope via a fold mirror and Dichroic A (Figure 36). On the incoming acquisition path, Dichroic A transmits, Dichroic B reflects, and the acquisition fold mirror directs the beam into the acquisition quadrant cell. On the incoming receive path, the two dichroics pass the receive beam and the aspheric focuses the beam into the receive fiber. The reflectivity of the two dichroics is plotted in Figure 37.
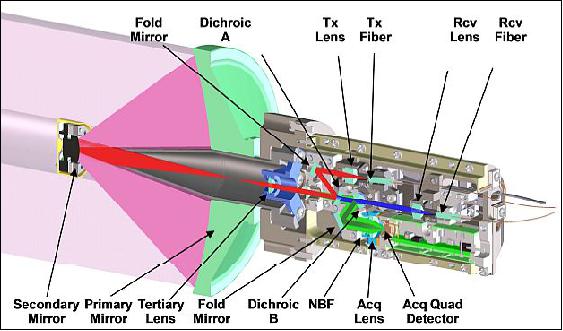

The telescope magnification of 47x support the implementation of commercially available aspheric lenses for the entire OA (Optical Assembly), allowing for a compact SOA. All lenses, the two fiber lenses, the acquisition lens, and the telescope tertiary are inexpensive anti-reflection coated molded aspheres. From the lens lots purchased, the lenses with the best on-axis wavefront performance are selected for the LLST. Additional material screening was performed for optical transmission and radiation resistance at 1550 nm.
The two communications paths, downlink transmit and uplink receive, require excellent on axis wavefront performance. On the downlink transmit path, high wavefront performance is required to maximize the energy density in the far-field at the Earth's ground stations. On the uplink receive path, high quality wavefront performance is required to maximize the coupling into the receive fiber. The acquisition path, however has somewhat different requirements. The acquisition quadrant cell has a coarse FOV of ± 1mrad and a smaller linear FOV of approximately ± 30 µrad . Outside the linear region of the quadrant detector, the quality of the beam at the detector is not required to be perfect. This allows for a relaxation of the off-axis wavefront requirements for the telescope.
The controller electronics module, a custom avionics module based on a single-board computer, provides closed-loop control of the various actuators in the optical module. It also provides command and telemetry interfaces for the LLST payload to the LADEE spacecraft and configures and controls the modem.

Overview of the modem module (Ref. 66): The modem design (Figure 39) consists of four vertically-stacked slices: the power, digital, analog and EO (Electro-Optic) slices. The advantage of the modular approach is that each slice can be built and tested in parallel which reduces integration and test time of the flight modem assembly and allows for design flexibility to address evolving requirements as the spacecraft design matures. All four slices are connected with external electrical cables and the transmit and receive fibers are run from the EO slice to the optical module. The total mass of the modem is ~10.96 kg and it occupies a volume of ~26 liter. The modem consumes ~78 W during normal operations.
For the optical downlink, the modem aggregates the various data sources on the spacecraft, encodes, interleaves and frames the data, modulates the data onto the downlink optical carrier and amplifies the optical signal prior to transmission. There are three sources of data for the downlink: high-rate LLST telemetry from the controller electronics (up to 5 Mbit/s), data received on the optical uplink (up to 20 Mbit/s), and science data from the LADEE spacecraft (up to 40 Mbit/s). The data source aggregation and downlink encoding is performed in an SRAM-based field programmable gate array (FPGA) in the modem digital slice.
The encoder consists of a ½-rate serially concatenated PPM (Pulse Position Modulation) turbo code which is computed using a simple convolutional encoder and performs within 1.5 dB of the theoretical channel capacity. PPM is used for the downlink because it is orthogonal with high power efficiency in the transmitter and is well-matched to the superconducting photon counting detectors in the ground terminal.
The energy efficiency of the optical downlink is -4.6 dB detected photons/bit or 25-100 µJ/bit at channel capacity and before modulation of the data, interleaving is used to mitigate the atmospheric effects on the downlink channel. The specific modulation used is a 16-ary PPM format with achievable user data rates from 39 – 620 Mbit/s which corresponds to PPM slot rates of 0.311 – 5 GHz. The wide range of available data rates enables demonstration of the optical link in a wide range of atmospheric conditions.
The 620 Mbit/s link has been specified to offer up to 16 multiplexed subchannels, where each individual subchannel provides a data rate of 38.55Mbit/s, resulting in a maximum aggregate data rate of roughly 620 Mbit/s. In order to achieve high link reliability, the proposed link is based on NASA's capacity-approaching modulation and coding scheme, which comprises a serial concatenation of an inner accumulate PPM (Pulse Position Modulation) and an outer convolutional code SCPPM (Serially Concatenated PPM). 75)
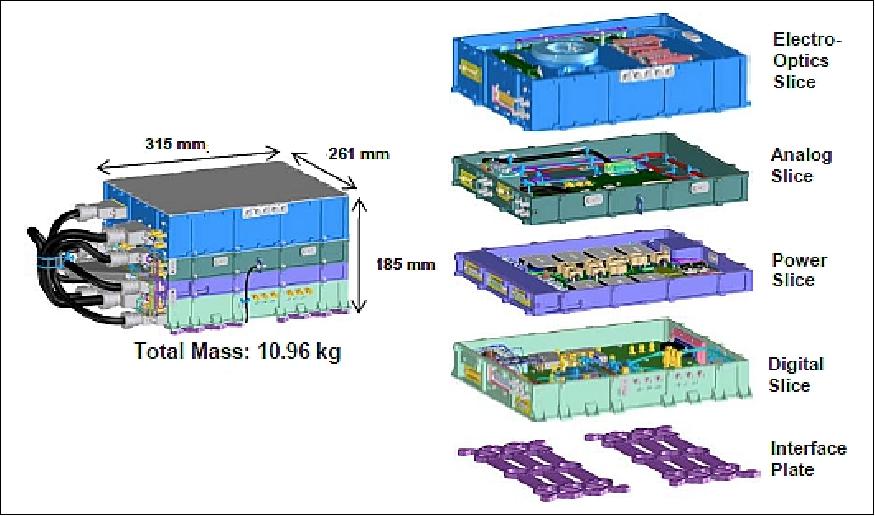
The modulation in the downlink occurs in the EO slice which utilizes a MOPA (Master Oscillator Power Amplifier) architecture. The master oscillator consists of a continuous wave DFB laser at ~ 1550 nm and the electrical to optical data conversion occurs in a LiNbO3 modulator. The optical amplification of the downlink data to a 0.5 W average power is achieved with a double-pass two-stage polarization-maintaining EDFA design. Each gain stage is pumped with two external grating stabilized 976 nm pump lasers. To optimize the power efficiency of the modem pairs of pump lasers are connected electrically in series.
Modem uplink performance: The uplink receiver is an optically pre-amplified direct detection receiver, as shown in Figure 40. The design consists of a two-stage polarization insensitive EDFA for high gain (> 40 dB) and low noise figure (< 4 dB) performance.

The expected average optical powers at the fiber input to the receiver range from -58 to -78 dBm. After the second gain stage a narrow-band 10 GHz FBG (Fiber Bragg Grating) optical filter is used to reduce spontaneous-emission noise in the signal incident on the tracking and communications photodetectors. Because of the 40ºC temperature swing in the modem, the peak wavelength shift in the FBG's is expected to be ~ ± 20 GHz and this assumes a Δλ/ΔT of ~ 1 GHz for the FBG's. Because of this large peak wavelength shift, temperature stabilization in the FBG's is required.
