SOHO (Solar and Heliospheric Observatory)
Non-EO
ESA
NASA
Operational (extended)
Quick facts
Overview
Mission type | Non-EO |
Agency | ESA, NASA |
Mission status | Operational (extended) |
Launch date | 02 Dec 1995 |
SOHO (Solar and Heliospheric Observatory)
Spacecraft Launch Mission Status Sensor Complement Mission Achievements SOHO - Comet hunter References
SOHO is an ESA/NASA collaborative mission within ESA's `Solar Terrestrial Science Program' (STSP), and it is also part of ISTP (International Solar Terrestrial Physics Program). So far, SOHO represents the most comprehensive space mission devoted to the study of the sun and of the heliosphere. Within the collaborative mission, the functions are divided in the following way: ESA is responsible for S/C procurement, integration and testing; NASA provided the spacecraft launch and mission operations from GSFC. The following overall mission objectives are pursued: 1) 2) 3) 4) 5) 6)
• Solar spectroscopy at soft X-ray and EUV wavelengths (study of the composition of the solar corona, of the structure and dynamics of the magnetic structures making up the corona, and of coronal holes, etc.)
• Study of the structure and dynamics of the solar interior through the observation of minute oscillations on the sun's surface (helio-seismology).
• Study of the solar wind and solar energetic particles, interaction with the Earth, plasma processes in both the solar and magnetospheric context.
History
SOHO was first proposed 13 years before its actual launch and the roots of SOHO were laid in earlier studies, namely those of GRIST (Grazing Incidence Solar Telescope) and DISCO (Dual Spectral Irradiance and Solar Constant Orbited). It is the combination of the objectives of these two missions that constitutes the core of the SOHO mission. 7)
In June 1976, GRIST had been competing with a 'Solar Probe' as well as other studies involving other disciplines for further study. Solar Probe envisaged a set of instruments on a spacecraft that would get close to the Sun. Although its assessment study cited four scientific disciplines interested in the mission, Solar Probe was not followed up at the time.
The GRIST study, on the other hand, proceeded to a feasibility stage. GRIST was preferred over Solar Probe because the wavelength range accessible through its optics was particularly useful for studying the hot outer solar atmosphere. GRIST was at that time being designed for flights on Spacelab.
Following the 1976 study, GRIST did not make it to project selection either. It was based on a collaboration with NASA which became a victim in 1981 of NASA's cancellation of the US probe in the International Solar Polar Mission (ISPM, the former 'Out-of-Ecliptic Mission', now called Ulysses). GRIST was 'parked', but restricted studies on its main spectrometers were supported by ESA.
In 1980, a group of French and American physicists observed the Sun continuously from Antarctica, studying solar physics with the best conditions available on Earth. These historic observations led to the decision to include the same sort of experiments on board a newly proposed mission called DISCO. DISCO would sit at the L1 Lagrange point between the Sun and Earth, which would be an ideal observing site. A miniaturized version of the South Pole experiment could be used as part of DISCO’s payload, provided its weight could be reduced.
DISCO was conceived as a fairly small and cheap spin-stabilized spacecraft, weighing no more than 520 kg. It was intended to prove that ESA could also undertake small and inexpensive missions. A first assessment was made in 1981, when DISCO had remained a relatively inexpensive spinning satellite, very similar to Cluster. ESA's Solar System Working Group preferred DISCO to a competing Mars mission called 'Keller', but DISCO eventually lost out to the Infrared Space Observatory in 1983.
SOHO itself developed as a mission in 1983, combining many of the aspects of the previously planned missions. It became important because it developed momentum, together with the Cluster mission, as part of the ISTP (International Solar–Terrestrial Physics) program. In May 1984, ESA identified SOHO as a part of the 'Cornerstone' of its long-term 'Horizon 2000' science program.
Partnerships: SOHO is part of the first Cornerstone project in ESA's Science program (the other is Cluster). Both are joint ESA/NASA projects in which ESA is the senior partner. SOHO and Cluster are also contributions to the ISTP program, to which ESA, NASA, Japan, Russia, Sweden and Denmark all contribute satellites monitoring the Sun and solar effects.
Of the spacecraft's 12 instruments, nine come from multinational teams led by European scientists, and three from US-led teams. More than 1500 scientists from around the world have been involved with the SOHO program.
SOHO was built by industrial companies in 14 European countries, led by Matra Marconi Space (now Astrium). The service module, with solar panels, thrusters, attitude control systems, communications, and housekeeping functions, was prepared in Toulouse, France. The payload module carrying the scientific instruments was assembled in Portsmouth, United Kingdom, and mated with the service module in Toulouse, France. NASA launched SOHO and is responsible for tracking, telemetry reception, and commanding (Ref. 7).
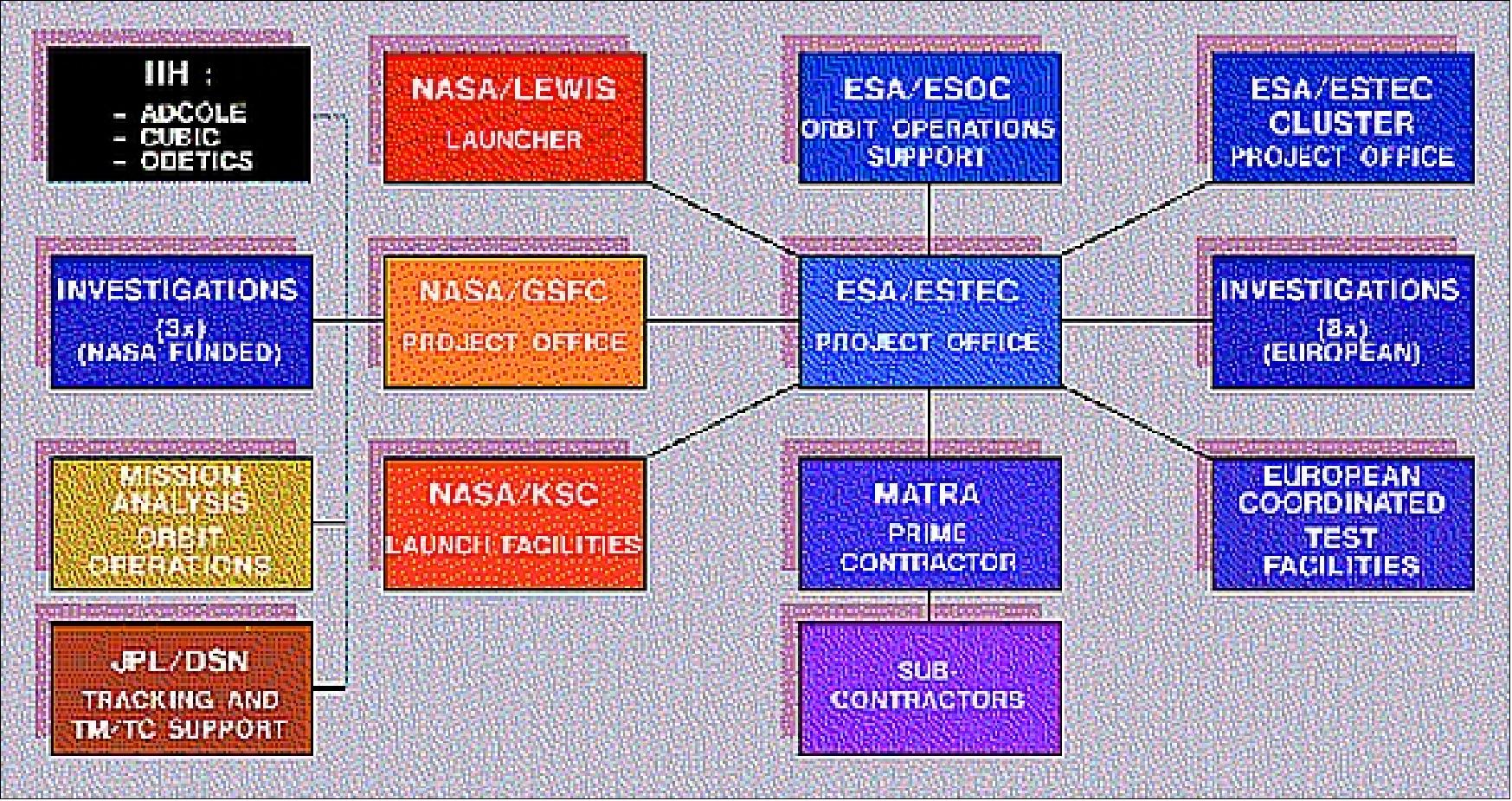
Figure 1: Overview of the main SOHO mission participants (image credit: ESA) 8)
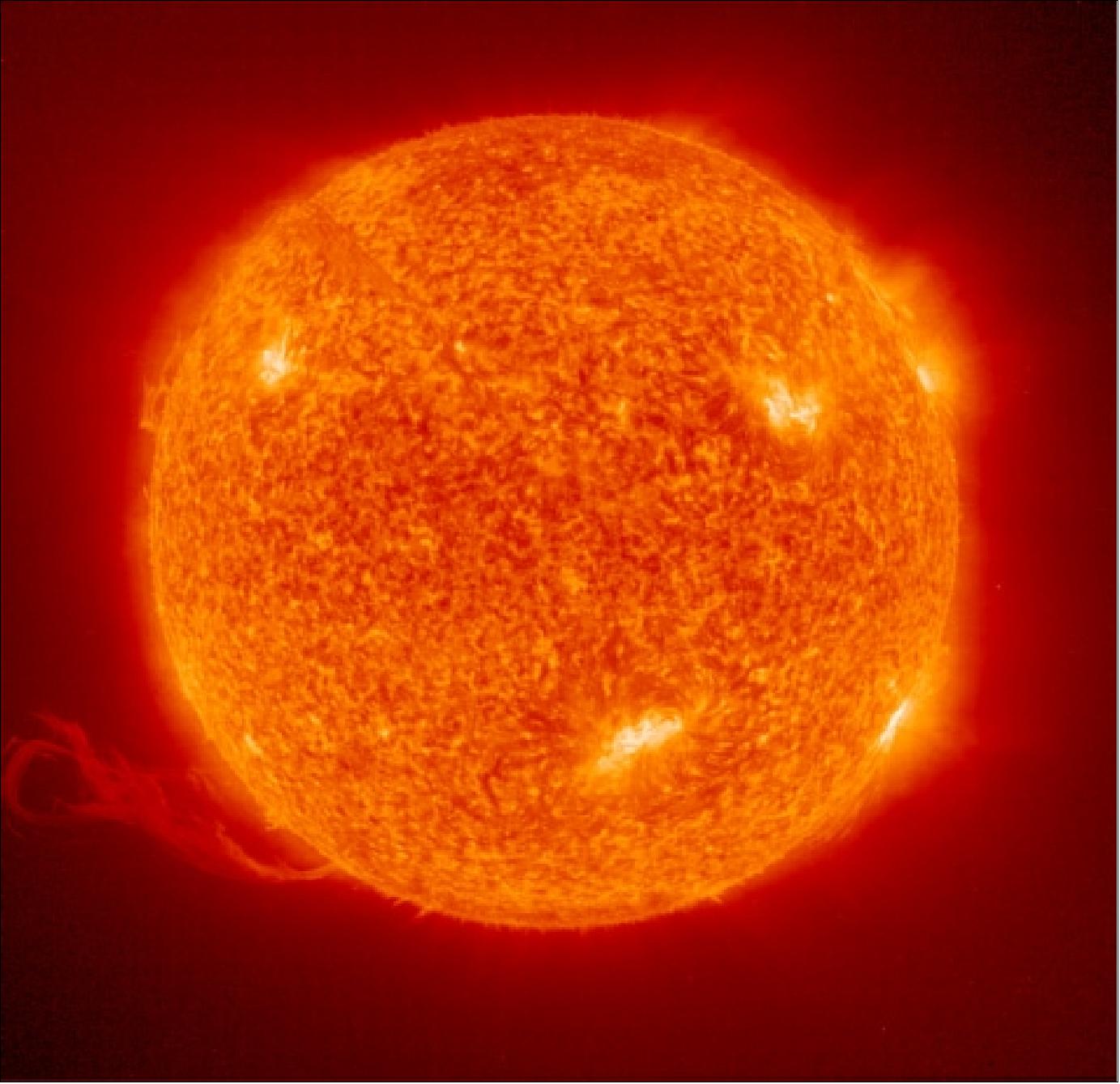
Figure 2: SOHO image of the sun in the extreme ultraviolet range with the EIT instrument (image credit: ESA, NASA)
Spacecraft
The spacecraft was built by EADS-Astrium [formerly Matra Marconi Space (MMS)] as prime contractor. The SOHO spacecraft consists of two major elements, the Service Module (SVM) and the Payload Module (PLM). 9)
• SVM is a box-shaped structure made of aluminium honeycomb panels attached to a corrugated aluminium cylinder by four shear webs. The four lateral panels carry the data-handling, communication, attitude and orbit control, and power subsystems. The box's upper floor houses the propulsion subsystem, tank and thruster masts. The high-gain antenna is mated into the aft part of the central cylinder. The SVM with solar panels, thrusters, attitude control systems, communications and housekeeping functions, was prepared in Toulouse, France.
• PLM provides an optical bench for the experiments and is composed of four upper lateral panels and a top panel connected to a central cylinder by a number of shear webs. The bottom section, consisting of three lower lateral panels connected to the central cylindrical tube by a number of shear webs and floors, houses the PLM electronics. The PLM, carrying the scientific instruments, was assembled in Portsmouth, UK, and mated with the service module in Toulouse.

Figure 3: Schematic illustration of the deployed SOHO spacecraft (image credit: ESA)

Figure 4: Artist's view of the deployed SOHO spacecraft (image credit: ESA)
SOHO has dimensions of 4.3 m x 2.7 m x 3.65 m (and 9.5 m span of the solar array).
AOCS (Attitude and Orbit Control Subsystem): The S/C is three-axis stabilized within 10 arcsec, with a sun-pointing stability of 1 arcsec over a period of 1.5 minutes. Actuators are 4 reaction wheels and two sets of eight thrusters. Attitude is measured by two fine-pointing sun sensors (FPSS), two star trackers also referred to as SSU (Star Sensor Unit), and three gyros. An SSU can track up to five stars. One of the stars is designated as guide star and is used as input for the control of the roll axis. When a single event upset occurs on the CCD inside or close to the guide star image the star data becomes unusable for control.
AAD (Attitude Anomaly Detector): The AOCS design is based on using independent hardware for anomaly detection and separate hardware for the safe mode. There is a pair of attitude anomaly detectors triggering when the sun off pointing is larger than 5º (Fine Sun Pointing Attitude Anomaly Detector, FSPAAD) and 25º (Coarse Sun Pointing Attitude Anomaly Detector, CSPAAD) respectively. These anomaly detectors are very simple (Figure 5). They consist of a cylinder with a hole at the top, internal baffles and a solar cell at the bottom (Ref. 79).
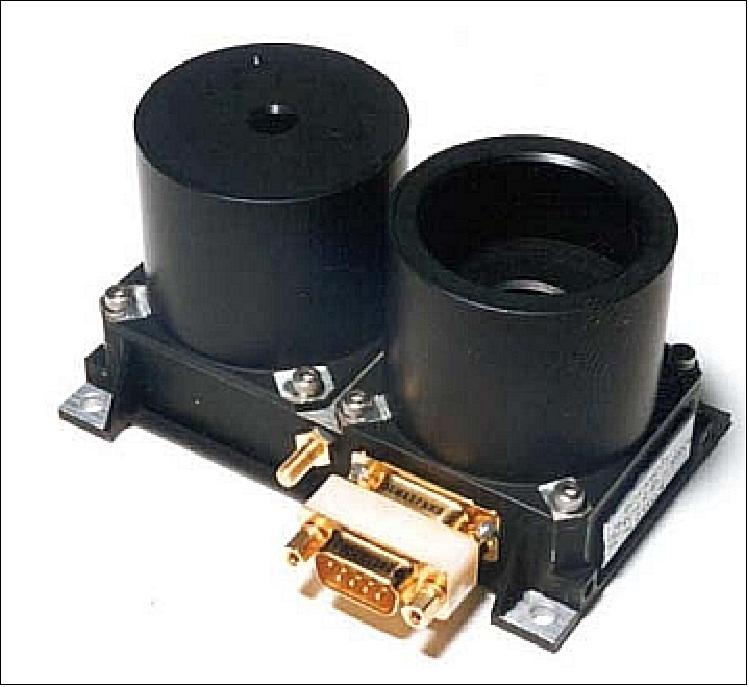
Figure 5: The FSPAAD (left) and CSPAAD (right), image credit: ESA
Power = 1500 W (solar cells) and 950 W from two 20 Ah NiCd batteries. The power bus is regulated to 28 V with a three-domain regulation of solar-array shunt mode, battery discharge and battery charge mode. S/C launch mass = 1850 kg, scientific payload mass = 610 kg, nominal lifetime = 3 years.
The data-handling central onboard software (in ADA) resides in a 16 bit computer (MAS 281) that uses the 1750A MIL-Standard instruction set and contains a set of programs controlling the data handling. It manages and distributes the onboard time, performs the thermal monitoring and thermal control of the satellite, manages the antenna pointing and monitoring, handles the inter-instrument data exchange, performs various other monitoring functions, and executes the initial sun-acquisition sequence. 10)
Spacecraft dimensions | 4.3 m x 2.7 m x 3.65 m (height, breadth, width) |
Length of solar array | 9.5 m |
Spacecraft mass at launch | 1850 kg |
Payload mass | 610 kg |
Spacecraft power | 1.5 kW |
Telemetry (real-time operations) | 200 kbit/s |
Table 1: Overview of some spacecraft parameters