Space Terminal | – 10.8 cm aperture, 0.5 W, 1.55 µm |
Ground Terminal | – Downlink Receiver |
LLST mass, power | 32.8 kg (with margin), 136.5 W |
LLGT (Lunar Lasercom Ground Terminal)
The LLGT (Figure 41) consists of an array of transceiver and receiver telescopes and a control room. The telescope arrays are used to demonstrate a scalable and cost-effective approach for providing large-aperture transmitters and receivers. They also provide spatial diversity which helps mitigate the deleterious effects of atmospheric turbulence on the optical uplink and downlink signals.
Four 15 cm refractive telescopes are used for the optical uplink and four 40 cm reflective telescopes are used as collectors for the optical downlink. Each telescope is fiber-coupled to the control room where the optical transmitters and receivers reside. All 8 telescopes are mounted on a single elevation over an azimuth gimbal. The gimbal provides coarse pointing for all of the telescopes with near-hemispherical coverage. The back-end optics for each telescope include a focal plane array and a fast-steering mirror for high-bandwidth tracking of the optical downlink and correction of any relative pointing biases between the telescopes on the gimbal. While mounting all of the telescopes on a single gimbal is not required for the arrayed transmitter/receiver concept, it simplifies the process of time-aligning the transmitted and received waveforms over the range of pointing required to support the optical links. The telescopes are housed in a fiberglass environmental enclosure which maintains a suitable environment for their operation.

The control room houses all of the electronics to control the gimbal and telescopes as well as the ground terminal modem electronics and optics. Four EDFA-based 10 W optical transmitters generate the pulse-position modulated signals for the optical uplink. Each transmitter is fiber coupled to one of the transceiver telescopes via a polarization-maintaining single-mode fiber.
The wavelengths of each of the transmitters are slightly detuned to allow for low-power-penalty non-coherent combining of the signals at the space-terminal receiver. The optical downlink receiver is based on photon-counting superconducting nanowire arrays. These detectors operate at cryogenic temperatures to provide very high photon detection efficiencies, and have been previously used to demonstrate high data rate optical communications with receiver efficiencies exceeding 1 bit / detected photon.
In order to achieve good coupling efficiency in the telescopes in the presence of atmospheric turbulence while preserving the polarization of the downlink signal, a custom multi-mode polarization-maintaining fiber is used to couple the receiver telescopes to the SNDAs. Custom FPGA-based digital electronics in the control room interface to the various data sources and destinations, generate the waveforms for the optical uplink, and demodulate and decode the detected signal on the optical downlink.
Ground Segment
The LADEE mission will utilize the MOC (Mission Operations Center) located at NASA/ARC ( Ames Research Center) in Moffett Field, California. The project will use the SOC (Science Operations Center) located at Goddard Space Flight Center (GSFC) in Greenbelt, MD. The primary ground station, the transportable LLGT (Lunar Lasercom Ground Terminal), will be located at the WSC (White Sands Complex) near Las Cruces, New Mexico.
The data from the ground station(s) will be routed to the MOC at ARC for processing, distribution and data storage/archiving. Science and instrument data, along with processed spacecraft health and safety data, will be transmitted to the GSFC SOC. The SOC will perform instrument data processing and scientific analysis of instrument data. Figure 42 shows a schematic representation of the mission system architecture and command and data flow within it (Ref. 15).
In order to increase the amount of time of operations in the face of the short LLCD mission (one month) and the possibility of clouds, the program also included two alternate terminals, the LLOT (Lunar Lasercom OCTL Terminal), residing at JPL's OCTL (Optical Communications Telescope Laboratory) at the Table Mountain Facility in California, and the LLOPS (Lunar Lasercom Optical Ground System, residing at ESA's OGS on Tenerife, the Canary Islands (Ref. 71).



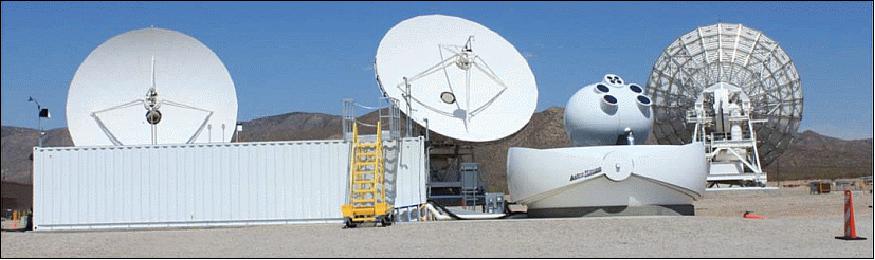
LLOC (Lunar Lasercom Operations Center): The signal, command, and telemetry flow from/to the LLOC is shown in Figure 46. The LLOC had a direct duplex data connection from the LLGT facility at White Sands to enable high rate (optical) data forwarding and reception. There was also a lower rate connection to the SOC (Science Operations Center) at NASA/GSFC through which LADEE spacecraft telemetry was received and LLST commands/telemetry were sent/received. Nearly full command and control of the LLGT was possible from the LLOC. Real-time telemetry from all three ground terminals was processed and displayed in the LLOC (Ref. 73).
Verbal information channels called "voice loops" were used in the LLOC to communicate real-time by voice to/from the MOC (Mission Operations Center), SOC, and all three ground terminals. All telemetry that came into the LLOC as well as all commands that went out of the LLOC were archived.

Ground Terminal Receiver
Tight size/weight/power constraints on the satellite payload (the average optical transmit power is < 1 W), in addition to the large R2 link loss due to the approximately 400 thousand kilometer separation between the earthbound ground terminal and lunar satellite, necessitate efficient usage of the optical signal appearing at the ground terminal receiver. Some constraints on the collection optics for LLCD are that: 77)
1) they be simple and relatively compact and transportable to support relocation on short notice — this feature initially provided flexibility in postponing the final ground terminal site selection in order to optimize mission link availability depending on the expected seasonal cloud coverage at launch time.
2) they mitigate communications performance degradation on days with strong atmospheric turbulence.
The receiver collection optics consist of four 40 cm ∅ telescopes mounted to a gimbal and aligned to a common boresight; the use of multiple telescopes provides spatial diversity to reduce the severity of atmospheric-induced fades; the use of a common, fixed bore-sight eliminates the need for dynamic, orientation-dependent deskew and synchronization procedures to time align signals across the telescope array. A clamshell enclosure protects these telescopes from the weather during periods of inactivity.

Light from each telescope is guided by a weakly polarization-maintaining multimode optical fiber (MMPMF), and lens coupled onto a 14 µm diameter array of four interleaved superconducting nanowire single-photon detectors (SNSPD) — this passive and relatively simple multimode scheme maintains high coupling efficiency in the presence of strong turbulence without the need for adaptive optics. Each of the total sixteen detector elements is independently biased and cooled to 2.6 K inside a closed-cycle cryostat to achieve detection efficiencies of ~60% per nanowire as measured from the input to the MMPMF.
Fast reset times (~15 ns) and small timing jitter (~60 ps FWHM) allow the SNSPD-based photon counting receiver to achieve high count rates with relatively few detector elements when compared to other technologies with similar detection efficiency. The nanowire geometry seen in Figure 47, which resembles a wire grid, results in a 3-4 dB reduction in detection efficiency for fields polarized perpendicular to the wires; thus, polarization alignment between the multimode fiber and detector sample is required.
The output electrical pulses from the detectors are conditioned first by cryogenic GaAs HEMT amplifiers packaged in proximity to the detectors on the sample mount at 2.6 K, and then subsequently by two additional stages of amplification at room temperature, outside of the refrigerator. The amplified waveforms pass through a one bit analog-to-digital converter (i.e., comparator) whose discrimination threshold is determined empirically to maximize SNR.
External Optical Signal Reception
ESA (European Space Agency) modified its OGS (Optical Ground Station) equipment to participate in the LLCD (Lunar Laser Communications Demonstration) experiments. The OGS is located at the Observatorio del Teide (OT) on Tenerife island, Spain. The OGS has been used to commission and test laser communication terminals, such as those on the ARTEMIS, OICETS, TerraSAR-X and NFIRE satellites. It is now being prepared to test the LCTs onboard the Alphasat, EDRS-A and EDRS-C satellites. 78)
Range | Lunar distance | 362,570 - 405,410 km |
Wavelength | Downlink | 1550.12 ± 0.1 nm |
Modulation | Downlink | 16 PPM (Pulse Position Modulation) |
Data rate | Downlink (up to for GCT) | 155 Mbit/s |
Downlink irradiance | At border of atmosphere | 0.17 - 1.7 nW/m2 |
Uplink irradiance | At LADEE spacecraft | 36 - 63 nW/m2 |
ESA's OGS will also use the laser to communicate with NASA's LADEE spacecraft orbiting the moon. ESA's OGS is being upgraded with a complementary unit and, together with two US ground terminals, will relay data at unprecedented rates using infrared light beams at a wavelength similar to that used in fiber-optic cables on Earth. 79)
The testing took place in July 2013 at a Zurich, Switzerland, facility owned by ESA's industrial partner RUAG and made use of a new detector and decoding system, a ranging system and a transmitter.
A NASA team, supported by the MIT/LL (Massachusetts Institute of Technology/ Lincoln Laboratory) and the Jet Propulsion Laboratory, brought over their laser terminal simulator, while ESA together with RUAG and Axcon of Denmark set up the European equipment to test compatibility between the two sets of hardware.
The first laser link-up with LADEE is expected to be attempted four weeks after launch, around mid-October 2013.


Legend to Figure 49: ESA's OGS is 2400 m above sea level on the volcanic island of Tenerife. Visible green laser beams are used for stabilizing the sending and receiving telescopes on the two islands. The invisible infrared single photons used for quantum teleportation are sent from the neighbouring island La Palma and received by the 1 m telescope located under the dome of the OGS. Initial experiments with entangled photons were performed in 2007, but teleportation of quantum states could only be achieved in 2012 by improving the performance of the setup.
Aside from inter-island experiments for quantum communication and teleportation, the OGS is also used for standard laser communication with satellites, for observations of space debris or for finding new asteroids. The picture is a multiple exposure also including Tenerife's Teide volcano (elevation of 3718 m) and the Milky Way in the background.
OGS Transmitter Design
The transmitter design is bi-static and was driven by the assumption that only a geometrical separation between the transmitters and the receiver could prevent cross-talk. It was anticipated that with the extremely high dynamic range of >100 dB between the transmitted and received signals it would not be possible to develop a monostatic design. However, the OCTL design from JPL demonstrated that a monostatic design is feasible. 80)
As shown in Figure 50 three individual transmit apertures with 40 mm diameter are arranged around the 1 m telescope receive aperture. One of the three transmit apertures is covered by a large aperture (120 mm diameter) corner cube retroreflector (CCRR), which send the transmitted light back into the receiver for alignment purposes. This alignment procedure is performed for each of the three transmitters after which the CCRR is removed.

Legend to Figure 50: The "cap" on one of the three transmitters is a CCRR (Corner-Cube Retro-Reflector) used to align the transmit beam direction of each of the three transmitters with the receiver. The CCRR is removed after alignment.
The three transmitter apertures are driven from three fiber amplifiers (3SPhotonics/Manlight) producing 40 W of optical output power each. The fiber amplifiers, attached to the side of the telescope tube, are driven from six seed lasers, three for acquisition and three for communication purposes. The communication lasers are followed by data modulators (Photline).
Three transmitters are used to:
• Increase the transmit power, because the most powerful commercial fiber amplifier delivers 40 W average power max.
• Decrease the atmospherically induced fading (scintillation) of the uplink beam. The three transmit beams are geometrically separated and therefore pass different sections of the atmosphere. The speckle pattern created in the far field is therefore different for each beam. The fact that the wavelength of each beam is slightly different prevents interference and generates a more homogeneous far-field pattern.
1) Acquisition laser system
For detection by the LLST (Lunar Lasercom Space Terminal), the acquisition signal requires a 50% duty cycle intensity modulation at 1 kHz. The initial design implemented was to drive the seed laser current with a corresponding modulation signal.
This approach failed for two reasons:
• The low frequency of the seed laser modulation current caused a laser diode temperature modulation, which lead to laser line-width spreading beyond the spectral width of the LLST input filter.
• The OGS fiber amplifiers were not able to cope with 1 kHz amplitude modulated input signal. As a matter of fact the internal optical isolator of one fiber amplifier (3SPhotonics/Manlight) was destroyed during testing with 1 kHz input modulation.
The solution found was to modulate the pump diodes of the third (and last) amplification stage of the fiber amplifiers with a 1 kHz modulation signal. This caused the modulation extinction ratio to drop to 10 dB (3 dB below specification) but proved sufficient to acquire the LLST. The company 3SPhotonics/Manlight implemented this additional feature into all three amplifiers in a very short time and the laser amplifier output power versus the drive current is shown in Figure 51.

Legend to Figure 51: The blue curve represents the unmodulated output power, the red curve the 1kHz modulated output power and the green curve is a multiplication of the red curve by two. Modulation more than halves the output power due to ringing.
The link margin for the acquisition uplink signal is given in Table 3. The three transmitters emit 40 W each, but the modulation penalty is taken into account. The scanning pattern loss only applies during initial acquisition because the OGS scans its transmit beams such that the irradiance drop in the far-field pattern does not exceed 3dB.
Parameter | Initial Acquisition Value | Stable Tracking Value |
Tx average power (3 x 40 W) | 50.8 dBm | 50.8 dBm |
Tx modulation penalty caused by the 50% duty cycle, 1 kHz intensity modulation (Figure 51) | -3.5 dB | -3.5 dB |
Tx antenna gain (40 mm 1/e2 diameter) | 98.1 dB | 98.1 dB |
Tx transmission loss | -0.4 dB | -0.4 dB |
Tx Strehl loss | -1.3 dB | -1.3 dB |
Atmospheric transmission loss | -1.5 dB | -1.5 dB |
Scanning pattern loss (6 arcsec step width) | -3.0 dB | 0.0 dB |
Free space loss (400,000 km) | -310.1 dB | -310.1 dB |
Rx antenna gain per unit area | 127.1 dB/m2 | 127.1 dB/m2 |
Irradiance at LLST | -43.8 dB/m2 | -43.8 dB/m2 |
Required acquisition signal irradiance | -54.4 dB/m2 | -44.4 dB/m2 |
Uplink acquisition margin | 10.6 dB | 3.6 dB |
2) Communication laser system
As the uplink modulation format for communication is 4-PPM with a pulse width of 3.2 ns, the pulse delay through the three transmitters must be identical to fractions of a nanosecond such that all three transmitters operate simultaneously.