Figure 6: The electrical architecture of the SOHO spacecraft
RF communications: Onboard solid-state recorder with 2 Gbit capacity. S-band data transmission (downlink frequency of 2.2-2.29 GHz, uplink of 2.025-2.110 GHz) with duplicated receivers and transmitters. RF coverage is provided by a pointable high-gain antenna (0.8 m dish) and two low-gain antennas, to ensure the full coverage. Housekeeping telemetry at 1 kbit/s, science data at 45 kbit/s, data dump (MDI, etc.) at 220 kbit/s.
The DSN (Deep Space Network) of NASA is providing TT&C support using existing ground station facilities at Madrid (Spain), Canberra (Australia) and Goldstone (CA, USA) and a central communications terminal at JPL. S/C operations are being performed by SMOCC (SOHO Mission Operations and Control Center) at NASA/GSFC. In addition, the SOHO POCC (Payload Operations Control Center) as well as other support functions are being performed at GSFC.
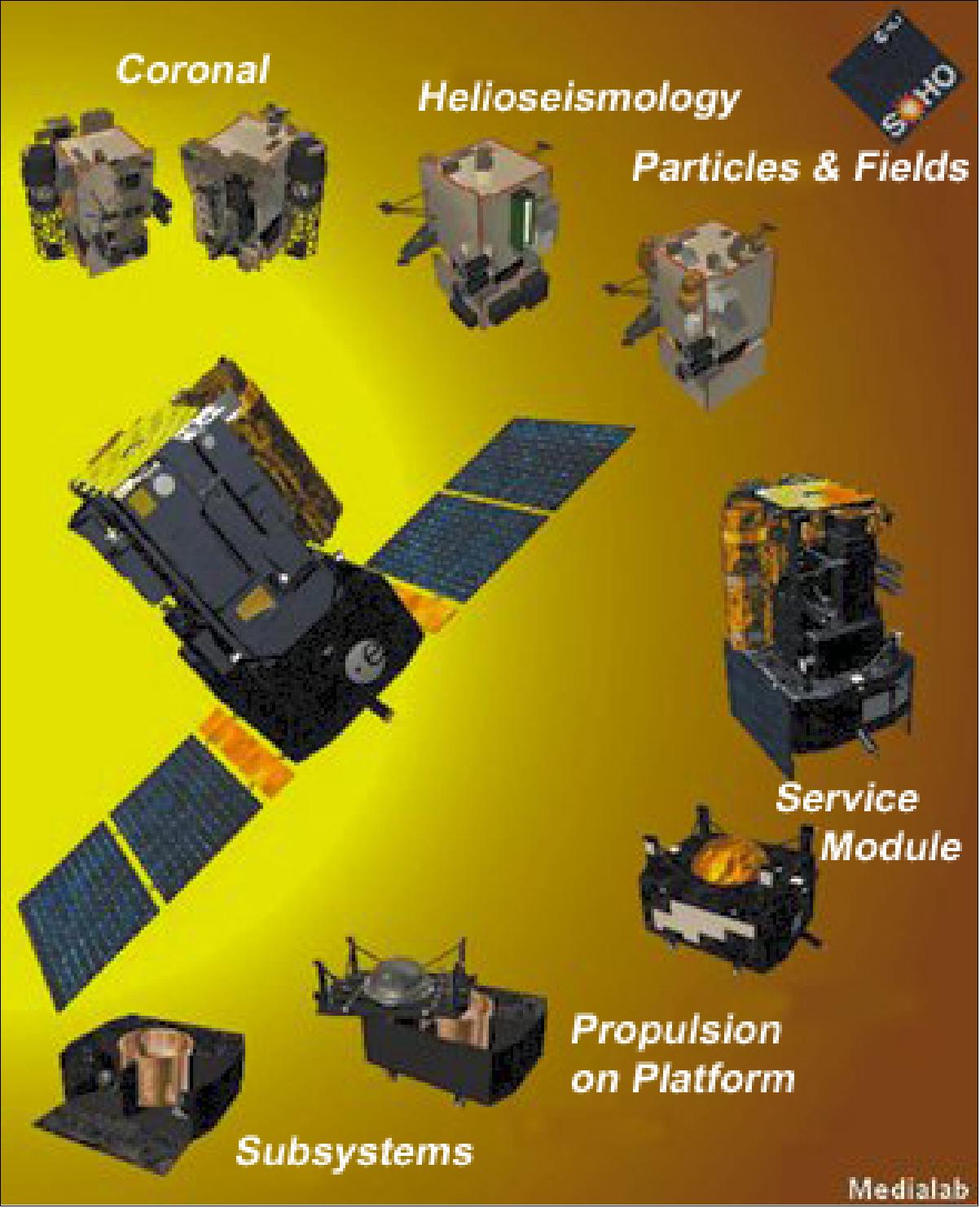
Figure 7: Two views of the SOHO spacecraft and its major components (image credit: ESA)
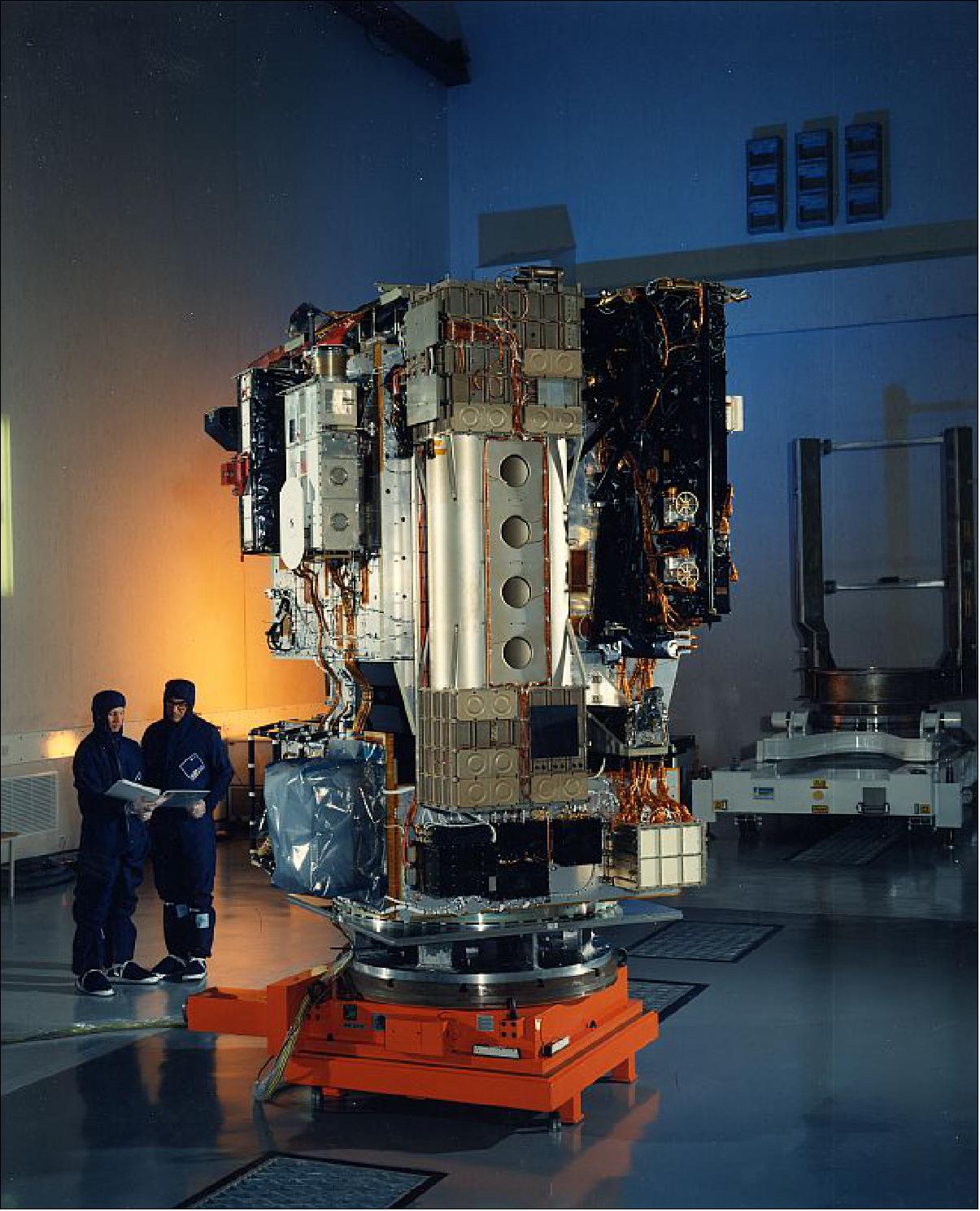
Figure 8: Photo of the SOHO spacecraft prior to launch (image credit: ESA/NASA)
Launch
A NASA provided launch of the SOHO spacecraft took place from Cape Canaveral, FL, on December 2, 1995 aboard an ATLAS-II AS vehicle.
Orbit: Halo orbit around the Sun/Earth Lagrangian Point (L1), about 1.5 million km from Earth. In the Sun/Earth system, L1 is located on the sunward side of the Sun-Earth axis, where SOHO enjoys an uninterrupted view of the sun. The pointing accuracy of the S/C in the direction of the sun is better than 1 arcsec. SOHO was inserted into its halo orbit around L1 on Feb. 14, 1996 (six weeks ahead of schedule - it retained practically all of its hydrazine supply). Just like the Earth, SOHO takes a full year to orbit the sun. Its orbit affords SOHO an uninterrupted view of the sun, unlike satellites that orbit the Earth and periodically see the sun ”eclipsed” by the Earth.

Figure 9: Schematic view of the SOHO halo orbit about L1 (image credit: NASA) 11)
Mission Status
• August 14, 2020: Scientists at Skolkovo Institute of Science and Technology (Skoltech), Moscow, Russia, together with colleagues from the Karl-Franzens University of Graz & the Kanzelhöhe Observatory (Austria), Jet Propulsion Laboratory of California Institute of Technology (USA), Helioresearch (USA) and Space Research Institute of the Russian Academy of Sciences (Russia) developed a method to study fast Coronal Mass Ejections, powerful ejections of magnetized matter from the outer atmosphere of the Sun. The results can help to better understand and predict the most extreme space weather events and their potential to cause strong geomagnetic storms that directly affect the operation of engineering systems in space and on Earth. The results of the study are published in the Astrophysical Journal. 12) 13)
- CMEs (Coronal Mass Ejections) are among the most energetic eruptive phenomena in our solar system and the main source of major space weather events. Huge clouds of plasma and magnetic flux are ejected from the atmosphere of the Sun into the surrounding space with speeds ranging from 100 to 3500 km/s. These gigantic solar plasma clouds and the accompanying powerful shock waves can reach our planet in less than a day, causing severe geomagnetic storms posing hazards to astronauts and technology in space and on Earth. One of the strongest Space Weather events occurred in 1859 when the induced geomagnetic storm collapsed the whole telegraph system in North America and Europe, the main means of communication for business and personal contacts in those days. If such an event occurs today, then modern devices are in no way protected. We may find ourselves without electricity, television, Internet, radio communications which would lead to significant and cascading effects in many areas of our life. Only a few years ago, in July 2012, an outburst of energy comparable to the event in the 19th century occurred on the Sun, but we were lucky because these outbursts did not touch the Earth. According to some experts, the damage from such an extreme event could cost up to several trillion dollars and the restoration of infrastructure and the economy could take up to 10 years. Thus, understanding and forecasting of the most hazardous extreme events is of prime importance for the protection of the society and technology against the global hazards of Space Weather.

Figure 10: Halloween Solar Storms during a two-week period in October and November of 2003, that affected a variety of technological systems around the world. A large active region with big sunspot group on the solar surface (left) erupted with a series of solar flares (middle) followed by the Coronal Mass Ejections (right) propagating into the interplanetary space. These events are usually accompanied by polar auroras and intense geomagnetic storms (image credit: SOHO/EIT/MDI/LASCO COR1+COR2)
- The current research resulted from an earlier work of Dr. Alexander Ruzmaikin, a former Ph.D. student of Academician Yakov Zeldovich and Dr. Joan Feynman, who has made important contributions to the study of Sun-Earth relations, the solar wind and its impact on the Earth magnetosphere, and who is the younger sister of Nobel Prize laureate Richard Feynman. In the current study, it was shown that the strongest and most intense geomagnetic storms are driven by fast Coronal Mass Ejections interacting in the interplanetary space with another Coronal Mass Ejection. Such interplanetary interactions among Coronal Mass Ejections occur in particular when they are launched in sequence one after another from the same active region. This type of ejection can be characterized using the concept of clusters that also generates an enhanced particle acceleration compared to the isolated plasma cloud. In general, the detection of clusters has important applications in many other extreme geophysical events such as floods and major earthquakes, as well as in interdisciplinary areas (hydrology, telecommunications, finance, and environmental studies).
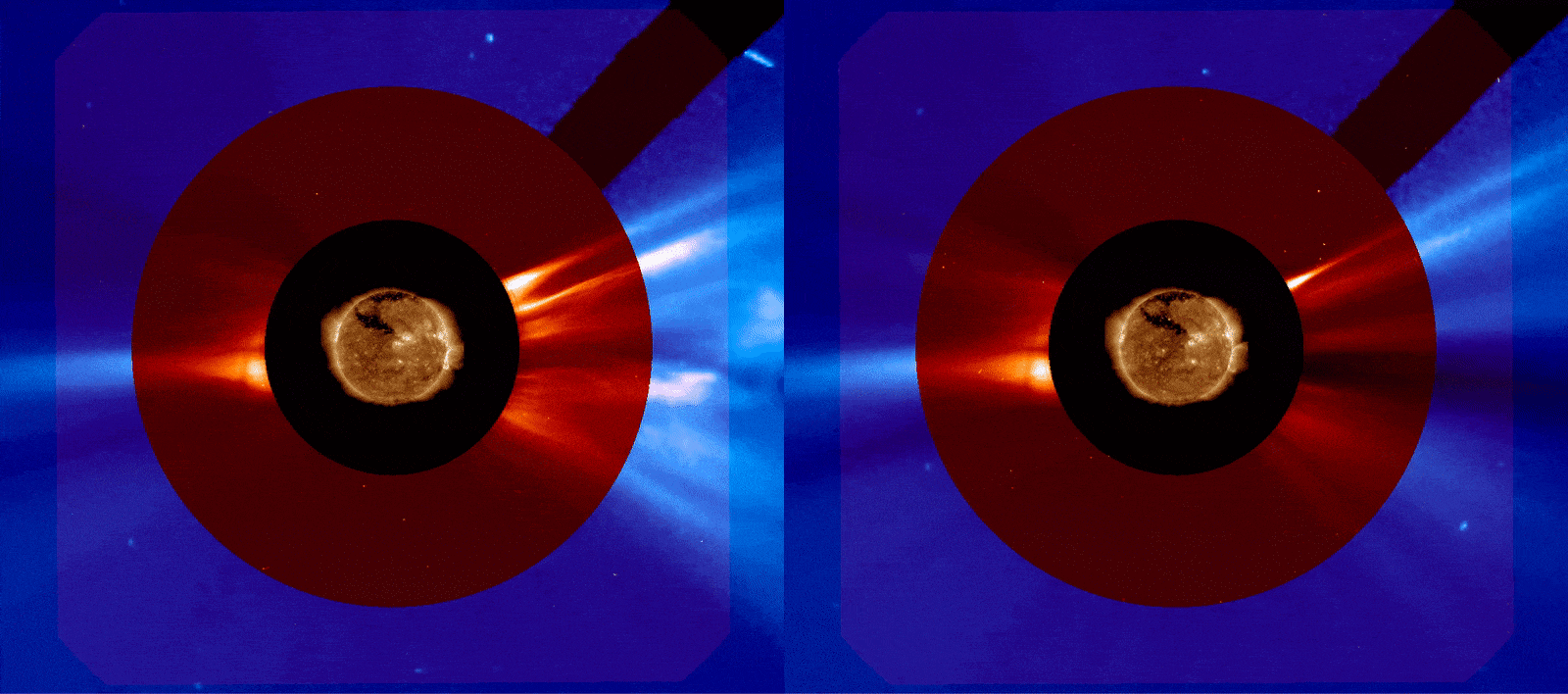
Figure 11: Cluster with two consecutive Coronal Mass Ejections on 9 (left) and 10 (right) September 2017 with speeds of 1148 and 3703 km/s respectively. The event occurred during the declining phase of the 11-year solar cycle n24 and forced the crew onboard International Space Station to move to the station’s shelter to protect themselves from the strong radiation emitted by the largest solar flare observed in the last 12 years (image credit: SDO/AIA +SOHO/LASCO COR1+COR2)
- “Understanding the characteristics of extreme solar eruptions and extreme space weather events can help us better understand the dynamics and variability of the Sun as well as the physical mechanisms behind these events,” says a research scientist of the Skoltech Space Center and the first author of the study, Dr. Jenny Marcela Rodríguez Gómez.
- Now we are at the beginning of a new 11-year cycle of solar activity, which, according to the predictions, will not be very strong. “However, this does not mean that no extreme events can happen”, says professor Astrid Veronig, co-author of the study and director of Kanzelhöhe Observatory of the University of Graz. Historically, extreme space weather events occurred during not-so-strong cycles or during the descending phase of a cycle. At the peak of the solar cycle, vast amounts of energy are released in the form of numerous Solar Flares and Coronal Mass Ejections. whereas during the descending phase of a cycle the energy accumulates and may be released in single but very powerful events. “Therefore, our modern technological society needs take this seriously, study extreme space weather events, and also understand all the subtleties of the interactions between the Sun and the Earth. And whatever storms may rage, we wish everyone a good weather in space,” says Tatiana Podladchikova, assistant professor at the Skoltech Space Center, research co-author.
• June 25, 2020: Solar activity fluctuates in a rhythm of about eleven years, which is reflected among other things in the frequency of sunspots. A complete magnetic period lasts 22 years. Scientists have long been puzzling over what causes this cycle. It must be related to the conditions beneath the "skin" of our star: A layer of hot plasma - electrically-conductive gas - extends from the surface to 200,000 kilometers below. The plasma within this convection zone is constantly in motion. A team of scientists from the MPS (Max Planck Institute for Solar System Research), the University of Göttingen and New York University Abu Dhabi has now succeeded in drawing the most comprehensive picture of the plasma flows in north-south-direction to date. The researchers have found a remarkably simple flow geometry: the plasma describes a single turnover in each solar hemisphere, which lasts for about 22 years. In addition, the flow in the direction of the equator at the bottom of the convection zone causes spots to form closer and closer to the equator during the solar cycle. 14) 15)
- The number of sunspots on the visible solar surface varies; sometimes there are more, sometimes fewer. The distance between two sunspot maxima is about eleven years, after 22 years the sunspots are again magnetically polarized in the same way. During the maximum not only large sunspots appear, but also active regions. In addition, impressive arcs of hot plasma reach far into the solar atmosphere, particles and radiation are hurled into space in violent eruptions. At the activity minimum, however, the sun calms down noticeably.
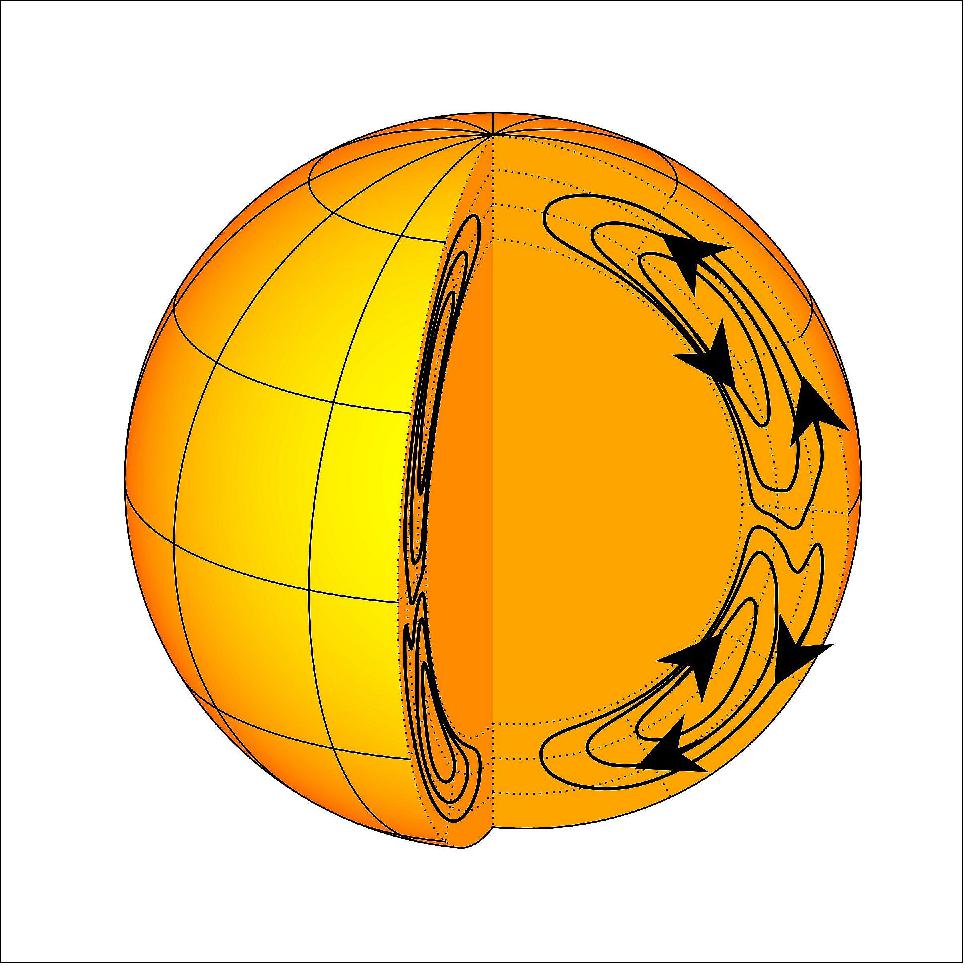
Figure 12: Powerful flows: Ionized gas inside the Sun moves toward the poles near the surface and toward the equator at the base of the convection zone (at a depth of 200,000 km (image credit: MPS)
- “Over the course of a solar cycle, the meridional flow acts as a conveyor belt that drags the magnetic field along and sets the period of the solar cycle”, says Prof. Dr. Laurent Gizon, MPS Director and first author of the new study. “Seeing the geometry and the amplitude of motions in the solar interior is essential to understanding the Sun’s magnetic field”, he adds. To this end, Gizon and his team used helioseismology to map the plasma flow below the Sun's surface.
- Helioseismology is to solar physics what seismology is to geophysics. Helioseismologists use sound waves to probe the Sun's interior, in much the same way geophysicists use earthquakes to probe the interior of the Earth. Solar sound waves have periods near five minutes and are continuously excited by near surface convection. The motions associated with solar sound waves can be measured at the Sun's surface by telescopes on spacecrafts or on the ground.
- In this study, Gizon and his team used observations of sound waves at the surface that propagate in the north-south direction through the solar interior. These waves are perturbed by the meridional flow: they travel faster along the flow than against the flow. These very small travel-time perturbations (less than 1 second) were measured very carefully and were interpreted to infer the meridional flow using mathematical modeling and computers.