As it turned out the largest contributor to the differential pulse delay through the system was the internal active fiber lengths of the three fiber amplifiers. Using fast photo-detectors and an oscilloscope it was possible to measure the individual pulse delay and up to 6 m in cable length difference were measured. This delay was then compensated by electrically driving the three laser modulators using coax-cables of different length. The individual components of the transmitter systems together with a block diagram are shown in Figure 52.The link margin for the communication uplink signal is given in Table 4. 81) 82)

Transmitted average power (3 x 40 W) | 50.8 dBm |
Tx antenna gain (40 mm, 1/e2 diameter) | 98.1 dB |
Tx transmission loss (0.91) | -0.4 dB |
Tx Strehl loss | -1.3 dB |
Atmospheric transmission loss (0.71) | -1.5 dB |
Free space loss (400,000 km) | -310.1 dB |
Rx antenna gain per unit area | 127.1 dB/m2 |
Irradiance at LLST | -37.3 dB/m2 |
Required communications irradiance at LLST | -42.0 dB/m2 |
Uplink communication margin | 4.7 dB |
OGS Receiver Design
Due to the weak signal irradiance from LLST (Lunar Lasercom Space Terminal) in lunar distance, the OGS optical receiver design is driven by the need to minimize transmission losses (Ref.80) . The receiver system is therefore installed in the Cassegrain focus, where the least number of optical elements in the telescope are present (2 reflective surfaces, namely primary and secondary mirror).
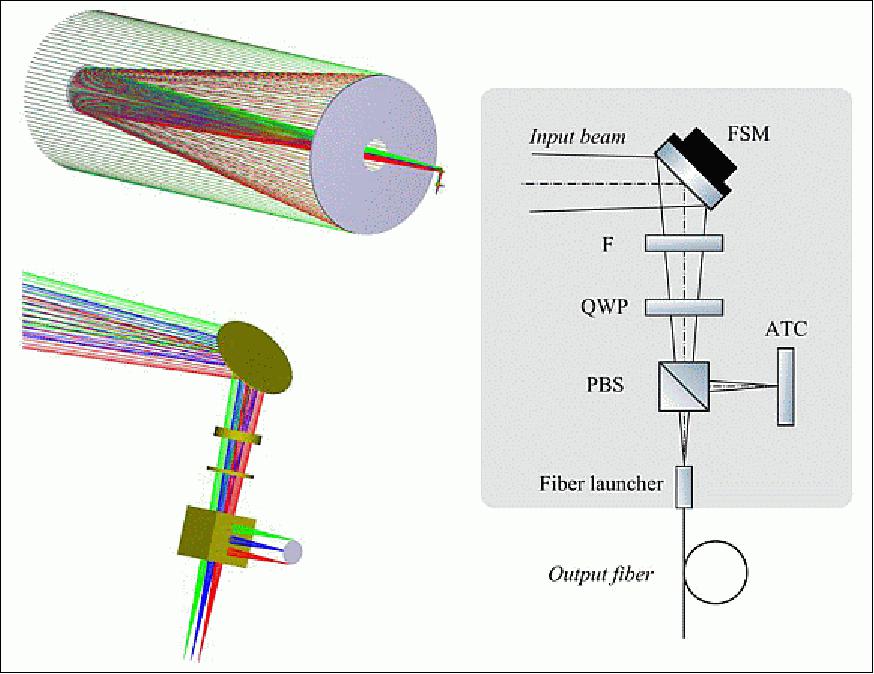
Acronyms in Figure 53: FSM (Fine Steering Mirror), F (Filter), QWP (Quarter Wave Plate), PBS (Polarizing Beam Splitter), ACT (Acquisition and Tracking Camera).
The focal plane instrumentation is schematically shown in Figure 53 and its real implementation in Figure 54. It does not include a collimator but routes the converging beam from the telescope via a filter wheel (where two narrow band-pass interference filters can be selected with 2.4 nm and 5 nm FWHM bandwidth), a tip/tilt FSM to adjust the PAA (Point Ahead Angle), a computer-rotated QWP and a PBS to the ACT and the receiver fiber. As the receive beam is circularly polarized, the QWP and PBS pair enable continuous adjustment of power ratio between the ATC and the detector fiber. During the initial satellite acquisition all power is directed towards ATC, until irradiance increases. Then the optical power towards the ATC is reduced (to the minimum level required to perform telescope pointing corrections) in favor of providing maximum power to the detector fiber.
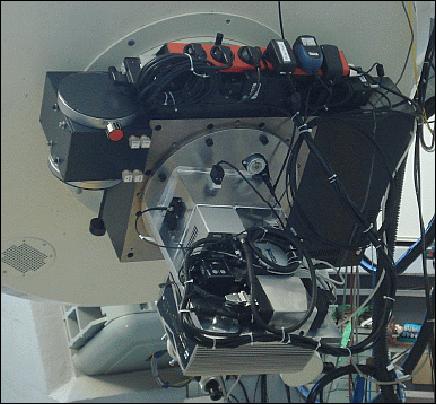
The design did not include a fast tip/tilt compensation loop, because a data receiver's FOV (Field of View) of 15 µrad was assumed to cover all possible angle of arrival fluctuations. Unfortunately, this turned out to be incorrect and was improved in the LLCD campaign in April 2014.
The detection, demodulation and data storage system was developed by RUAG Space and is installed in the Coudé laboratory of the OGS. It is connected to the focal plane instrumentation at the telescope via a 200 µm core diameter multimode fiber and its location provides a temperature stabilized environment.
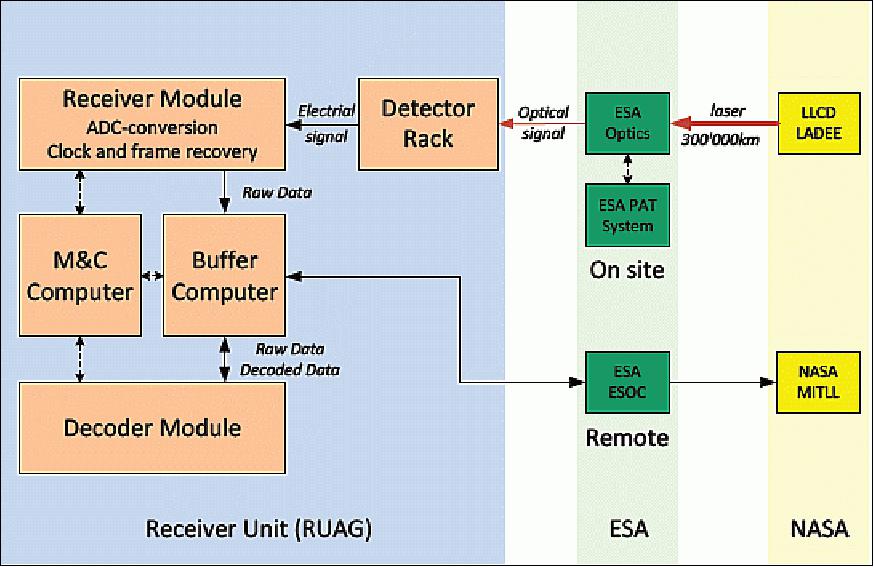
The downlink budget is given in Table 5. For the acquisition and tracking camera (ATC) it takes the minimum irradiance specified from LLST (at the start of acquisition) at the top of the atmosphere and evaluates the received power per ATC pixel. This is put in relation with the expected background noise sources from the blue sky brightness (at daytime) and from the sun-illuminated lunar surface, taking an ATC pixel size or 20 µm x 20 µm and a seeing diameter of 1 arcsec = 65 µm into consideration.
After initial acquisition of the LLST signal, the tracking phase starts and the signal strength increases by a factor of 10. In the right column of Table 5 the received power is given for the OGS data detector, which is coupled via a 30 meter long graded index multimode fiber to the focal plane instrumentation. The assumption is made that the power splitting ration between the ACT and the fiber is set such that 90% reach the fiber and 10% the ACT. As the fiber core diameter is 200 µm (which corresponds to 3 arcsec), no coupling loss is assumed.
Parameter | ATC value | Detector value |
Irradiance from LLST at the border of the atmosphere | -67.7 dBm/m2 | -57.7 dBm/m2 |
Atmospheric transmission loss | -1.5 dB | -1.5 dB |
Telescope transmission loss included filter | -3.2 dB | -3.2 dB |
Seeing loss (pixel/1arcsec); beam splitting loss ATC/MMF (0.9) and MMF insertion loss (0.8) | -9.1 dB | -1.4 dB |
Rx antenna loss factor per unit area (0.72 m2) | -1.4 dB/m2 | -1.4 dB/m2 |
Received power | -82.9 dBm | -65.2 dBm |
Lunar irradiance background | -111.8 dBm | -92.8 dBm |
Blue sky brightness | -97.7 dBm | 78.7 dBm |
References
1) Tom Morgan, "Lunar Atmosphere and Dust Environment Explorer (LADEE)," Lunar Exploration Science Group, 22nd General Meeting, Oct. 2008, URL: http://ssedso.gsfc.nasa.gov/initiatives/lunar/LESWG/pubs_presentations/22ndGM/GSFC.ppt
2) Butler Hine, Stevan Spremo, Mark Turner, Robert Caffrey, "The Lunar Atmosphere and Dust Environment Explorer Mission," Proceedings of the 2010 IEEE Aerospace Conference, Big Sky, MT, USA, March 6-13, 2010
3) http://nssdc.gsfc.nasa.gov/nmc/spacecraftDisplay.do?id=LADEE
4) G. T. Delory, R. Elphic, T. Morgan, T. Colaprete, M. Horanyi, P. Mahaffy, B. Hine, D. Boroson, "The Lunar Atmosphere and Dust Environment Explorer (LADEE)," 40th Lunar and Planetary Science Conference, March 23-27, 2009, The Woodlands, TX, USA, URL: http://www.lpi.usra.edu/meetings/lpsc2009/pdf/2025.pdf
5) Rick Elphic, Brian Day, "NASA's Lunar Atmosphere and Dust Environment Explorer: Little Mission, Big Science," May 31, 2011, URL: http://learningcenter.nsta.org/products/symposia_seminars/NASAk12/files/Lunar
AtmosphereandDustEnvironmentExplorer-5-31-2011.pdf
6) T. Delory, R. C. Elphic, A. Colaprete, P. Mahaffy, M. Horanyi, "The LADEE Mission: The next step after the discovery of water on the moon," 41st Lunar and Planetary Science Conference (2010), The Woodlands, TX, USA, March 1-5, 2010, URL: http://www.lpi.usra.edu/meetings/lpsc2010/pdf/2459.pdf
7) R. C. Elphic, G. T. Delory, E. J. Grayzeck, A. Colaprete, M. Horanyi, P. Mahaffy, B. Hine, J. Salute D. Boroson, "The Lunar Atmosphere and Dust Environment Explorer (LADEE): T-Minus One Year and Counting," 2012, URL: http://www.lpi.usra.edu/meetings/leag2012/presentations/Elphic.pdf
8) Jason Emperador, "Lunar Atmosphere and Dust Environment Explorer Mission, LADEE S/C to Instrument Interface Protection Analysis, A06.LADEE.SCEIA Revision (-), Effective Date: 03/22/2011, URL: http://tomgraf.wikispaces.com/file/view/LADEE+Spacecraft+to+Instrument+Interface
+Protection+Analysis.pdf
9) "LADEE Fact Sheet," NASA, January 29, 2013, URL: http://www.nasa.gov/pdf/734209main_LADEE-fact%20-sheet-20130129.pdf
10) R. C. Elphic, B. Hine, G. T. Delory, J. S. Salut, S. Noble, A. Colaprete, M. Horanyi, P. Mahaffy, D. Boroson, "The Lunar Atmosphere and Dust Environment Explorer (LADEE): T-minus six months and counting," 44th Lunar and Planetary Science Conference, The Woodlands, TX, USA, March 18-22, 2013, paper: 3112, URL: http://www.lpi.usra.edu/meetings/lpsc2013/pdf/3112.pdf
11) Allen Verfeld, "LADEE mission studying the Moon's atmosphere," Urban Astronomer, Feb. 07, 2014 [web source no longer available]
12) "LADEE Arrives at Wallops for Moon Mission," Space Travel, June 10, 2013, URL: http://www.space-travel.com/reports/LADEE_Arrives_at_Wallops_for_Moon_Mission_999.html
13) Rachel Hoover, "LADEE Project Manager Update," NASA, Nov. 29, 2012, URL: http://www.nasa.gov/mission_pages/LADEE/news/2012-11-29-pm_update.html
14) "LADEE Completes Mission Critical Design Review," Moon Daily, August 4, 2011, URL: http://www.moondaily.com/reports/LADEE_Completes_Mission_Critical_Design_Review_999.html
15) Butler Hine, Stevan Spremo, Mark Turner, Robert Caffrey, "The Lunar Atmosphere and Dust Environment Explorer (LADEE) Mission," Proceedings of the 2010 IEEE Aerospace Conference, Big Sky, MT, USA, March 6-13, 2010, URL: http://ntrs.nasa.gov/archive/nasa/casi.ntrs.nasa.gov/20100021423_2010023280.pdf
16) "NASA Awards Propulsion System Contract for Moon-Bound Mission," NASA release: C09-159, Dec. 21, 2009, URL: http://www.nasa.gov/centers/ames/news/releases/2009/C09-159.html
17) Bert Vermeire, Josh Patin, Cameron Alakija, Mark Allard, "Digitally Intensive Precision Ranging Subsystem of the Lunar Atmospheric and Dust Environment Explorer's Transponder," Proceedings of the AIAA/USU Conference on Small Satellites, Logan, Utah, USA, August 2-7, 2014, paper: SSC14-III-10, URL: http://digitalcommons.usu.edu/cgi/viewcontent.cgi?article=3035&context=smallsat
18) Vanessa Kuroda, Mark Allard, Brian Lewis, Michael Lindsay, "Comm for Small Sats: The Lunar Atmosphere and Dust Environment Explorer (LADEE) Communications Subsystem," Proceedings of the AIAA/USU Conference on Small Satellites, Logan, Utah, USA, August 2-7, 2014, paper: SSC14-XII-4, URL: http://digitalcommons.usu.edu/cgi/viewcontent.cgi?article=3075&context=smallsat
19) "NASA's First Laser Communication System Integrated, Ready for Launch," NASA, March 14, 2013, URL: http://www.nasa.gov/mission_pages/LADEE/news/llcd-integrated.html
20) "LADEE Status," NASA, Sept. 7, 2013, URL: http://www.nasa.gov/mission_pages/ladee/main/index.html#.Ui1wxn_ODWJ