Figure 13: Sound waves propagate throughout the Sun and can be measured at the solar surface, for example at A and B. Just as seismology is used to see inside the earth, helioseismology is used to see inside the Sun (here near the wave path connecting A and B), image credit: MPS, Z.-C. Liang
- Because it is small, the meridional flow is extremely difficult to see in the solar interior. “The meridional flow is much slower than other components of motion, such as the Sun’s differential rotation”, Gizon explains. The meridional flow throughout the convection zone is no more than its maximum surface value of 50 kilometers per hour. “To reduce the noise level in the helioseismic measurements, it is necessary to average the measurements over very long periods of time”, says Dr. Zhi-Chao Liang of MPS.
- The team of scientists analyzed, for the first time, two independent very long time series of data. One was provided by SOHO, the oldest solar observatory in space which is operated by ESA and NASA. The data taken by SOHO’s Michelson Doppler Imager (MDI) covers the time from 1996 until 2011. A second independent data set was provided by the Global Oscillation Network Group (GONG), which combines six ground-based solar telescopes in the USA, Australia, India, Spain, and Chile to offer nearly continuous observations of the Sun since 1995.
- “The international solar physics community is to be commended for delivering multiple datasets covering the last two solar cycles”, says Dr. John Leibacher, a former director of the GONG project. “This makes it possible to average over long periods of time and to compare answers, which is absolutely essential to validate inferences”, he adds.
Figure 14: Plasma in motion. The meridional flow, visualized here by moving corks, sets the period of the Sun’s magnetic cycle by transporting the magnetic field around the convection in 22 years, i.e. one magnetic sunspot cycle (video credit: MPS, Z.-C. Liang)
- Gizon and his team find the flow is equatorward at the base of the convection zone, with a speed of only 15 kilometers per hour (running speed). The flow at the solar surface is poleward and reaches up to 50 km/hour. The overall picture is that the plasma goes around in one gigantic loop in each hemisphere. Remarkably, the time taken for the plasma to complete the loop is approximately 22 years – and this provides the physical explanation for the Sun’s eleven-year cycle.
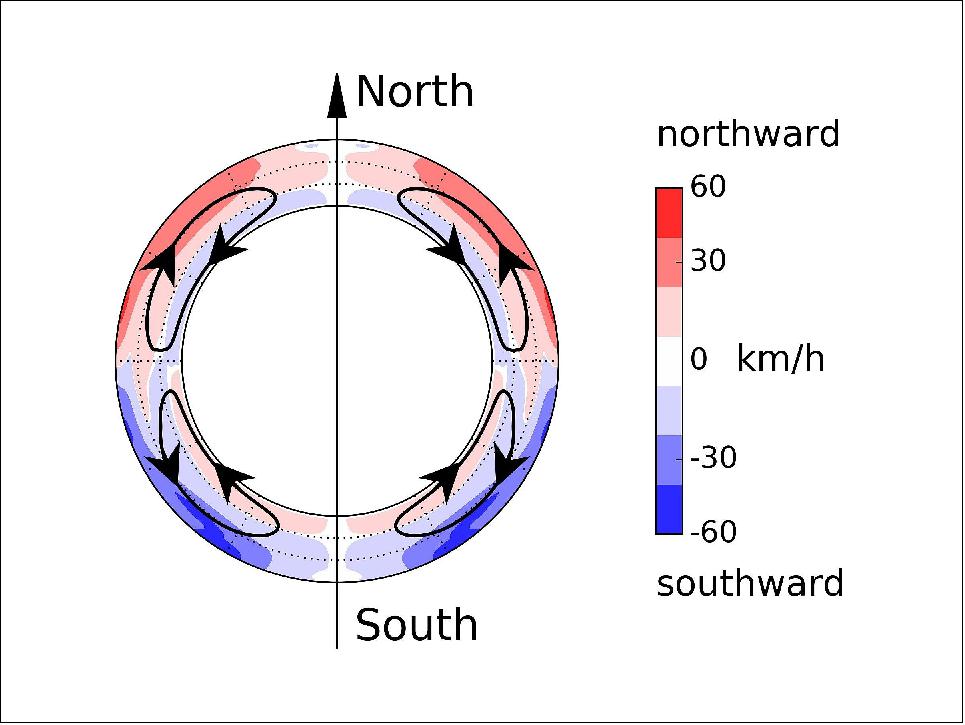
Figure 15: Driving force: Helioseismology was used to measure the Sun’s meridional flow. This flow controls the evolution of the global magnetic field and the number of sunspots (image credit: MPS)
- Furthermore, sunspots emerge closer to the equator as the solar cycle progresses, as is seen in the butterfly diagram. “All in all, our study supports the basic idea that the equatorward drift of the locations where sunspots emerge is due to the underlying meridional flows”, says Dr. Robert Cameron of MPS. “It remains to be understood why the solar meridional flow looks like it does, and what role the meridional flow plays in controlling magnetic activity on other stars” adds Laurent Gizon.
- The computing infrastructure for this work was supported by the German Aerospace Center (DLR) with funds from the Federal Ministry of Economics and Technology (BMWi) allocated by the German Bundestag. Partial support was provided by the European Research Council under Synergy Grant WHOLE SUN. The Center for Space Science at New York University Abu Dhabi is funded by the NYUAD Institute.
• June 17, 2020: Using data from the ESA/NASA SOHO mission, a European citizen scientist spotted a never-before-seen comet in the satellite data — the 4,000th comet discovery in the spacecraft’s 25-year history. 16)
- Currently nicknamed SOHO-4000 and pending its official designation from the Minor Planet Center, the comet has a rather small nucleus, estimated around five to ten meters in size, and it was extremely faint and close to the Sun when discovered. This means SOHO is the only observatory that has spotted the comet, as it is impossible to see from Earth – with or without a telescope.

Figure 16: The comet is seen here in an image from SOHO alongside the spacecraft’s 3999th comet discovery. The two comets are relatively close, approximately 1.5 million km apart, suggesting that they could have been connected together as recently as a few years ago (image credit: ESA/NASA/SOHO/K. Battams)
- An animation based on the SOHO data is available here.
- Author of the discovery is Trygve Prestgard, who recently completed a Master’s degree in geophysics from Université Grenoble Alpes in France.
- Like most other SOHO-discovered comets, SOHO-4000 is part of the Kreutz family of sungrazers. The Kreutz family of comets all follow the same general trajectory, one that carries them skimming through the outer atmosphere of the Sun.
- “I feel very fortunate to have found SOHO’s 4,000th comet. Although I knew that SOHO was nearing its 4,000th comet discovery, I did not initially think that this sungrazer would be it,” said Trygve, who first spotted the comet in SOHO’s data. “It was only after discussing with other SOHO comet hunters, and counting through the most recent sungrazer discoveries, that the idea sunk in. I am honored to be part of such an amazing collaborative effort.”
- Like most who have discovered comets in SOHO’s data, Trygve is a citizen scientist, searching for comets in his free time with the Sungrazer Project – a NASA-funded citizen science project, managed by Karl Battams from the US Naval Research Lab, which grew out of comet discoveries by citizen scientists early into SOHO’s mission.
- “I have been actively involved in the Sungrazer Project for about eight years. My work with sungrazers is what solidified my long-term interest in planetary science,” said Trygve, who has discovered around 120 previously-unknown comets using data from SOHO and NASA’s STEREO mission. “I enjoy the feeling of discovering something previously unknown, whether this is a nice “real time” comet or a “long-gone” overlooked one in the archives.”
- Launched in 1995, SOHO studies the Sun from its interior to its outer atmosphere, with an uninterrupted view from its vantage point between the Sun and Earth, about a million miles from our planet. But over the past two and half decades, SOHO has also become the greatest comet finder in human history.
- SOHO is a cooperative effort between ESA and NASA. Mission control is based at NASA’s Goddard Space Flight Center in Greenbelt, Maryland. SOHO’s LASCO (Large Angle and Spectrometric Coronagraph Experiment), which is the instrument that provides most of the comet imagery, was built by an international consortium, led by the US Naval Research Lab.
• May 13, 2020: Currently crossing the skies above Earth, Comet C/2020 F8 (SWAN) has the potential to become a more prominent naked eye object by late May or early June. Yet it wasn’t discovered by someone looking up at the night sky. Instead, the person was looking at a computer screen. 17)
- Amateur astronomer Michael Mattiazzo from Australia spotted this icy visitor from the outer Solar System while inspecting images that had been posted online from the Solar Wind ANisotropies (SWAN) instrument aboard SOHO, the ESA/NASA Solar and Heliospheric Observatory.
- SWAN captures images in ultraviolet light, including a specific ultraviolet wavelength called Lyman alpha. This is a wavelength that is characteristically emitted by hydrogen atoms. The instrument’s primary goal is to map changes in the solar wind, the variable flow of charged particles that is continuously released by the Sun into interplanetary space. In addition, it has become an effective discoverer of comets too because comets are also sources of hydrogen.

Figure 17: Spotting a comet in the all-sky maps from SOHO's instrument SWAN. An animation showing the movement of Comet C/2020 F8 (SWAN) through successive all-sky maps made by the Solar Wind ANisotropies (SWAN) instrument aboard the ESA/NASA Solar and Heliospheric Observatory (SOHO). The sequence covers the period from 1 April to 9 May 2020. The comet can be seen as a moving white blob on the left of the image, just below the middle line of the coordinate grid, indicated with an arrow starting from the 5 April map. The comet is small to start with but gradually grows bigger. It was using these maps that Australian amateur Michael Mattiazzo discovered the comet. Between 17 and 27 April, the comet disappears behind the black areas that identify regions of the sky too bright to be observed, but by 28 April it is clearly visible again towards the left edge of the map. When the comet leaves the left-hand side of the image, it reappears on the right-hand side after 2 May (the image represents the 360-degree sky mapping used by SWAN) (image credit: ESA/NASA/SOHO)
- In the case of a comet, the hydrogen comes from the water vapor the icy core releases into space when heated by the Sun. And there is more, as solar radiation can break water molecules (H2O) into a single hydrogen atom (H) and a hydrogen-oxygen pair (which scientists call a hydroxyl radical, or OH). The result is a cloud of hydrogen that surrounds the comet, giving off a bright spot of Lyman-alpha light that can be spotted in the SWAN maps.
- Almost every day, SWAN records a complete map of the sky. These raw sky maps are full of stars, making it difficult to pick out new comets, which may arrive at random from any direction. To make the job easier, successive maps are automatically subtracted from one another, removing the stars and leaving only variable or moving sources visible.
- These ‘difference images’ are regularly posted online on the SOHO website, meaning that anyone with internet access can look at these ‘comet tracker’s maps’ and join the search for new comets. To date, twelve of them have been spotted in the SWAN data since 1996, all of them by amateur astronomers, or citizen scientists as they are also known.
- In the case of this current comet, Mattiazzo (who has already discovered eight comets using this method) found it by comparing the SWAN maps from several days in early April 2020.
From discovery to observation
- Once the comet had been announced, Austrian astrophotographer Gerald Rhemann obtained a beautiful image of it from the desert in Namibia, clearly showing the spherical gas cloud of the comet’s coma and its extended ion tail. When the image was published as Astronomy Picture of the Day (APOD) on 29 April, it helped bring the comet to wide scale attention.
- Another image, taken a few days later by British astrophotographer Damian Peach using a remote telescope in Chile and also featured as APOD, portrays the impressive comet’s tail as it closed in on Earth. The closest approach is estimated for today, 13 May, at around 85 million km from our planet.
- The SWAN team’s comet expert, Michael Combi from the University of Michigan, estimates that by 15 April the comet was ejecting about 1300 kg of water vapor every second, or about 4.4 x 1028 H2O molecules every second. That is a fast rate of ejection when compared to other comets.
- “This is already three times more than Comet 67P/Churyumov-Gerasimenko at its best, when it was visited by ESA’s Rosetta mission between 2014 and 2016,” says Jean-Loup Bertaux, former principal investigator and proposer of the SWAN instrument.

Figure 18: Comet SWAN in all-sky map from SOHO. One of the images that was used by Australian amateur Michael Mattiazzo to discover Comet C/2020 F8 (SWAN). The image was taken by the Solar Wind ANisotropies (SWAN) instrument aboard the ESA/NASA Solar and Heliospheric Observatory (SOHO). It is a full-sky map showing differences in ultraviolet brightness between 10 and 11 April 2020. Most spots are just residuals of star signals that have not been completely eliminated in the subtraction process. They are easily discarded, however, because they stay fixed in the sky, while the comet (indicated by the labelled white arrow) changes position from day to day. On all SWAN maps, there are two large black areas. These are regions that are too bright to be observed. One is centered on the Sun (indicated here with a yellow star), the other on parts of the SOHO spacecraft that reflect sunlight. These areas are often surrounded by bright areas, because the stray light generated by the sun shade and SOHO is variable and so cannot be fully eliminated by the difference process (image credit: ESA/NASA/SOHO)
Will Comet SWAN become an obvious naked eye object?
- The comet’s vigor could be significant for observers on Earth. The more material ejected from the comet, the more sunlight it reflects and the more visible it becomes. Currently moving from the southern to the northern skies, it is just faintly visible to the naked eye, but current estimates suggest that, by the end of May, it could be significantly brighter – if it survives that long.
- Comets are fragile objects, and can often break apart as they approach the Sun. In late April, the much anticipated Comet ATLAS suffered this fate, breaking into at least 30 fragments. Comet SWAN is now entering the ‘danger zone’ and will reach its closest point to the Sun on 27 May – at this time, the solar heating will be at its maximum.
- It can be extremely difficult to predict the behavior of comets that make such close approaches to the Sun, but scientists are hopeful that Comet SWAN will remain bright enough to see as it continues its journey. If the comet survives, star gazers on Earth should look for it near the bright star Capella in the constellation of Auriga, the Charioteer. This is almost certainly the only time the comet will be visible in our lifetimes: estimates are not yet fully precise, but it is clear that the comet’s orbital period is measured in thousands or even millions of years.
Waiting for the 4000th comet
- Although Comet SWAN is only the 12th discovery from that particular instrument, it is the 3932nd comet discovered by SOHO.
- “Almost all of SOHO's comet discoveries so far have been made by citizen scientists scouring images returned by SOHO's LASCO instrument,” says Karl Battams, LASCO team comet expert at the US Naval Research Laboratory and lead researcher of the Sungrazer Project.
- “It’s extremely exciting that our sun-watching observatory has spotted so many comets since its launch in 1995,” says Bernhard Fleck, ESA SOHO project scientist. “We are eagerly awaiting, along with comet enthusiasts around the world, for the 4000th discovery, which might happen real soon.”
Note: The SWAN instrument on the SOHO mission was built in cooperation between Service d’Aéronomie (now, LATMOS) and the Finnish Meteorological Institute in Helsinki. It is still fully operational and since 1996 has observed the changing Lyman-alpha sky pattern over more than two full solar cycles.
• March 25, 2019: Last Wednesday (20 March), all locations on our planet enjoyed roughly the same number of hours of day and night. This event, called an equinox, takes place twice a year – around 20 March and then again around 23 September. 18)
- On these two occasions along Earth’s yearly orbit around the Sun, sunlight shines directly overhead at the equator. The March equinox marks the beginning of spring in the northern hemisphere and of autumn in the southern one, and vice versa for the September equinox.
- The ESA/NASA SOHO solar observatory enjoys an alternative view of our parent star, staring at the Sun since 1995 from a vantage position – orbiting the first Lagrange point (L1) some 1.5 million kilometers from Earth towards the Sun. Over the years, SOHO has been monitoring the surface and stormy atmosphere of our star, as well as keeping an eye on the solar wind, the flow of charged particles streaming out through the Solar System, enabling a wealth of scientific discoveries.

Figure 19: This montage of images shows SOHO’s view of the Sun at different ultraviolet wavelengths in the early morning of 20 March; the equinox occurred at 21:58 GMT. From left to right, the images shown in this view were taken at increasing wavelengths (171 Å, 195 Å, 284 Å and 304 Å, respectively) with SOHO’s EIT (Extreme-ultraviolet Imaging Telescope), which currently takes snapshots of the Sun twice a day. Each wavelength channel is sensitive to solar material at a different range of temperatures, peering at different heights into the Sun’s atmosphere. From left to right, the brightest material in each image corresponds to temperatures of 1 million, 1.5 million, 2 million and 60,000–80,000ºC, respectively. (image credit: ESA/NASA, SOHO)
- Similar SOHO views of the Sun were also featured in a previous Space Science Image of the Week in 2017. Look at them side by side to spot any differences between the Sun then and now. For more information about SOHO, including realtime images of the Sun, visit: https://soho.nascom.nasa.gov
- Meanwhile, at the IABG facilities in Ottobrunn, Germany, the Solar Orbiter spacecraft is undergoing final testing ahead of launch early next year. This new joint ESA/NASA mission will perform unprecedented close-up observations of the Sun from a unique orbit that will allow scientists to study our star and its corona in much more detail than previously possible, as well as providing high-resolution images of the uncharted polar regions of the Sun.
• February 20, 2019: The outermost part of our planet’s atmosphere extends well beyond the lunar orbit – almost twice the distance to the Moon. A recent discovery based on observations by the ESA/NASA SOHO (Solar and Heliospheric Observatory) mission, shows that the gaseous layer that wraps around Earth reaches up to 630,000 km away, or 50 times the diameter of our planet. 19) 20) 21)
- “The Moon flies through Earth’s atmosphere,” says Igor Baliukin of Russia’s Space Research Institute, lead author of the paper presenting the results. “We were not aware of it until we dusted off observations made over two decades ago by the SOHO spacecraft.”
- Where our atmosphere merges into outer space, there is a cloud of hydrogen atoms called the geocorona. One of the spacecraft instruments, SWAN (Solar Wind Anisotropies), used its sensitive sensors to trace the hydrogen signature and precisely detect how far the very outskirts of the geocorona are.
- These observations could be done only at certain times of the year, when the Earth and its geocorona came into view for SWAN.
- For planets with hydrogen in their exospheres, water vapor is often seen closer to their surface. That is the case for Earth, Mars and Venus.
- “This is especially interesting when looking for planets with potential reservoirs of water beyond our Solar System,” explains Jean-Loup Bertaux, co-author and former principal investigator of SWAN.

Figure 20: Where Earth’s atmosphere merges into outer space, there is a cloud of hydrogen atoms called the geocorona. A recent discovery based on observations by the ESA/NASA SOHO (Solar and Heliospheric Observatory) mission shows, that the geocorona extends well beyond the orbit of the Moon, reaching up to 630,000 km above Earth’s surface, or 50 times the diameter of our planet. Note: the illustration is not to scale (image credit: ESA)
- The first telescope on the Moon, placed by Apollo 16 astronauts in 1972, captured an evocative image of the geocorona surrounding Earth and glowing brightly in ultraviolet light. “At that time, the astronauts on the lunar surface did not know that they were actually embedded in the outskirts of the geocorona,” says Jean-Loup.

Figure 21: The Earth and its hydrogen envelope, or geocorona, as seen from the Moon. This ultraviolet picture was taken in 1972 with a camera operated by Apollo 16 astronauts on the Moon (image credit: NASA)
Cloud of hydrogen
- The Sun interacts with hydrogen atoms through a particular wavelength of ultraviolet light called Lyman-alpha, which the atoms can both absorb and emit. Since this type of light is absorbed by Earth’s atmosphere, it can only be observed from space.
- Thanks to its hydrogen absorption cell, the SWAN instrument could selectively measure the Lyman-alpha light from the geocorona and discard hydrogen atoms further out in interplanetary space.

Figure 22: The intensity of hydrogen atom emission in the outermost part of Earth’s atmosphere, the geocorona, as measured by the SWAN instrument on board the ESA/NASA SOHO mission. Low intensity is indicated in blue, high intensity in red. The data revealed that the geocorona extends well beyond the orbit of the Moon, reaching up to 630,000 km above Earth’s surface, or 50 times the diameter of our planet. Earth is located at the center of the white area, too small to be visible at this scale; the extent of the Moon’s orbit around Earth is indicated as a dotted ellipse for reference [image credit: ESA/NASA/SOHO/SWAN; I. Baliukin et al (2019)]
- The new study revealed that sunlight compresses hydrogen atoms in the geocorona on Earth’s dayside, and also produces a region of enhanced density on the night side. The denser dayside region of hydrogen is still rather sparse, with just 70 atoms/cm3 at 60,000 km above Earth’s surface, and about 0.2 atoms at the Moon’s distance.
- “On Earth we would call it vacuum, so this extra source of hydrogen is not significant enough to facilitate space exploration,” says Igor.
- The good news is that these particles do not pose any threat for space travelers on future crewed missions orbiting the Moon.
- “There is also ultraviolet radiation associated to the geocorona, as the hydrogen atoms scatter sunlight in all directions, but the impact on astronauts in lunar orbit would be negligible compared to the main source of radiation – the Sun,” says Jean-Loup Bertaux.
- On the down side, the Earth’s geocorona could interfere with future astronomical observations performed in the vicinity of the Moon.
- “Space telescopes observing the sky in ultraviolet wavelengths to study the chemical composition of stars and galaxies would need to take this into account,” adds Jean-Loup.
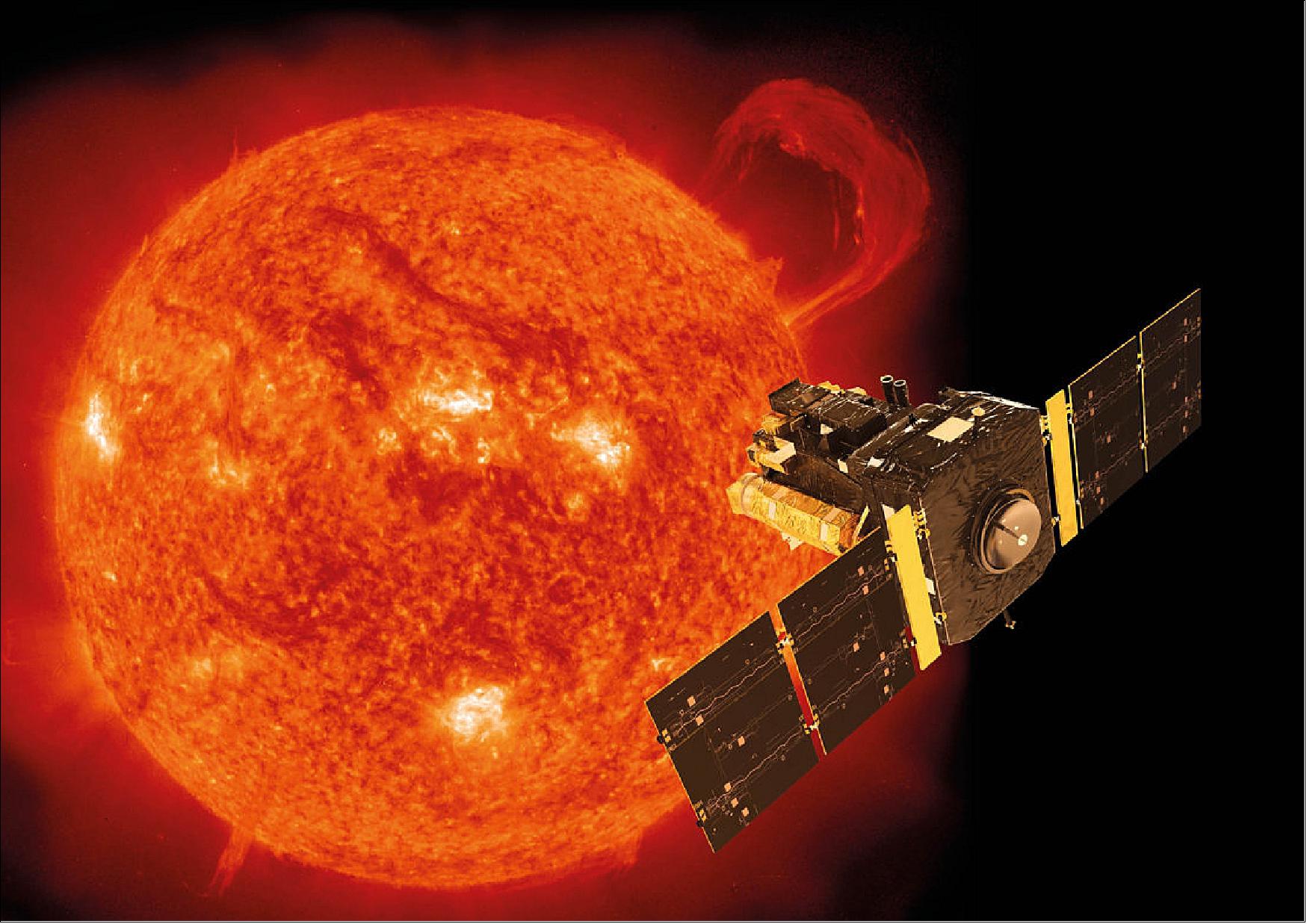
Figure 23: Artist’s impression of the ESA/NASA SOHO spacecraft with the Sun as seen by the satellite’s extreme-ultraviolet imaging telescope on 14 September 1999 (image credit: Spacecraft: ESA/ATG medialab; Sun: ESA/NASA SOHO, CC BY-SA 3.0 IGO)
The power of archives
- Launched in December 1995, the SOHO space observatory has been studying the Sun, from its deep core to the outer corona and the solar wind, for over two decades. The satellite orbits around the first Lagrange point (L1), some 1.5 million km from Earth towards the Sun.
- This location is a good vantage point to observe the geocorona from outside. SOHO’s SWAN instrument imaged Earth and its extended atmosphere on three occasions between 1996 and 1998.
- Jean-Loup and Igor’s research team in Russia decided to retrieve this data set from the archives for further analysis. These unique views of the whole geocorona as seen from SOHO are now shedding new light on Earth’s atmosphere.
- “Data archived many years ago can often be exploited for new science,” says Bernhard Fleck, ESA SOHO project scientist. “This discovery highlights the value of data collected over 20 years ago and the exceptional performance of SOHO.”
• The 2nd of December 2018 marks the 23rd launch anniversary of the SOHO (Solar and Heliospheric Observatory) mission. SOHO is the longest-lived heliophysics mission still operating and has provided a nearly continuous record of solar and heliospheric phenomena over a full 23-year magnetic cycle (two 11-year sunspot cycles). SOHO's findings have been documented in over 5,000 papers in the peer reviewed literature, authored by more than 4,000 scientists worldwide. 22)
- SOHO provided the first ever images of structures and flows below the Sun's surface and of activity on the far side of the Sun. SOHO discovered sunquakes and eliminated uncertainties in the internal structure of the Sun as a possible explanation for the "neutrino problem" which concerned the large discrepancy between the high flux of solar neutrinos — particles which are now believed to possess mass and travel at almost the speed of light — predicted from the Sun's luminosity and the much lower flux that is observed.
- The ultraviolet imagers and spectrometers on SOHO have revealed an extremely dynamic solar atmosphere where plasma flows play an important role and discovered dynamic solar phenomena such as coronal waves. SOHO measured the acceleration profiles of both the slow and fast solar wind and identified the source regions of the fast solar wind.
- SOHO revolutionized our understanding of solar-terrestrial relations and dramatically boosted space weather forecasting capabilities by providing, in a near-continuous stream, a comprehensive suite of images covering the dynamic atmosphere and extended corona. SOHO has measured and characterized over 28,000 coronal mass ejections (CMEs). CMEs are the most energetic eruptions on the Sun and the major driver of space weather. They are responsible for all of the largest solar energetic particle events in the heliosphere and are the primary cause of major geomagnetic storms. SOHO's visible-light CME measurements are considered a critical part of the US National Space Weather Action Plan.
- For two solar activity cycles SOHO has measured the total solar irradiance (the "solar constant") as well as variations in the extreme ultraviolet flux, both of which are important to understand the impact of solar variability on Earth's climate. Besides watching the Sun, SOHO has become the most prolific discoverer of comets in astronomical history: as of late 2018, more than 3,400 comets have been found by SOHO, most of them by amateurs accessing SOHO real-time data via the Internet.