21) "NASA launches spacecraft to study Moon atmosphere," Space Travel, Sept. 7, 2013, URL: http://www.space-travel.com/reports/NASA_launches_spacecraft_to_study_Moon_atmosphere_999.html
22) Dwayne Brown, Rachel Hoover, Keith Koehler, "NASA Prepares for First Virginia Coast Launch to Moon," NASA Release 13-265, URL: http://www.nasa.gov/press/2013/august/nasa-prepares-for-first-virginia-coast-launch-to-moon/#.UhzH8n9fvr4
23) http://www.nasa.gov/mission_pages/LADEE/main/
24) Elizabeth Zubritsky, Bill Steigerwald, Alison Hawkes, "Meteoroid Strikes Eject Precious Water From Moon," NASA/GSFC Release 19-03, 15 April 2019, URL: https://www.nasa.gov/press-release/goddard/2019/ladee-lunar-water
25) M. Benna, D. M. Hurley, T. J. Stubbs, P. R. Mahaffy & R. C. Elphic, "Lunar soil hydration constrained by exospheric water liberated by meteoroid impacts," Nature Geoscience, ISSN 1752-0908, Published: 15 April 2019, https://doi.org/10.1038/s41561-019-0345-3
26) Darryl E. Waller, "NASA's LADEE mission shows the force of meteoroid strikes on lunar exosphere," NASA, Dec. 17, 2015, URL: http://www.nasa-usa.de/feature/ames/ladee/nasa-s-ladee-mission-shows-the-force-of-meteoroid-strikes-on-lunar-exosphere
27) A. M. Cook, D. H. Wooden, A. Colaprete, D. A. Glenar, T. J. Stubbs,"First detection of dust in the lunar tail: LADEE UVS measurements," 46th LPSC (Lunar and Planetary Science Conference), The Woodlands, TX, USA, March 16-20, 2015, URL: http://www.hou.usra.edu/meetings/lpsc2015/pdf/2147.pdf
28) Nancy Neal-Jones, "NASA's LRO Spacecraft Captures Images of LADEE's Impact Crater," NASA/GSFC, Oct. 28, 2014, URL: http://www.nasa.gov/content/goddard/nasa-s-lro-spacecraft-captures-images-of-ladee-s-impact-crater/#.VJFE83vGX_U
29) "Lunar Laser Communications Demonstration (LLCD)," NASA, May 6, 2014, URL: http://www.nasa.gov/content/optical-communications-demonstrations/#.VE44ghZ9D_U
30) John J. Rush, Bernard L. Edwards, "International Interoperability Standards Development for Space Optical Communication," Proceedings of ICSOS 2014 (International Conference on Space Optical Systems and Applications), Kobe, Japan, May 7-9, 2014
31) "Laser-powered farewell to Moon Mission," ESA, April 25, 2014, URL: http://www.esa.int/Our_Activities/Operations/Laser-powered_farewell_to_Moon_mission
32) Rick Elphic, "LADEE Project Scientist Update: The Legacy Lives On!," NASA/ARC, April 22, 2014, URL: http://www.nasa.gov/ames/ladee-project-scientist-update-the-legacy-lives-on/#.U1n9JKKegkA
33) Bob King, "LADEE Sees Zodiacal Light before Crashing into Moon, but Apollo Mystery Remains," Universe Today, April 24, 2014, URL: http://www.universetoday.com/111482/ladee-sees-zodiacal-light-before-crashing-into-moon-but-apollo-mystery-remains/
34) Rachel Hoover, Dwayne Brown, Dewayne Washington, "NASA Completes LADEE Mission with Planned Impact on Moon's Surface," NASA, April 18, 2014, URL: http://www.nasa.gov/ames/nasa-completes-ladee-mission-with-planned-impact-on-moons-surface/#.U1HYhaKegkA
35) Ken Kremer, "NASA's Highly Productive LADEE Dust Explorer Probe Crashes into the Moon as Planned," Universe Today, April 18, 2014, URL: http://www.universetoday.com/111013/nasas-highly-productive-ladee-dust-explorer-probe-crashes-into-the-moon-as-planned/
36) Dwayne Brown, Rachel Hoover, "NASA Satellite to Continue Gathering Data Up to Planned Lunar Impact," NASA Release 14-098, April 3, 2014, URL: http://www.nasa.gov/press/2014/april/nasa-satellite-to-continue-gathering-data-up-to-planned-lunar-impact/#.Uz4VCaKegkA
37) Rachel Hoover, "LADEE Sends Its First Images of the Moon Back to Earth," NASA/ARC, Feb. 13, 2014, URL: http://www.nasa.gov/ames/ladee-sends-its-first-images-of-the-moon-back-to-earth/#.Uv8Z4_vnNeO
38) Rachel Hoover, "NASA Extends Moon Exploring Satellite Mission," NASA, Jan. 31, 2014, URL: http://www.nasa.gov/ames/nasa-extends-moon-exploring-satellite-mission/#.Uu8oo_vnNeM
39) Nancy Neal-Jones, "NASA's LRO Snaps a Picture of NASA's LADEE Spacecraft," Jan. 29, 2014, URL: http://www.nasa.gov/content/goddard/nasas-lro-snaps-a-picture-of-nasas-ladee-spacecraft/#.Uu8nyPvnNeN
40) Rick Elphic, "LADEE Project Scientist Update: Milestones, Maneuvers and Moisture?," NASA, Jan. 31, 2014, URL: http://www.nasa.gov/ames/ladee-project-scientist-update-milestones-maneuvers-and-moisture/#.Uu8iHfvnNeM
41) Dewayne Washington, "Laser Demonstration Reveals Bright Future for Space Communication," NASA/GSFC, URL: http://esc.gsfc.nasa.gov/267/278/279/490.html
42) Rick Elphic, "LADEE Project Scientist Update: Science Observations Begin," NASA, Dec. 11, 2013, URL: http://www.nasa.gov/content/ames/ladee-project-scientist-update-science-observations-begin/#.UqnHKCeFf_o
43) "LADEE Project Manager Update: Commissioning Complete," NASA News, Nov. 22, 2013, URL: http://www.nasa.gov/content/ladee-project-manager-update-commissioning-complete/#.UqFilSeFf_o
44) Rachel Hoover, Dwayne Brown, "NASA Spacecraft Begins Collecting Lunar Atmosphere Data," NASA news release 13-79AR, Nov. 21, 2013, URL: http://www.nasa.gov/content/nasa-spacecraft-begins-collecting-lunar-atmosphere-data/#.Uo7r7yfgy9w
45) "Moon mission beams laser data to ESA station," ESA, Nov. 1, 2013, URL: http://www.esa.int/Our_Activities/Operations/Moon_mission_beams_laser_data_to_ESA_station
46) Dewayne Washington, "Historic Demonstration Proves Laser Communication Possible," NASA, Oct. 28, 2013, URL: http://www.nasa.gov/content/goddard/historic-demonstration-proves-laser-communication-possible/#.Unc1RlPgy9w