Figure 24: SOHO,the ESA-NASA cooperative solar mission with 'pick of the week image' (image credit: ESA)
Extended life for ESA's Science Missions
• November 14, 2018: The SPC (Science Program Committee) of ESA has confirmed the continued operations of ten scientific missions in the Agency's fleet up to 2022. After a comprehensive review of their scientific merits and technical status, the SPC has decided to extend the operation of the five missions led by ESA's Science Program: Cluster, Gaia, INTEGRAL, Mars Express, and XMM-Newton. The SPC also confirmed the Agency's contributions to the extended operations of Hinode, Hubble, IRIS, SOHO, and ExoMars TGO. 23)
- This includes the confirmation of operations for the 2019–2020 cycle for missions that had been given indicative extensions as part of the previous extension process, and indicative extensions for an additional two years, up to 2022.
Note: Every two years, all missions whose approved operations end within the following four years are subject to review by the advisory structure of the Science Directorate. Extensions are granted to missions that satisfy the established criteria for operational status and science return, subject to the level of financial resources available in the science program. These extensions are valid for the following four years, subject to a mid-term review and confirmation after two years.
- The decision was taken during the SPC meeting at ESA/ESAC (European Space Astronomy Center) near Madrid, Spain, on 14 November.
- ESA's science missions have unique capabilities and are prolific in their scientific output. Cluster, for example, is the only mission that, by varying the separation between its four spacecraft, allows multipoint measurements of the magnetosphere in different regions and at different scales, while Gaia is performing the most precise astrometric survey ever realized, enabling unprecedented studies of the distribution and motions of stars in the Milky Way and beyond.
- Many of the science missions are proving to be of great value to pursue investigations that were not foreseen at the time of their launch. Examples include the role of INTEGRAL and XMM-Newton in the follow-up of recent gravitational wave detections, paving the way for the future of multi-messenger astronomy, and the many discoveries of diverse exoplanets by Hubble.
- Collaboration between missions, including those led by partner agencies, is also of great importance. The interplay between solar missions like Hinode, IRIS and SOHO provides an extensive suite of complementary instruments to study our Sun; meanwhile, Mars Express and ExoMars TGO are at the forefront of the international fleet investigating the Red Planet.
- Another compelling factor to support the extension is the introduction of new modes of operation to accommodate the evolving needs of the scientific community, as well as new opportunities for scientists to get involved with the missions.23)
• November 5, 2018: This week, to coincide with the fifteenth annual European Space Weather Week, ESA is celebrating the dynamic phenomenon of space weather. 24)
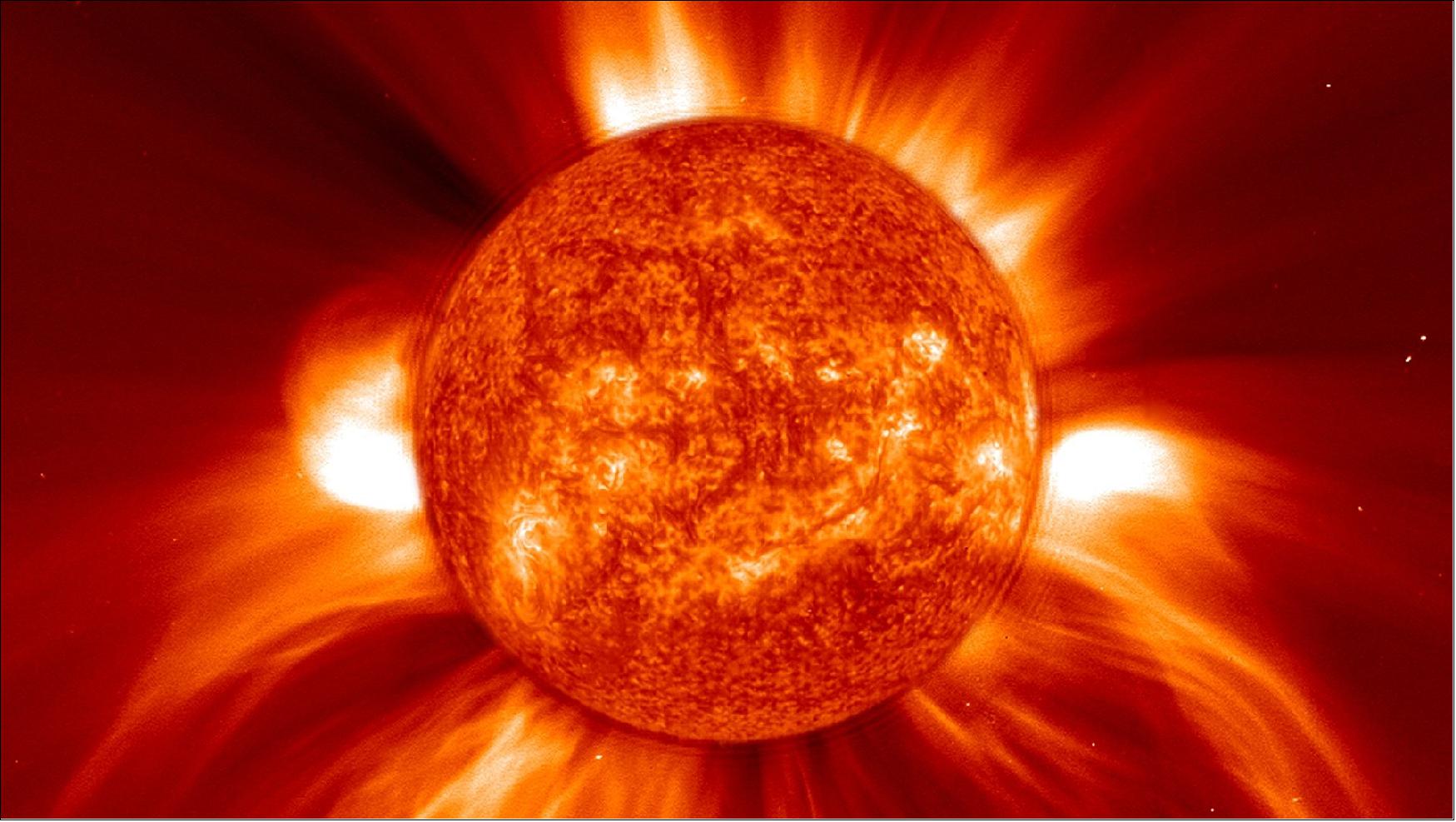
Figure 25: This image, acquired by the LASCO C2 coronagraph instrument on the ESA-NASA SOHO spacecraft, was taken 8 January 2002 and shows a widely spreading coronal mass ejection (CME) as it blasts more than a billion tons of matter out into space at millions of kilometers per hour (image credit: ESA/NASA/SOHO)
Legend to Figure 25: This C2 image was turned 90 degrees so that the blast seems to be pointing down. A second image, from the EIT disk imager on a different day was enlarged and superimposed on the C2 image so that it filled the occulting disk for effect.
- We’re taking a closer look at space weather, the ever-present solar wind and the ever-changing cycles of the Sun — a star that sees 11 years pass in relative calm followed by another 11 years of immense activity, driving sunspots, solar flares, Coronal Mass Ejections and solar-particle events.
- It’s difficult to comprehend the size and sheer power of our Sun — a churning ball of hot gas 4.6 billion years old and 1.3 million times larger than Earth — that for the most part remains a regular, yet distant part of our lives.
- In space, however, this hotly glowing star plays a remarkable role – dominating our solar system. Unpredictable and temperamental, the Sun has made life on the inner planets of the Solar System, save one exception, impossible, due to the intense radiation combined with the colossal amounts of energetic material it blasts in every direction, creating the ever-changing conditions in space known as ‘space weather’.
- Considering all of this, how did life come to thrive on Earth? Our magnetic field protects us from the solar wind, a constant stream of electrons, protons and heavier particles – 'ions' – from the Sun, and from Coronal Mass Ejections (CMEs), the Sun’s occasional outbursts of multi-billion-ton clouds of solar plasma into space. The most extreme events, arrivals of fast CMEs or high-speed solar wind streams, disturb our protective magnetic shield, creating geomagnetic storms.
- Serious problems for modern life: These storms have the potential to cause serious problems for modern technological systems, disrupting or damaging satellites in space and the multitude of services – like navigation and telecoms – that rely on them, or blacking out power grids and radio communication and creating a radiation hazard for astronauts in space.
- Watching for solar hazards: While these events can’t be stopped, advance warning of an oncoming solar storm would give spacecraft controllers and power-grid and network operators time to take protective measures.
- ESA’s planned Lagrange mission will support provision of just such advance warnings. Watching the Sun from a unique position in space, the Lagrange satellite will allow monitoring of the potentially hazardous sunspots and high-speed solar wind streams before they come into view from Earth, and detect solar events and their propagation toward the Earth with higher accuracy than is possible today. Data from the Lagrange mission will be transmitted to the Earth and distributed into ESA’s Space Weather Service Network in near real-time to generate warnings and forecasts.
- Protective measures against space weather are becoming increasingly important, as much of modern human society becomes increasingly reliant on space-based services, vulnerable to the Sun’s unpredictable outbursts.
- As a result, at ESA's next Ministerial Council in 2019, space weather and the needed early warning services will be a main topic presented as part of the Agency's vision for the future in the emerging domain of space safety and security.
- This week, ESA will highlight the unique phenomenon of space weather, from the science behind it and how we study it, to its effect on satellites in space and ESA’s plans for the future.
• February 26, 2018: The activity cycle of the Sun – where the number of sunspots increase and decrease – has been monitored regularly for around 250 years, but the use of space-based telescopes has given us a whole new perspective of our nearest star.
- On 22 December 2017, SOHO (Solar and Heliospheric Observatory) reached 22 years in space. That duration is significant because it is the average length of the complete solar magnetic cycle. Sunspot cycles are known to occur over about 11 years, but the full cycle is double this length owing to the behavior of the magnetic fields. The Sun’s polarity gradually changes through its cycle, so that after 11 years the orientation of the field will have flipped between the northern and southern hemispheres. At the end of a 22-year cycle, the orientation of the magnetic field is the same as it was at the start. 25)
- Each image of Figure 26 is a snapshot of the Sun taken every spring with the EIT (Extreme ultraviolet Imaging Telescope) on SOHO. Observing in the ultraviolet reveals the Sun’s corona – the extremely hot atmosphere, up to some 2 million degrees, that extends millions of kilometers into space.
- When the Sun is at its most active, strong magnetic fields show up as bright spots in the ultraviolet images of the corona. Activity also becomes obvious on the photosphere, which is the surface we see in visible light.
- When the Sun is active, sunspots appear on the surface. Concentrations of magnetic fields can reduce the surface temperature in some areas and this reduced temperature makes these areas appear black in visible light images. The last 11-year cycle began in 1996, and the current one started in 2008, with solar maximum occurring in 2014.
- By monitoring the Sun for almost a complete 22-year cycle, SOHO has provided a wealth of data on solar variability. This has been vital for monitoring the interaction of the Sun’s activity with Earth, and improving capabilities in space weather forecasting.
- SOHO has made many important discoveries with its suite of instruments, such as revealing the existence of sunquakes, detecting waves travelling through the corona and identifying the source of the ‘fast’ solar wind.
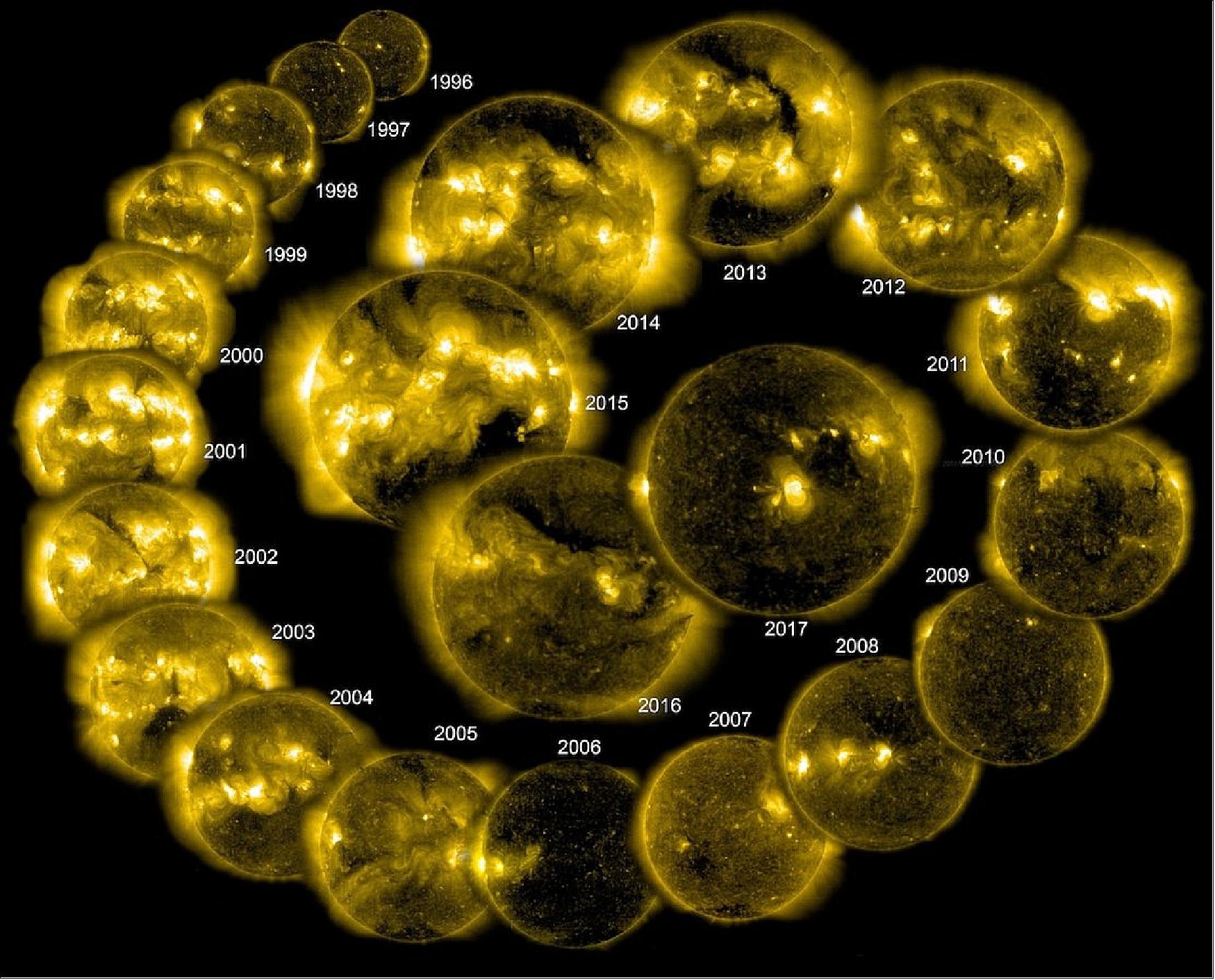
Figure 26: SOHO 22 years in space - the average length of a complete solar magnetic cycle. It takes 11 years for the orientation of the field to flip between the northern and southern hemisphere, and another 11 years to flip back to its original orientation. (image credit: SOHO (ESA & NASA))
• December 7, 2017: ESA's SPC (Science Program Committee) has approved indicative extensions, up to 2019-2020, for the operation of eight scientific missions. 26)
- During its meeting at ESA Headquarters in Paris, on 21-22 November, the SPC approved indicative extensions for the continued operation of five ESA-led missions: Gaia, INTEGRAL, Mars Express, SOHO, and XMM-Newton. This followed a comprehensive review of the current operational status and outlook of the missions and their expected scientific returns during the extension period. The decision will be subject to confirmation towards the end of 2018.
- The lifetime of Gaia, ESA's billion star surveyor, was extended by eighteen months, from 25 July 2019 to 31 December 2020. This is the first time that Gaia, which was launched in 2013 and originally funded for a five-year mission, has been subject to the extension process. — Mars Express, SOHO, and XMM-Newton each received extensions of two years, so their operations will continue at least until the end of 2020.
- The SPC extended the operations of the high-energy observatory INTEGRAL by one year, until 31 December 2019. A proposal to extend INTEGRAL until the end of 2020, as well as a proposal concerning a two-year extension of the magnetospheric plasma mission, Cluster, will be presented to the next meeting of the SPC in February 2018.
- The go-ahead was also given to continue ESA's contributions to the operations of three international collaborative missions: the HST (Hubble Space Telescope), and IRIS (Interface Region Imaging Spectrograph), which are both led by NASA, as well as the Japanese-led mission Hinode.
- Every two years, all missions whose approved operations end within the following four years are subject to review by the advisory structure of the Science Directorate. Extensions are granted to missions that satisfy the established criteria for operational status and science return, subject to the level of financial resources available in the science program. These extensions are valid for the following four years, subject to a mid-term review and confirmation after two years. Extensions for operations in the period 2017-2018 were approved by the SPC in November 2016, but the indicative extension, for 2019-2020, had been deferred until the November 2017 meeting to allow the SPC to evaluate the outcome of the ESA Ministerial Council meeting in December 2016.
• November 3, 2017: The SOHO mission of ESA and NASA got a visit from an old friend this week when comet 96P entered its field of view on Oct. 25, 2017. The comet entered the lower right corner of SOHO’s view, and skirted up and around the right edge before leaving on Oct. 30. SOHO also spotted comet 96P in 1996, 2002, 2007 and 2012, making it the spacecraft’s most frequent cometary visitor. 27)

Figure 27: The comet entered the lower right corner of SOHO’s view, and skirted up and around the right edge before leaving on Oct. 30. Jupiter can be seen passing left-to-right behind the solid central disk — called an occulting disk — that blocks sunlight and allows SOHO to see the solar atmosphere, planets and comets (image credit: ESA/NASA’s Goddard Space Flight Center/SOHO/NRL/Karl Battams/Joy Ng)
- At the same time, comet 96P passed through a second NASA mission’s view: STEREO (Solar and Terrestrial Relations Observatory ) also watched the comet between Oct. 26-28, from the opposite side of Earth’s orbit. It is extremely rare for comets to be seen simultaneously from two different locations in space, and these are the most comprehensive parallel observations of comet 96P yet. Scientists are eager to use these combined observations to learn more about the comet’s composition, as well as its interaction with the solar wind, the constant flow of charged particles from the Sun.
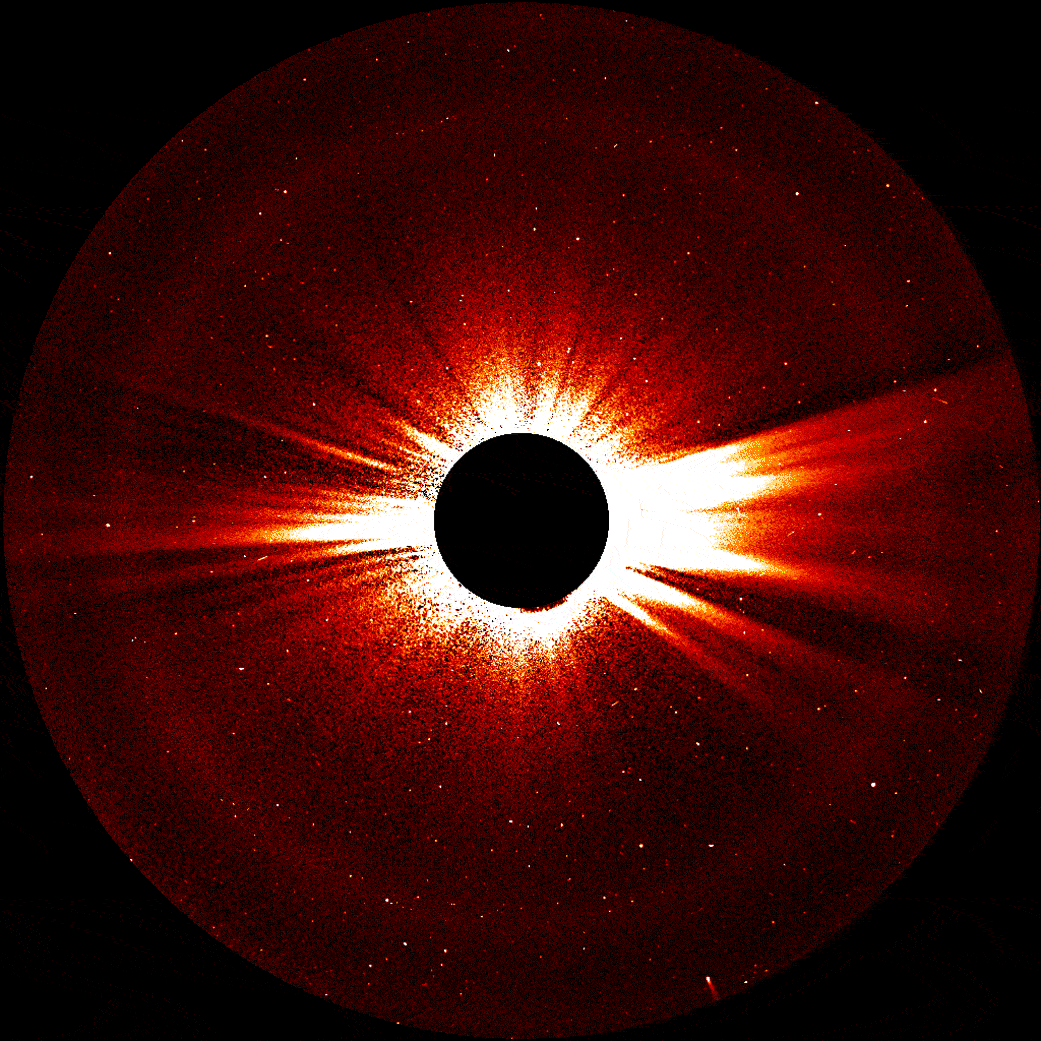
Figure 28: The comet entered the bottom of STEREO’s view and crossed it diagonally before leaving on Oct. 28. Most of the corona has been suppressed in order to bring out the comet, leaving only the dynamic flow of the solar wind (image credit: NASA’s Goddard Space Flight Center/STEREO/Bill Thompson/Joy Ng)
- Both missions gathered polarization measurements of the comet; these are measurements of sunlight in which all the light waves become oriented the same way after passing through a medium — in this case, particles in the tail of the comet. By pooling the polarization data together, scientists can extract details on the particles that the light passed through.
- “Polarization is a strong function of the viewing geometry, and getting multiple measurements at the same time could potentially give useful information about the composition and size distribution of the tail particles,” said William Thompson, STEREO chief observer at NASA/GSFC (Goddard Space Flight Center) in Greenbelt, Maryland.
- Comet 96P — also known as comet Machholz, for amateur astronomer Dan Machholz’s 1986 discovery of the comet — completes an orbit around the Sun every 5.24 years. It makes its closest approach to the Sun at a toasty 11 million miles — a very close distance for a comet.
- When comet 96P appeared in SOHO’s view in 2012, amateur astronomers studying the SOHO data discovered two tiny comet fragments some distance ahead of the main body, signaling the comet was actively changing. This time around they have detected a third fragment — another breadcrumb in the trail that indicates the comet is still evolving.
- Scientists find comet 96P interesting because it has an unusual composition and is the parent of a large, diverse family, referring to a group of comets sharing a common orbit and originating from a much larger parent comet that over millennia, broke up into smaller fragments. Comet 96P is the parent of two separate comet groups, both of which were discovered by citizen scientists studying SOHO data, as well as a number of Earth-crossing meteor streams. By studying the comet’s ongoing evolution, scientists can learn more about the nature and origins of this complex family.
• August 01, 2017: Scientists using the ESA/NASA SOHO solar observatory have found long-sought gravity modes of seismic vibration that imply the Sun’s core is rotating four times faster than its surface. 28) 29)
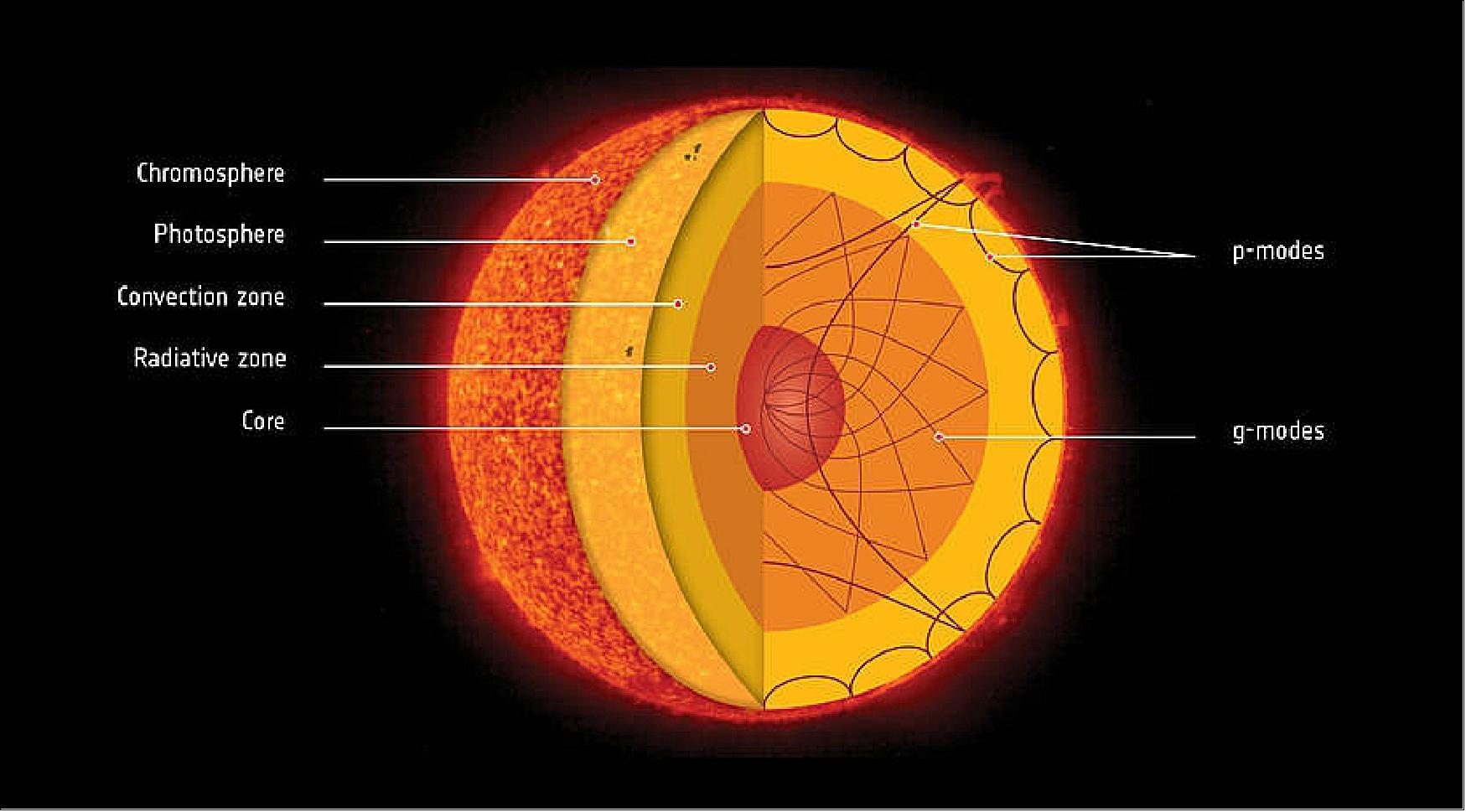
Figure 29: Illustration of the solar interior showing a type of wave called g-modes on the Sun. These g-modes reveal that the solar core is rotating about four times faster than the surface (image credit: ESA)
- Just as seismology reveals Earth’s interior structure by the way in which waves generated by earthquakes travel through it, solar physicists use ‘helioseismology’ to probe the solar interior by studying sound waves reverberating through it. On Earth, it is usually one event that is responsible for generating the seismic waves at a given time, but the Sun is continuously ‘ringing’ owing to the convective motions inside the giant gaseous body.
- Higher frequency waves, known as pressure waves (or p-waves), are easily detected as surface oscillations owing to sound waves rumbling through the upper layers of the Sun. They pass very quickly through deeper layers and are therefore not sensitive to the Sun’s core rotation.
- Conversely, lower frequency gravity waves (g-waves) that represent oscillations of the deep solar interior have no clear signature at the surface, and thus present a challenge to detect directly.
- In contrast to p-waves, for which pressure is the restoring force, buoyancy (gravity) acts as the restoring force of the gravity waves.
- “The solar oscillations studied so far are all sound waves, but there should also be gravity waves in the Sun, with up-and-down, as well as horizontal motions like waves in the sea,” says Eric Fossat, lead author of the paper describing the result, published in Astronomy & Astrophysics. 30)
- “We’ve been searching for these elusive g-waves in our Sun for over 40 years, and although earlier attempts have hinted at detections, none were definitive. Finally, we have discovered how to unambiguously extract their signature.”
- Eric and his colleagues used 16.5 years of data collected by SOHO’s dedicated GOLF (Global Oscillations at Low Frequencies) instrument. By applying various analytical and statistical techniques, a regular imprint of the g-modes on the p-modes was revealed.
- In particular, they looked at a p-mode parameter that measures how long it takes for an acoustic wave to travel through the Sun and back to the surface again, which is known to be 4 hours 7 minutes. A series of modulations was detected in this p-mode parameter that could be interpreted as being due to the g-waves shaking the structure of the core.
- The signature of the imprinted g-waves suggests the core is rotating once every week, nearly four times faster than the observed surface and intermediate layers, which vary from 25 days at the equator to 35 days at the poles.
- “G-modes have been detected in other stars, and now thanks to SOHO we have finally found convincing proof of them in our own star,” adds Eric. “It is really special to see into the core of our own Sun to get a first indirect measurement of its rotation speed. But, even though this decades long search is over, a new window of solar physics now begins.”
- The rapid rotation has various implications, for example: is there any evidence for a shear zone between the differently rotating layers? What do the periods of the g-waves tell us about the chemical composition of the core? What implication does this have on stellar evolution and the thermonuclear processes in the core?
- “Although the result raises many new questions, making an unambiguous detection of gravity waves in the solar core was the key aim of GOLF. It is certainly the biggest result of SOHO in the last decade, and one of SOHO’s all-time top discoveries,” says Bernhard Fleck, ESA’s SOHO project scientist.
- ESA’s upcoming solar mission, Solar Orbiter will also ‘look’ into the solar interior but its main focus is to provide detailed insights into the Sun’s polar regions, and solar activity. Meanwhile ESA’s future planet-hunting mission, Plato, will investigate seismic activity in stars in the exoplanet systems it discovers, adding to our knowledge of relevant processes in Sun-like stars.
• June 26, 2017: Residents of Earth’s northern hemisphere enjoyed the greatest number of daylight hours in a single day all year on 21 June 2017. This summer solstice occurs when the tilt of Earth’s axis is most inclined towards the Sun, which sits directly over the Tropic of Cancer.
- The ESA/NASA SOHO solar observatory provided an alternative view. It has been staring at the Sun since 1995, studying its interior, monitoring its surface and stormy atmosphere, and how the ‘solar wind’ blows through the Solar System. 31)

Figure 30: The montage of images shows SOHO’s view of the Sun at different ultraviolet wavelengths in the early morning of 21 June, 2017, corresponding to solar material at a range of temperatures (image credit: SOHO, ESA & NASA)
- From left to right of Figure 30, the brightest material in each image corresponds to temperatures of 60,000–80,000ºC, 1 million, 1.5 million and 2 million degrees, respectively. The higher the temperature, the higher you are looking in the solar atmosphere. The hottest areas appear brighter, while the darker regions are relatively cooler.
- Back on Earth, the Sun is now beginning to trace a lower path through the sky each day. Winter solstice occurs in six months’ time, 21 December, when Earth’s axis is tilted furthest away from the Sun. With the Sun directly over the Tropic of Capricorn, this results in the shortest number of daylight hours in the northern hemisphere. The situation is reversed for the southern hemisphere, where 21 June marks the winter solstice, and 21 December the summer solstice.
Figure 31: ESA's SOHO Mission to the Sun - Space Documentary. SOHO is a spacecraft built by a European industrial consortium led by Matra Marconi Space that was launched on a Lockheed Martin Atlas II AS launch vehicle on December 2, 1995 to study the Sun, and has discovered over 3000 comets (video credit: ESA, Published on Dec 27, 2016)
• December 12, 2016: While this scene (Figure 32) looks like the mesmerizing result of shaking up a festive snow globe, it is in fact the disturbing effect of one of the most powerful solar storms ever recorded. Over two weeks in October and November 2003 the Sun was unprecedentedly active, with giant sunspots – over 10 times the diameter of Earth – generating flares on an almost daily basis. 32)
- Solar flares are classed according to the energy they release at X-ray wavelengths. There are five major categories: A, B, C, M and X, further divided into 10 subclasses. M1 flares are 10 times more powerful than C1, and X1 flares are 10 times more powerful than M1 flares, or 100 times more powerful than C1.
- Some of the flares witnessed in this two-week period were so powerful they broke right through the top of the X-class range, which is usually given as X10. A flare erupting on 4 November was estimated to have reached at least X28.
- The ESA/NASA SOHO (Solar and Heliospheric Observatory) mission, launched in 1995 and still operating today, was monitoring the Sun’s stormy behavior during this time. This image shows its detectors being completely swamped by high-energy protons that were accelerated to nearly the speed of light (300,000 km/s) in the X17 flare of 28 October 2003.
- When Earth is in the firing line of associated CMEs (Coronal Mass Ejections), it can lead to beautiful and bright auroras in the atmosphere, giving unparalleled insight into the interaction of the Sun and Earth. CMEs can also cause serious disruption to radio communications, air traffic control and power grids.
- Although these powerful storms reveal the extremes of the Sun’s activity, fortunately for Earth, those on the scale of the 2003 events do not occur very often.
- The image was taken by SOHO’s LASCO C3 instrument. A special disc (indicated by the large blue circle) inside the instrument blocks the Sun (indicated by the inner white circle), so that details of the extended outer solar atmosphere can be observed. Watch a movie of the event depicted in this scene, here.
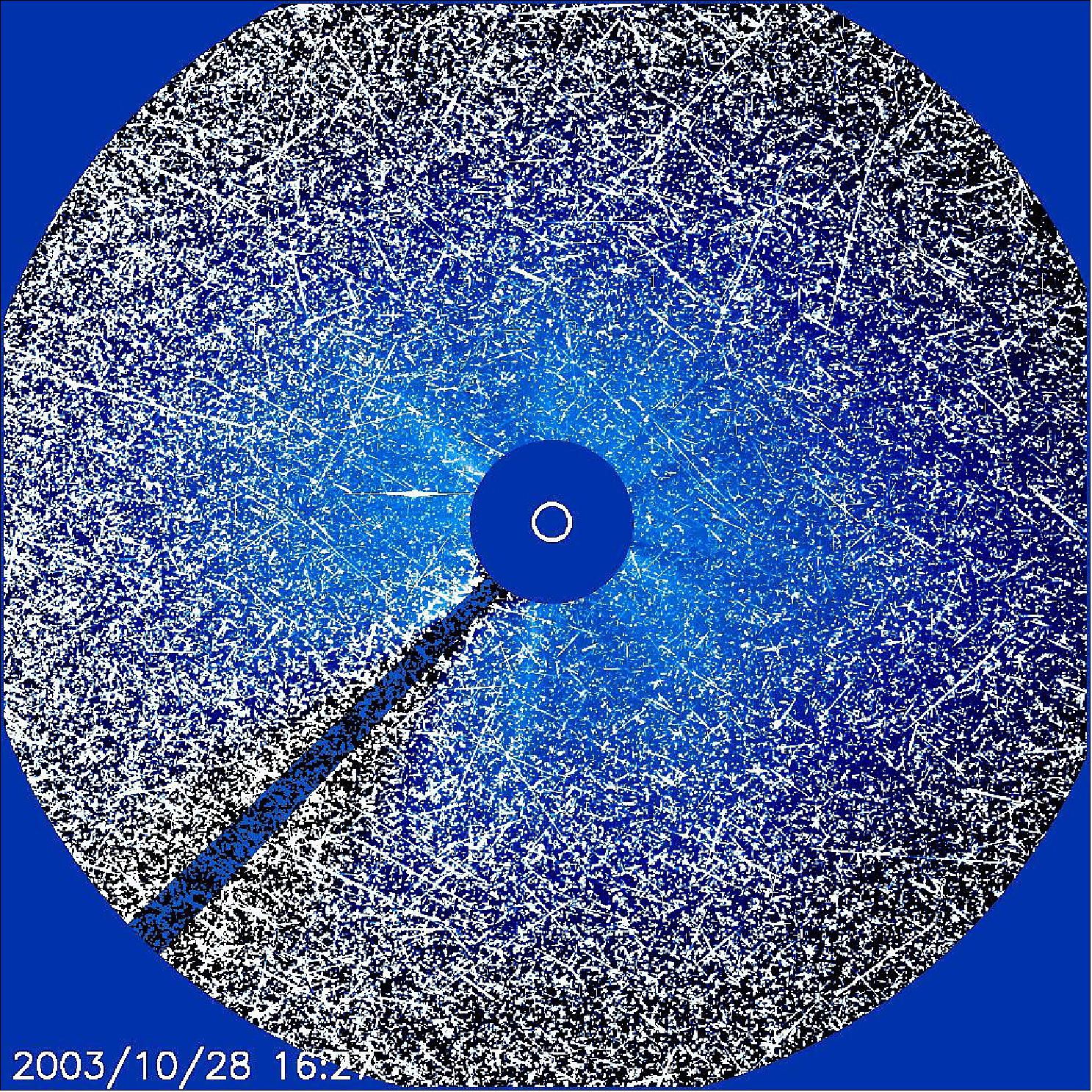
Figure 32: This snowstorm-like scene is the result of a most powerful solar flare swamping the Sun-watching SOHO satellite. The scene was observed on October 28, 2003 with the LASCO C3 instrument (image credit: SOHO, ESA & NASA)
• On December 2, 2016, the SOHO mission was 21 years on orbit.
• November 22, 2016: ESA's Science Program Committee (SPC) has today confirmed two-year mission extensions for nine scientific missions in which the Agency is participating. This secures their operations until the end of 2018. — After a comprehensive review of their current operational status and the likely scientific return from each mission, the SPC decided to extend the operation of six ESA-led missions (Cluster, INTEGRAL, Mars Express, PROBA-2, SOHO and XMM-Newton) from 1 January 2017 to 31 December 2018. 33)
- The go-ahead was also given to continue ESA's contributions to the operations of three international collaborative missions: the Hubble Space Telescope and the Interface Region Imaging Spectrograph (IRIS), which are both led by NASA, as well as Solar-B (Hinode), which is a Japanese-led mission.
• June 2016: ESA’s Science Program Committee has approved seven mission extensions. SOHO operations are currently approved up to December 2018, subject to mid-term review in 2016. Four of SOHO’s large number of remote sensing instruments have been turned off or have been hibernated in the past years, after they have been superseded by similar, improved instruments on more recent missions or because their detectors had worn out. 34)
- The MDI (Michelson Doppler Imager) instrument stopped taking science data on 12 April 2011, the UVCS (Ultraviolet Coronagraph Spectrometer) on 23 January 2013, the SUMER (Solar UV Measurement of Emitted Radiation) instrument on 8 August 2014, and the CDS (Coronal Diagnostic Spectrometer) on 5 September 2014. The EIT (Extreme-Ultraviolet Imaging Telescope), which has been superseded by the Atmospheric Imaging Assembly instrument on NASA’s SDO (Solar Dynamics Observatory), only takes two synoptic sets of images in four wavelengths per day in order to track detector behavior and to maintain the uniform dataset, which now spans nearly a full magnetic cycle (22 years).
- The other seven instruments are fully functional and continue to make unique contributions to the Heliophysics System Observatory. In addition to the unique and critically important white-light coronagraph observations from the Sun–Earth line by its LASCO (Large-Angle and Spectrometric Coronagraph), SOHO continues to provide vital measurements of total solar irradiance, low-frequency global solar oscillations, integrated extreme ultraviolet solar output, energetic particles and solar wind plasma parameters. The goal is to establish a unique long-term data record of these important parameters over a full 22-year magnetic cycle.
- Total and spectral solar irradiance (TSI and SSI) are key parameters in assessing the Sun’s influence on changes in Earth’s climate (Yeo et al. (2014) 35) reconstructed daily TSI and SSI measurements from 1974 to 2013 using data from MDI on SOHO, the Helioseismic and Magnetic Imager instrument on SDO and the Kitt Peak Vacuum Telescope. The reconstructed TSI model replicates 92% of the variability of the measured PMOD-WRC (Physikalisch-Metorologisches Observatorium Davos/World Radiation center) TSI composite, the last 19 years of which are based on measurements from the VIRGO (Variability of Solar Irradiance and Variability) instrument on SOHO (Figure 33). The consistency of reconstructed daily solar irradiance time series with observations from multiple sources demonstrates its validity for climate models. It also provides further evidence that photospheric magnetism is the prime driver of variations in solar irradiance on timescales greater than a day.