47) Joshua Buck, Dewayne Washington, "NASA Laser Communication System Sets Record with Data Transmissions to and from Moon," NASA, Release 13-309, Oct. 22, 2013, URL: http://www.nasa.gov/press/2013/october/nasa-laser-communication-system-sets-record-with-data-transmissions-to-and-from/#.UmdTWFOzLWI
48) "NASA Laser Communication System Sets Record with Data Transmissions to and from Moon," Space Daily, Oct. 24, 2013, URL: http://www.spacedaily.com/reports/NASA_Laser_Communication_System_Sets
_Record_with_Data_Transmissions_to_and_from_Moon_999.html
49) "Lunar Laser Communication Demonstration on LADEE achieves world-record data download speeds from the moon," MIT/LL, Oct. 2013, URL: https://web.archive.org/web/20131024205342/http://www.ll.mit.edu/news/LLCDachievesrecorddatadownloadspeed.html
50) "LADEE status updates," NASA, Oct. 17, 2013, URL: http://www.nasa.gov/centers/ames/ladee-status-update-oct-17-2013/#.UmSxLlOzLWI
51) "LADEE Project Manager Update: Instrument Checkout Complete, Cruising to the Moon," NASA, Sept. 25, 2013, URL: http://www.nasa.gov/content/ladee-project-manager-update-instrument-checkout-complete-cruising-to-the-moon/#.UmSxi1OzLWI
52) "LADEE Project Manager Update: Perigee Maneuver 1 Complete," NASA, Sept. 13, 2013, URL: http://www.nasa.gov/content/ladee-project-manager-update-perigee-maneuver-1-complete/#.UmTQrFOzLWI
53) Michael Collier, Paul R. Mahaffy, Richard Hodges, Mehdi Benna, Todd T. King, "Neutral Mass Spectrometer (NMS) for the Lunar Atmosphere and Dust Environment Explorer (LADEE) Mission," NASA Lunar Science Forum, NASA/ARC, Moffett Field, CA, USA, July 19-21, 2011
54) T. King, F. Jaeger, E. Raaen, E. Lyness, M. Collier, M. Benna, "Calibration of the Neutral Mass Spectrometer for the Lunar Atmosphere and Dust Environment Explorer (LADEE) Mission," 43rd Lunar and Planetary Science Conference, The Woodlands, Tx, USA, March 19-23, 2012, URL: http://www.lpi.usra.edu/meetings/lpsc2012/pdf/2144.pdf
55) M. Benna, P. R. Mahaffy, R. R. Hodges, "Early results from exospheric observations by the Neutral Mass Spectrometer (NMS)," 45th LPSC (Lunar and Planetary Science Conference), The Woodlands, TX, USA, March 17-21, 2014, URL: http://www.hou.usra.edu/meetings/lpsc2014/pdf/1535.pdf
56) Kara Vargo, "The LADEE Ultraviolet and Visible Light Spectrometer ," NASA Lunar Science Forum, NASA/ARC, Moffett Field, CA, USA, July 19-21, 2011
57) A. Colaprete, R. C. Elphic, D. Landis, J. Karcz, L. Osetinsky, M. Shirley, K. Vargo, D. Wooden, "Overview of the LADEE Ultraviolet-Visible Spectrometer: Design, Performance and Planned Operations," 44th Lunar and Planetary Science Conference ,The Woodlands, TX, USA, March 18-22, 2013, URL: http://www.lpi.usra.edu/meetings/lpsc2013/pdf/2293.pdf
58) A. Colaprete, R. C. Elphic, D. Landis, J. Karcz, M. Shirley, K. Vargo, D. Wooden, B. Hermalyn, "Overview of the LADEE Ultraviolet-Visible Spectrometer: Design, Operations and initial results," 45th LPSC (Lunar and Planetary Science Conference), The Woodlands, TX, USA, March 17-21, 2014, URL: http://www.hou.usra.edu/meetings/lpsc2014/pdf/2566.pdf
59) Mihaly Horányi, Zoltan Sternovsky, Eberhard Grün, Sascha Kempf , Ralf Srama, F. Postberg, "LDEX: Lunar Dust Experiment with Chemical Analysis Capability to search for Water," 42nd Lunar and Planetary Science Conference (2011), The Woodlands, TX, USA, March 7-11, 2011, URL: http://www.lpi.usra.edu/meetings/lpsc2011/pdf/1656.pdf
60) Zoltan Sternosvky, Mihaly Horanyi, Eberhard Gruen, Siegfried Auer, Sascha Kempf, Keith Drake, Jianfeng Xia, Ralf Srama, Anna Mocker, "LDEX: The dust detector on LADEE (Lunar Atmosphere and Dust Environment Explorer)," NASA Lunar Science Forum, NASA/ARC, Moffett Field, CA, USA, July 19-21, 2011
61) M. Horányi, Z. Sternovsky, M. Lankton, D. James, J. Szalay, K. Drake, A. Shu, A. Colette, E. Grün, S. Kempf, R. Srama, A. Mocker, "The Dust Environment of the Moon: Expectations for the Lunar Dust Experiment (LDEX)," 43rd Lunar and Planetary Science Conference, The Woodlands, Tx, USA, March 19-23, 2012, paper: 2635, URL: http://www.lpi.usra.edu/meetings/lpsc2012/pdf/2635.pdf
62) M. Horányi, S. Gagnard, D. Gathright, E. Grün, D. James, S. Kempf, M. Lankton, R. Srama, Z. Sternovsky, J. Szalay, "The dust environment of the moon as seen by the Lunar Dust Experiment (LDEX)," 45th LPSC (Lunar and Planetary Science Conference), The Woodlands, TX, USA, March 17-21, 2014, URL: http://www.hou.usra.edu/meetings/lpsc2014/pdf/1303.pdf
63) J. R. Szalay, M. Horanyi, A. R. Poppe, J. S. Halekas, "LDEX observations and correlations with ARTEMIS measurements," 45th LPSC (Lunar and Planetary Science Conference), The Woodlands, TX, USA, March 17-21, 2014, URL: http://www.hou.usra.edu/meetings/lpsc2014/pdf/1500.pdf
64) "Special Session: Lunar Dust and Exosphere featuring the first results from LADEE," 45th LPSC (Lunar and Planetary Science Conference), The Woodlands, TX, USA, March 17-21, 2014, URL: http://www.hou.usra.edu/meetings/lpsc2014/pdf/sess201.pdf
65) Bryan S. Robinson, Don M. Boroson, Dennis A. Burianek, Daniel V. Murphy, "Overview of the lunar laser communications demonstration," Proceedings of SPIE, Vol. 7993, "Free-Space Laser Communication Technologies XXIII," San Francisco, CA, USA, Jan. 26, 2011, doi:10.1117/12.878313
66) Steven Constantine, Laura E. Elgin, Mark L. Stevens, Joseph A. Greco, Kenneth Aquino, Daniel D. Alves, Bryan S. Robinson, "Design of a high-speed space modem for the lunar laser communications demonstration," Proceedings of SPIE, Vol. 7923, "Free-Space Laser Communication Technologies XXIII," San Francisco, CA, USA, Jan. 26, 2011, doi:10.1117/12.878927
67) D. M. Boroson, J. J. Scozzafava, D. V. Murphy, B. S. Robinson, H. Shaw, "The Lunar Laser Communications Demonstration (LLCD)," Third IEEE International Conference on Space Mission Challenges for Information Technology (SMC-IT), Pasadena, CA, USA, July 19-23, 2009 [web source no longer available]