Figure 33: Composite of total solar irradiance measurements from 1975 to 2015 (image credit: (Physikalisch-Metorologisches Observatorium Davos / World Radiation Center)
Figure 34: Highlights from SOHO's 20 Years in Space. Dr. Joe Gurman of NASA's Goddard Space Flight Center provides commentary on selected shots from SOHO's 20 years in space (video credit: NASA Goddard, Published on Dec 1, 2015)
• On December 2, 2015, the collaborative SOHO mission of ESA and NASA, celebrated 20 years on orbit - observing the sun from L1 (Lagrangian Point 1). During its pioneering career it has returned a wealth of new information on the Sun’s deep core through to the hot and dynamic outer atmosphere, the solar wind and solar energetic particles. There aren’t a whole lot of 20th century spacecraft still in operation; SOHO joins the ranks of Hubble and the twin Voyager spacecraft as platforms from another era that have long exceeded their operational lives. 36) 37) 38) 39)
- Originally planned for a two-year mission, its numerous extensions have allowed it to cover nearly two 11-year solar cycles — the complete cycle 23 and already a large fraction of cycle 24. SOHO is thus the longest-lived Sun-watching mission. SOHO is a veritable Swiss Army Knife for solar astrophysics, not only monitoring the Sun across optical and ultraviolet wavelengths, but also employing the Michelson Doppler Imager to record magnetogram data and LASCO (Large Angle Spectrometric Coronograph) able to create an artificial solar eclipse and monitor the pearly white corona of the Sun.
- SOHO has revolutionized solar physics and the way we perceive our host star. Seeing inside the Sun - starquakes: Just as seismology reveals Earth’s interior by studying seismic waves from earthquakes, solar physicists use ‘helioseismology’ to probe the solar interior by studying the frequency and oscillations of sound waves reverberating through it. SOHO pioneered the new field of ‘local area helioseismology’, providing the first images of structures and gas flows below the Sun’s surface and even images of activity on the far side. It discovered ‘sunquakes’ and a slow subsurface current of gas flowing from the equator towards the poles. — Deeper inside the Sun, about a third of the way towards the center at the transition between its turbulent outer shell – the convection zone – and the more orderly radiative zone, SOHO found that the speed of the rotating gas changes abruptly. The measurements indicated that, near the equator, the outer layers rotate faster than the inner layers, while at mid-latitudes and near the poles the situation is reversed. This boundary region is of particular interest because it is where the solar dynamo that creates the Sun’s ever-changing magnetic field is believed to operate.
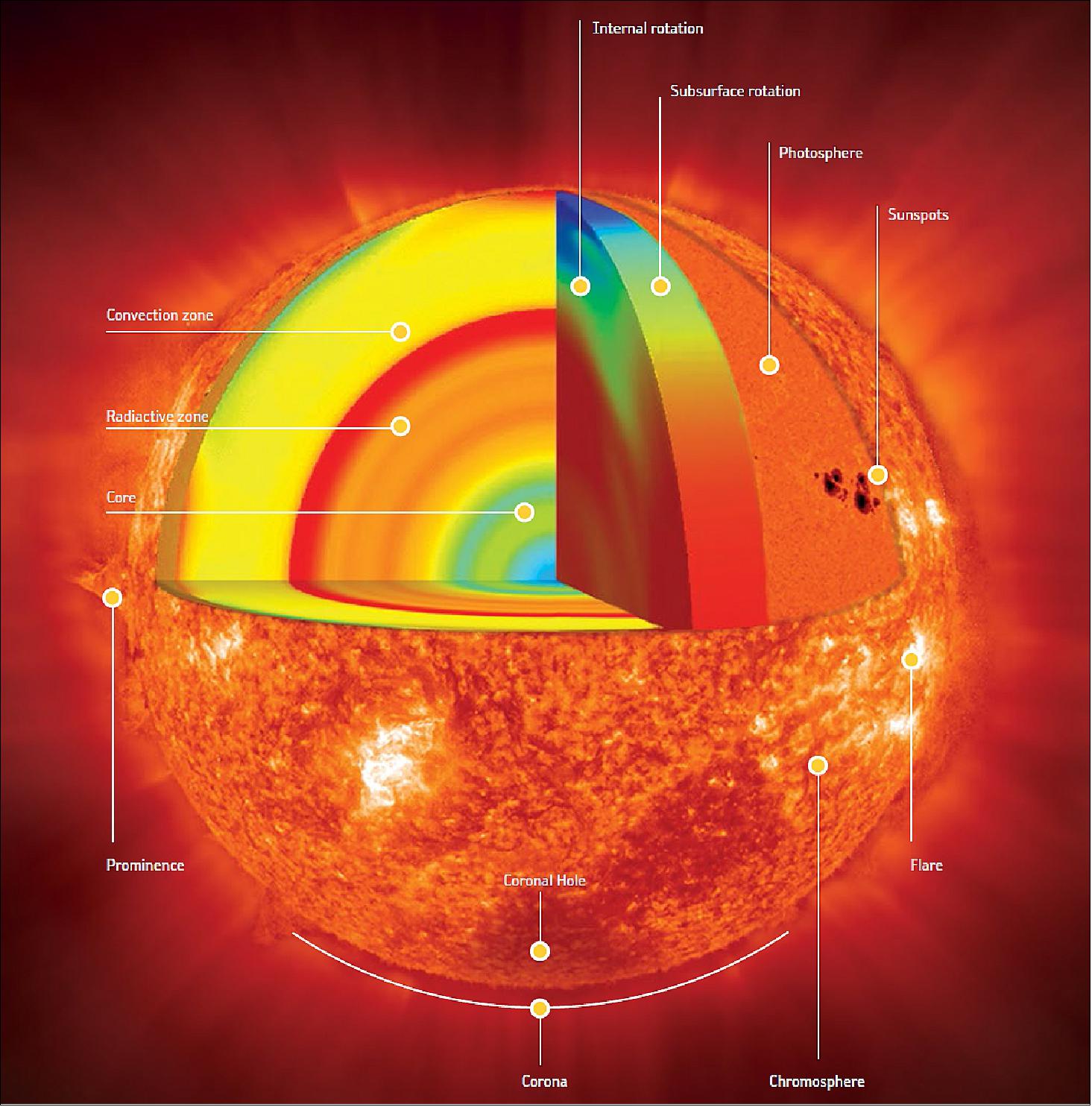
Figure 35: The anatomy of our Sun (image credit: ESA, NASA, Ref. 39)
Legend to Figure 35: Left cutaway: The Sun’s interior explored with sound waves. Red depicts layers where sound travels faster than predicted by theory, implying that the temperature is higher than expected, while blue indicates slower speeds and lower temperatures. The prominent red layer marks the transition between the turbulent outer convection zone and the more stable inner radiative zone.
Right cutaway: The Sun’s internal rotation, where red depicts fast rotation and blue slower rotation. Outer layers: Visible light images show sunspots, cool dark features in the photosphere, which lies below the chromosphere. Flares, resulting from the release of a buildup of magnetic energy, and coronal mass ejections (CMEs, giant clouds of electrically charged atomic particles launched into space) often occur in magnetically active regions around sunspot groups.
- SOHO also shed light on the ‘solar neutrino problem’ – a major discrepancy between the rate at which neutrinos were predicted to be created by nuclear fusion in the deep solar interior and the rate measured at Earth. SOHO confirmed that the standard model of the Sun is correct, ruling out that possible explanation. Instead, the discrepancy had to be explained by the physics of the neutrino, as confirmed by better neutrino measurements a few years later.
- The solar heating mystery: Why the Sun’s corona is heated to the extremely high 1–2 million °C when the visible surface (the photosphere) is ‘only’ about 5500°C has long been a mystery of solar physics. SOHO has revealed an extremely dynamic atmosphere where plasma flows and small-scale transient events play an important role. They also discovered new dynamic phenomena such as solar tornadoes and global coronal waves – disturbances associated with coronal mass ejections that can travel around the entire solar globe – and provided evidence for the upwards transfer of magnetic energy from the surface to the corona through a magnetic carpet – a weave of magnetic loops extending above the Sun’s surface.

Figure 36: Composite image combining EIT (Extreme-ultraviolet Imaging Telescope) images from three wavelengths to reveal solar features unique to each wavelength. Blue areas indicate ‘cooler’ regions at 1 million ºC, green ‘warmer’ regions at 1.5 million ºC, and red ‘hot’ regions at 2 million ºC (image credit: ESA, NASA)
- A near-loss and a dramatic recovery: The mission almost ended on 25 June 1998 when control was lost during a routine maneuver. It took three months to restore operations in one of the most dramatic recovery operations in space history, including 2.5 weeks to thaw frozen hydrazine propellant in the tank and pipes. Unexpectedly, all 12 instruments survived despite the extreme temperatures they suffered during the time contact was lost. — But the drama was not over yet: all three gyroscopes later failed, the last in December 1998. New control software that does not rely on gyros was developed and installed in February 1999, allowing the spacecraft to return to full scientific operations. This made SOHO the first spacecraft to be stabilized in three axes without gyros.