68) John Rush, Ken Perko, "NASA Plan for Development of Optical Communication for Space Applications," May 15, 2009, URL: http://www.spacepolicyonline.com/pages/images/stories/Rush_NRC_Optical
_Comm_Briefing_15_May_09.pdf
69) Bernard L. Edwards, Dave Israel, Keith Wilson, John Moores, Andrew Fletcher, "Overview of the Laser Communications Relay Demonstration Project," Proceedings of SpaceOps 2012, The 12th International Conference on Space Operations, Stockholm, Sweden, June 11-15, 2012, URL: http://www.spaceops2012.org/proceedings/documents/id1261897-paper-001.pdf
70) http://esc.gsfc.nasa.gov/267/271.html
71) Don M. Boroson, "Overview of the Lunar Laser Communication Demonstration," Proceedings of ICSOS 2014 (International Conference on Space Optical Systems and Applications), Kobe, Japan, May 7-9, 2014
72) Bryan S. Robinson, Don M. Boroson, Dennis A. Burianek, Daniel V. Murphy, Farzana I. Khatri, Jamie W. Burnside, Jan E. Kansky, Abhijit Biswas, Zoran Sodnik, Donald M. Cornwell, "The NASA Lunar Laser Communication Demonstration—Successful High-Rate Laser Communications To and From the Moon," SpaceOps 2014, 13th International Conference on Space Operations, Pasadena, CA, USA, May 5-9, 2014, paper: AIAA 2014-1685, URL: http://arc.aiaa.org/doi/pdf/10.2514/6.2014-1685
73) Farzana I. Khatri, Bryan S. Robinson, Marilyn D. Semprucci, Don M. Boroson,"Lunar Laser Communications Demonstration Operations Architecture," Proceedings of the 65th International Astronautical Congress (IAC 2014), Toronto, Canada, Sept. 29-Oct. 3, 2014, paper: IAC-14-B6.2.9
74) C. E. DeVoe, A. D. Pillsbury, F. Khatri, J. M. Burnside, A. C. Raudenbush, L.J. Petrilli, T. Williams, "Optical overview and qualification of the LLCD space terminal," Proceedings of the ICSO (International Conference on Space Optics), Tenerife, Canary Islands, Spain, Oct. 7-10, 2014, URL: http://congrexprojects.com/Custom/ICSO/2014/Papers/1.%20Tuesday%207%20October/Session
%202D%20Free%20Space%20Optical%20Comms/3.66838_DeVoe.pdf
75) C. Roth, D. Stadelmann, F. Arnold, C. Benkeser, Q. Huang, "High-throughput hardware decoder implementation for optical deep-space communications," Proceedings of TTC 2013, 6th International Workshop on Tracking Telemetry and Command Systems for Space Applications, Darmstadt, Germany, Sept. 10-13, 2013
76) http://spaceflightsystems.grc.nasa.gov/PlanetaryScience/documents/LADEE-LLCD.pdf
77) M. M. Willis, A. J. Kerman, M. E. Grein, J. Kansky, B. R. Romkey, E. A. Dauler, D. Rosenberg, B. S. Robinson, D. V. Murphy, D. M. Boroson, "Performance of a Multimode Photon-Counting Optical Receiver for the NASA Lunar Laser Communications Demonstration," Proceedings of the ICSOS (International Conference on Space Optical Systems and Application) 2012, Ajaccio, Corsica, France, October 9-12, 2012, URL: http://icsos2012.nict.go.jp/pdf/1569603327.pdf
78) Marc Sans, Zoran Sodnik, Igor Zayer, Robert Daddato, "Design of the ESA Optical Ground Station for Participation in LLCD," Proceedings of the ICSOS (International Conference on Space Optical Systems and Application) 2012, Ajaccio, Corsica, France, October 9-12, 2012, URL: http://icsos2012.nict.go.jp/pdf/1569600363.pdf
79) "Laser communications set for moon mission," ESA, July 30, 2013, URL: http://www.esa.int/Our_Activities/Operations/Laser_communications_set_for_Moon_mission
80) Zoran Sodnik, Hans Smit, Marc Sans, Igor Zayer, Marco Lanucara, Klaus-Jürgen Schulz, Dirk Giggenbach, Peter Becker, Ramon Mata-Calvo, Christian Fuchs, Johannes Widmer, Felix Arnold, Martin Mosberger, Angel Alonso, Iciar Montilla, "Results from a Lunar Laser Communication Experiment between NASA's LADEE Satellite and ESA's Optical Ground Station," Proceedings of ICSOS 2014 (International Conference on Space Optical Systems and Applications), Kobe, Japan, May 7-9, 2014, URL: http://elib.dlr.de/89892/1/__192.168.178.179_NAS-Share_OP_Projects_publications_All_2014-Sodnik
-CNF-ICSOS2014-LOCL_Results_2014_Sodnik_ICSOS2014_LOCL_final_S2-1.pdf
81) Dirk Giggenbach, Peter Becker, Ramon Mata-Calvo, Christian Fuchs, "Lunar Optical Communications Link (LOCL): Measurements of Received Power Fluctuations and Wavefront Quality," Proceedings of ICSOS 2014 (International Conference on Space Optical Systems and Applications), Kobe, Japan, May 7-9, 2014
82) Igor Zayer, Robert Daddato, Marco Lanucara, Klaus-Jürgen Schulz, Zoran Sodnik, Hans Smit, Marc Sans, Johan Rothman, Dirk Giggenbach, Peter Becker, Ramon Mata-Calvo, Christian Fuchs, "Lunar Optical Communications Link (LOCL) Demonstration between NASA's LADEE Spacecraft and ESA's Optical Ground Station," SpaceOps 2014, 13th International Conference on Space Operations, Pasadena, CA, USA, May 5-9, 2014, URL: http://arc.aiaa.org/doi/pdf/10.2514/6.2014-1919
83) Martin Mosberger, Johannes Widmer, Felix Arnold, Fabio Gambarara, "Receiver Performance of ESA Ground Terminal During Lunar Laser Communication Demonstration (LLCD)," Proceedings of ICSOS 2014 (International Conference on Space Optical Systems and Applications), Kobe, Japan, May 7-9, 2014
The information compiled and edited in this article was provided by Herbert J. Kramer from his documentation of: "Observation of the Earth and Its Environment: Survey of Missions and Sensors" (Springer Verlag) as well as many other sources after the publication of the 4th edition in 2002. - Comments and corrections to this article are always welcome for further updates (eoportal@symbios.space).
Spacecraft Launch Mission Status Sensor Complement Ground Segment References
Back to Top