Figure 37: Members of the SOHO recovery team at NASA Goddard Space Flight Center in September 1998, including staff from ESA, NASA, Matra Marconi Space (now Airbus DS), Allied Signal Technical Services Co., Computer Sciences Corp. and Space Applications Corp (image credit NASA/ESA, Ref. 39)
- SOHO was also on hand to document the 2003 Halloween flares, the demise of comet ISON on U.S. Thanksgiving Day 2013 (see Figure 45), and the deep and strangely profound solar minimum that marked the transition from solar cycle No 23 to No 24.
- “SOHO has been truly extraordinary and revolutionary in countless ways,” says astrophysicist Karl Battams at NRL (Naval Research Laboratory) in Washington D.C. “SOHO has completely changed our way of thinking about the Sun, solar active regions, eruptive events, and so much more. I honestly can’t think of a more broadly influential space mission than SOHO.”
- NASA/ESA has extended SOHO’s current mission out to the end of 2016. With any luck, SOHO will complete solar cycle 24, and take us into cycle 25 to boot.
- Current (2015) missions such as SDO (Solar Dynamics Observatory), Hinode, STEREO, and PROBA-2 have joined SOHO in watching the Sun around the clock. The solar occulting disk capabilities of SOHO’s LASCO C2 and C3 camera remains unique, though ESA’s PROBA-3 mission launching in 2018 will feature a free-flying solar occulting disk.
- SOHO has observed more than 20, 000 coronal mass ejections to date, pinpointing their sources on the Earth-facing hemisphere of the Sun, and determining their speed and direction to provide up to three days’ warning – sufficient to take action on Earth. “SOHO is recognized as a unique resource that is critically important for space weather research and improved space weather predictions,” says Bernhard Fleck, ESA’s SOHO project scientist and mission manager. - In addition to investigating how the Sun works, SOHO is the most prolific discoverer of comets in astronomical history, with the destinies of more than 3000 tracked as these icy worlds endured fiery encounters with the Sun.
- A prime goal was to observe where the solar wind – electrically charged atomic particles streaming from the Sun – is produced and how it is accelerated to beyond 3 million km/h. Scientists have made great strides in answering this fundamental question. They measured the acceleration profiles of both the ‘slow’ and ‘fast’ solar wind and found that the fast solar wind streams into interplanetary space by ‘surfing’ on waves produced by vibrating magnetic field lines. Mapping the outflow of the plasma from coronal holes – darker, cooler and less dense areas of the Sun’s corona where the Sun’s magnetic field reaches into space, allowing hot gas to escape – revealed a clear connection between the flow speed and the structure of the chromosphere.
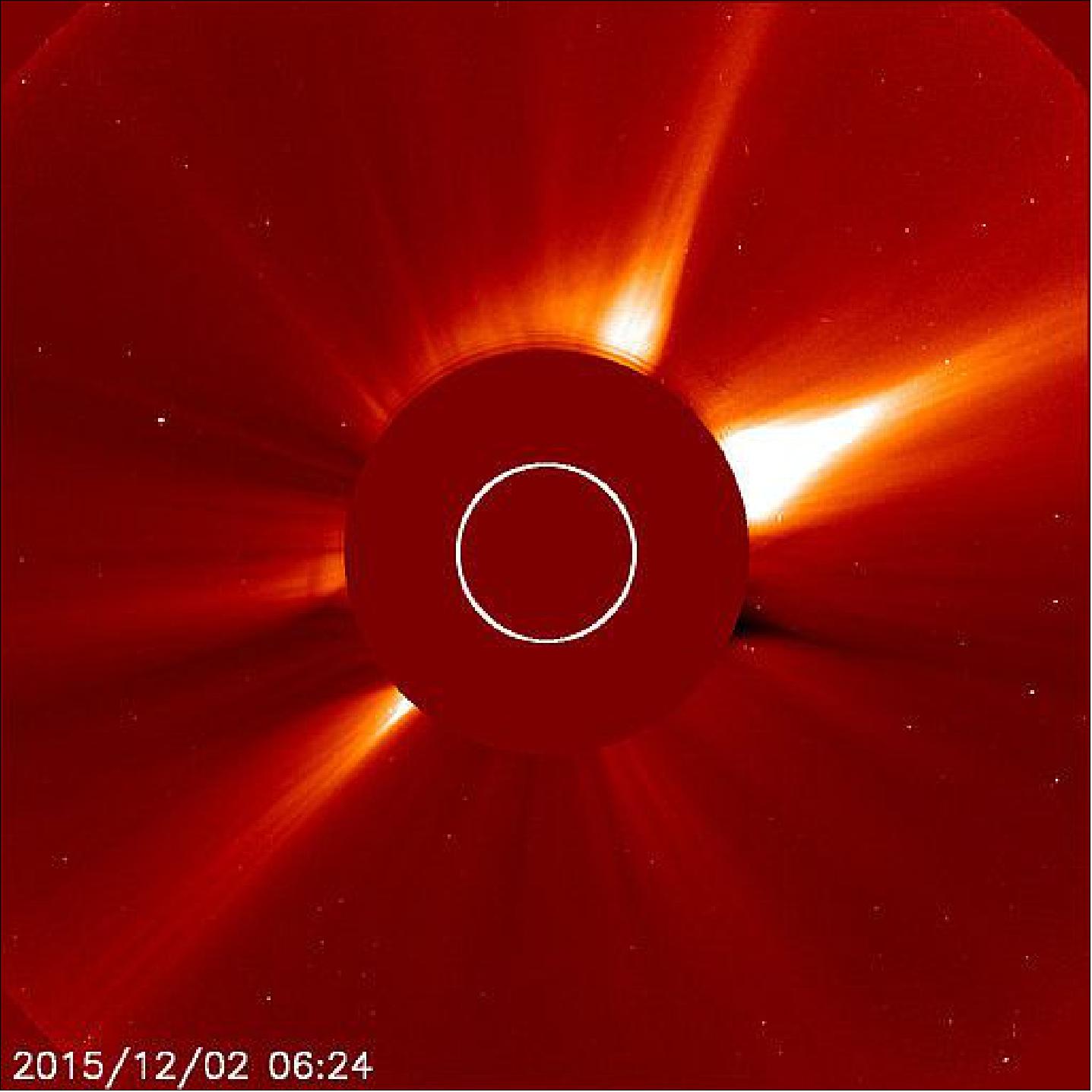
Figure 38: The Sun as seen through SOHO’s LASCO C2 telescope at 06:24 GMT on Dec. 2, 2015, on the 20th anniversary of the satellite’s launch (image credit: ESA, NASA)
Legend to Figure 38: The small white circle in the center of the image indicates the position and size of the Sun behind the telescope's occulting 'coronagraph mask', seen as the wider disc. The mask blocks out the dazzling light that otherwise drowns out this region, allowing details of the corona – the Sun's dynamic outer atmosphere – to be seen.
• October 25, 2015: For an astronomer, discovering a comet can be the highlight of a lifetime. Great comets carry the names of their discoverers into history. Comet Halley, Comet Lovejoy, Comet Hale-Bopp are just a few examples . — Imagine the frustration, though, if every time you discovered a comet, it was rapidly destroyed. Believe it or not, this is what happens almost every day to the most prolific comet hunter of all time. 40)
- SOHO, the joint ESA/NASA mission, is orbiting the sun in a Halo orbit at L1 (Lagrangian Point 1), about 1.5 million km from Earth. In September, SOHO discovered its 3000th comet—more than any other spacecraft or astronomer. And, just about all of SOHO’s comets have been destroyed. "They just disintegrate every time we observe one," said Karl Battams, a solar scientist at NRL (Naval Research Laboratory) in Washington, D.C. “SOHO sees comets that pass very close to the sun — and they just can’t stand the intense sunlight.”
- The overwhelming majority of SOHO's comet discoveries belong to the Kreutz family. Kreutz sungrazers are fragments from the breakup of a single giant comet thousands of years ago. They get their name from 19th century German astronomer Heinrich Kreutz, who studied them in detail. On average, a new member of the Kreutz family is discovered every three days. Unfortunately for these small comets, their orbits swoop perilously close to the sun. "There's only one Kreutz comet that made it around the sun – Comet Lovejoy. And we are pretty confident it fell apart a couple of weeks afterwards," says Battams.
- Although SOHO’s comets are rapidly destroyed, they nevertheless have great scientific value. For instance, the comets’ tails are buffeted and guided by the sun’s magnetic fields. Watching how the tails bend and swing can tell researchers a great deal about the sun’s magnetic field.
- Prior to the launch of SOHO in 1995, only a dozen or so comets had ever even been discovered from space, while some 900 had been discovered from the ground since 1761. SOHO has turned the tables on these figures, making itself the greatest comet hunter of all time. — But SOHO hasn’t reached this lofty perch alone. The spacecraft relies on people who sift through its data. Anyone can help because SOHO’s images are freely available online in realtime. Many volunteer amateur astronomers scan the data on a daily basis for signs of a new comet. The result: 95% of SOHO comets have been found by citizen scientists. Whenever someone spots a comet, they report it to Battams. He goes over the imagery to confirm the sighting and then submits it to the Central Bureau for Astronomical Telegrams, which gives it an official name.
• Sept. 15, 2015: The ESA/NASA SOHO mission detected its 3000th comet on 14 September 2015, seen as the dot in the cross-hairs in the inset of Figure 39 heading towards the Sun (left). It was spotted by Worachate Boonplod of Samut Songkhram, Thailand. 41) 42)
- SOHO's mission is to observe the sun and interplanetary space, above Earth's atmosphere that blocks so much of the sun's radiation. From there, SOHO watches the solar disk itself and its surrounding environment, tracking the constant outward flow of particles known as the solar wind, as well as giant explosions of escaping gas called coronal mass ejections, or CMEs. In its two decades in orbit, SOHO has opened up a new era of solar observations, dramatically extending our understanding of the star we live with.
- The telescope’s comet prowess, however, was unplanned and has turned out to be an unexpected benefit. With its clear view of the sun's surroundings, SOHO can easily spot a special kind of comet called a sungrazer, because of its close approach to the sun.
- "SOHO has a view of about 12-and-a-half million miles beyond the sun," said Joe Gurman, mission scientist for SOHO at NASA's Goddard Space Flight Center in Greenbelt, Maryland. "So we expected it might from time to time see a bright comet near the sun. But nobody dreamed we'd approach 200 a year."
- SOHO's great success as a comet finder is dependent on the people who sift through its data – a task open to the world as the data is publicly available online in near-real time. A cadre of volunteer amateur astronomers dedicate themselves to searching the data via the NASA-funded Sungrazer Project. While scientists often search SOHO imagery for very specific events, various members of the astronomy community choose to comb through all the imagery in fine detail. The result: 95 percent of SOHO comets have been found by these citizen scientists.
- "The people who have found comets represent a very broad cross section as the program is open to anyone who has interest," said Karl Battams, a solar scientist at the Naval Research Laboratory in Washington, D.C. Battams has been charged with running the SOHO comet-sighting website since 2003. "There are scientists, teachers, writers. We have even had two 13-year-olds."
- More than just a celebrated bright vision in the night sky, comets can tell scientists a great deal about the place and time where they originated. Comets are essentially a clump of frozen gases mixed with dust. They are often pristine relics that can hold clues about the very formation of our solar system. On the other hand, if they have made previous trips around the sun, they can hold information about the distant reaches of the solar system through which they've traveled.
- Watching these sungrazing comets also help us learn about our sun. Their tails of ionized gas illuminate magnetic fields around the sun, so they can act as a tracer that helps scientists observe these invisible fields. Such fields have even ripped off comet tails allowing astronomers to watch the lost tails blowing in the steady outpouring of solar particles streaming off our closest star. The tails act as giant windsocks in this solar wind, showing researchers the details of the wind's movement.
- At almost 20 years old, the SOHO mission is a respected elder in NASA's Heliophysics System Observatory – the fleet of spacecraft that both watches the sun and measure its effects near Earth and throughout the solar system. SOHO is a cooperative effort between ESA and NASA. Mission control is based at NASA Goddard. SOHO’s LASCO (Large Angle and Spectrometric Coronagraph Experiment), which is the instrument that provides most of the comet imagery, was built by an international consortium, led the Naval Research Lab in Washington, D.C.

Figure 39: Illustration of the 3000th comet, acquired by the LASCO instrument on SOHO and spotted on Sept. 14, 2015 by Worachate Boonplod of Samut Songkhram, Thailand (ESA/NASA/SOHO)
• July 13, 2015: SOHO has been watching the Sun for almost 20 years. In that time it has seen solar activity ramp up and die down repeatedly. Its EIT (Extreme ultraviolet Imaging Telescope) has taken images of the resulting waxing and waning of the Sun’s corona – its atmosphere – that are impossible to record from the ground. 43)
- Our atmosphere blocks more than 75% of ultraviolet radiation from reaching Earth’s surface, and extreme ultraviolet is entirely absorbed. To see it, spacecraft such as SOHO must be used.
- In this composition of Figure 40, brighter images show times when there was more activity on the Sun. This activity is driven by the Sun’s magnetic field and follows a cycle of about 11 years. The brightest images occur around the time of solar maximum. At these times, the magnetic field of the Sun is highly dynamic, changing its configuration and releasing energy, partly in the form of ultraviolet radiation into space.
- The high temperature of the corona is a puzzle to solar physicists since the bright ‘surface’ of the Sun is only about 5700ºC and therefore is incapable of directly heating the corona to over a million degrees. Although scientists think that magnetic activity may be responsible, the exact mechanism is still under investigation.
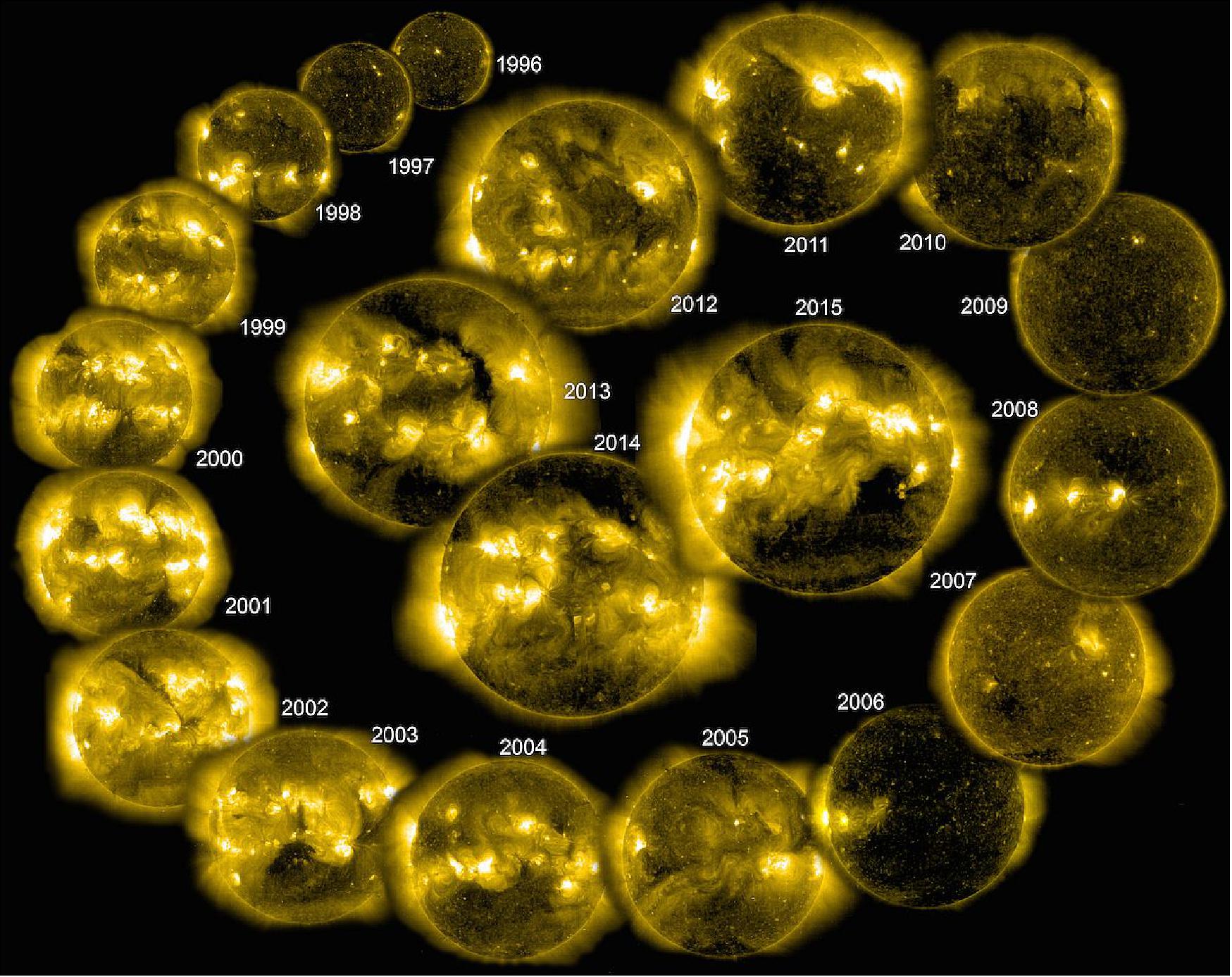
Figure 40: Twenty SOHO views of the Sun. The individual images in this composition show gas with a temperature of about 2 million degrees Celsius in the Sun’s atmosphere. This atmosphere, known as the corona after the Latin word for crown, extends millions of kilometers from the Sun. The corona carries away the particles of the solar wind. If accelerated streams of particles reach Earth and strike our atmosphere, they can spark colorful displays of the aurora. Each of the images shown here was taken in the springtime (image credit: SOHO, ESA&NASA)
• March 19, 2015: This week's spectacular glowing auroras in the night sky further south than usual highlighted the effect that 'space weather' can have on Earth. A strong solar flare was detected on the Sun on March 15 , an event generally associated with strong mass ejections and solar radiation storms. The stream of particles pouring out from our nearest star - the solar wind - was detected by satellite to be speeding up. 44)
- Ground-based sensors recorded a magnetic impulse at the moment the shockwave from the Sun swept past our planet. That triggered a geomagnetic storm, generating a spectacular Aurora seen by thousands down to the southernmost parts of Scandinavia and in Wales (Figure 42).
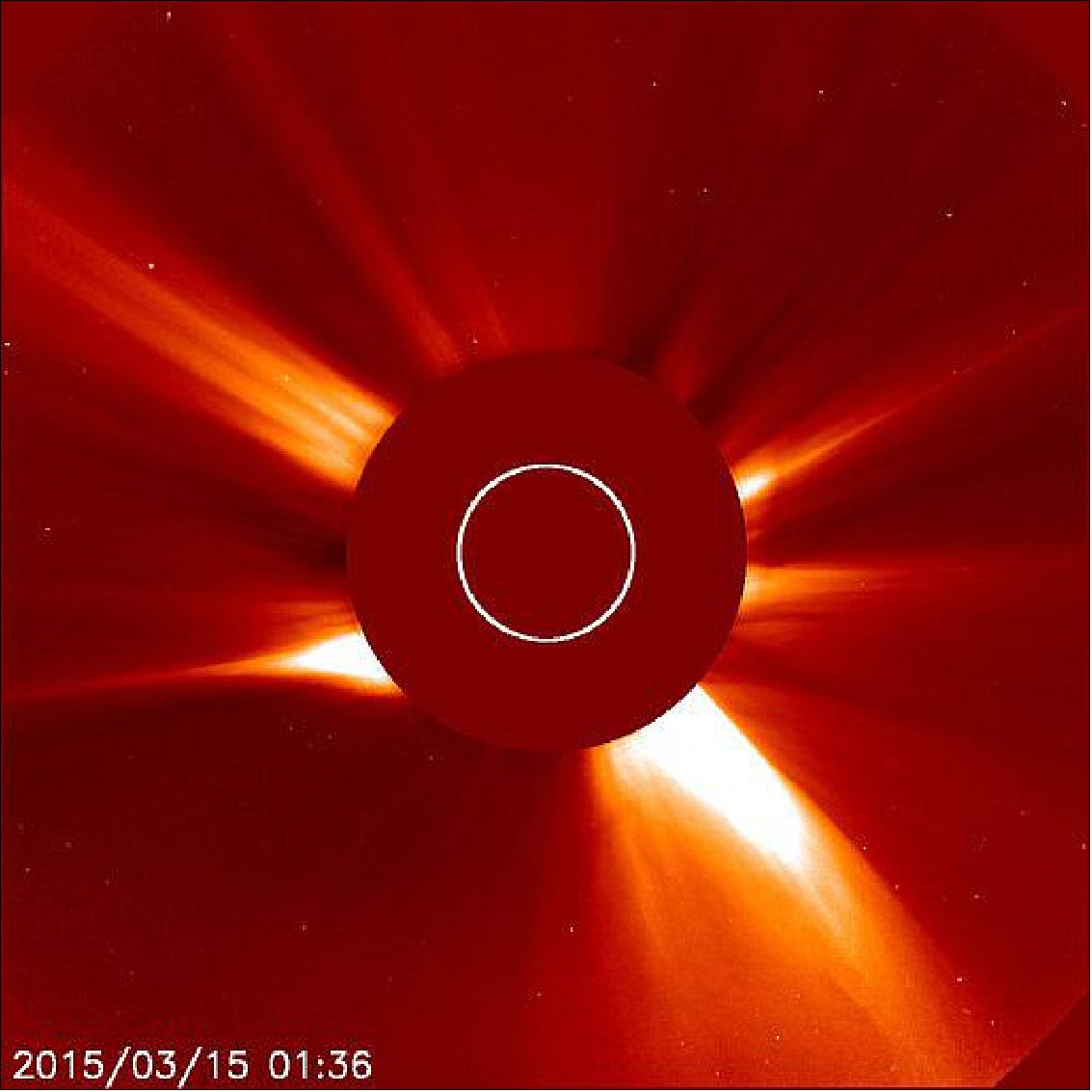
Figure 41: Coronal mass ejection viewed by SOHO LASCO C2 instrument on March 15, 2015 (image credit: ESA, NASA)

Figure 42: Aurora Borealis on 17 March 2015 (image credit: Crey – CC BY 2.0)
• SOHO Comet 2875, Feb. 20, 2015: A newly-discovered comet, skirting the Sun, was spotted in imagery taken by the LASCO wide-field coronograph of SOHO. It's a 'non-group comet,' meaning that it does not appear to be related to any other comet or comet family that we have on record. Most comets that SOHO sees belong to the Kreutz family. Kreutz sungrazers are fragments from the breakup of a single giant comet many centuries ago. It first appears as a faint dot in the upper right, then eventually swoops just under the Sun. Towards the end of the video, as the comet begins to sport a tail, the Sun bursts out with a coronal mass ejection to add something more to the scene. 45) 46)
- This was SOHO's comet discovery number 2,875. Observers (mostly amateurs) using SOHO data discover over a hundred of these a year. SOHO sees these non-group comets a few times a year.
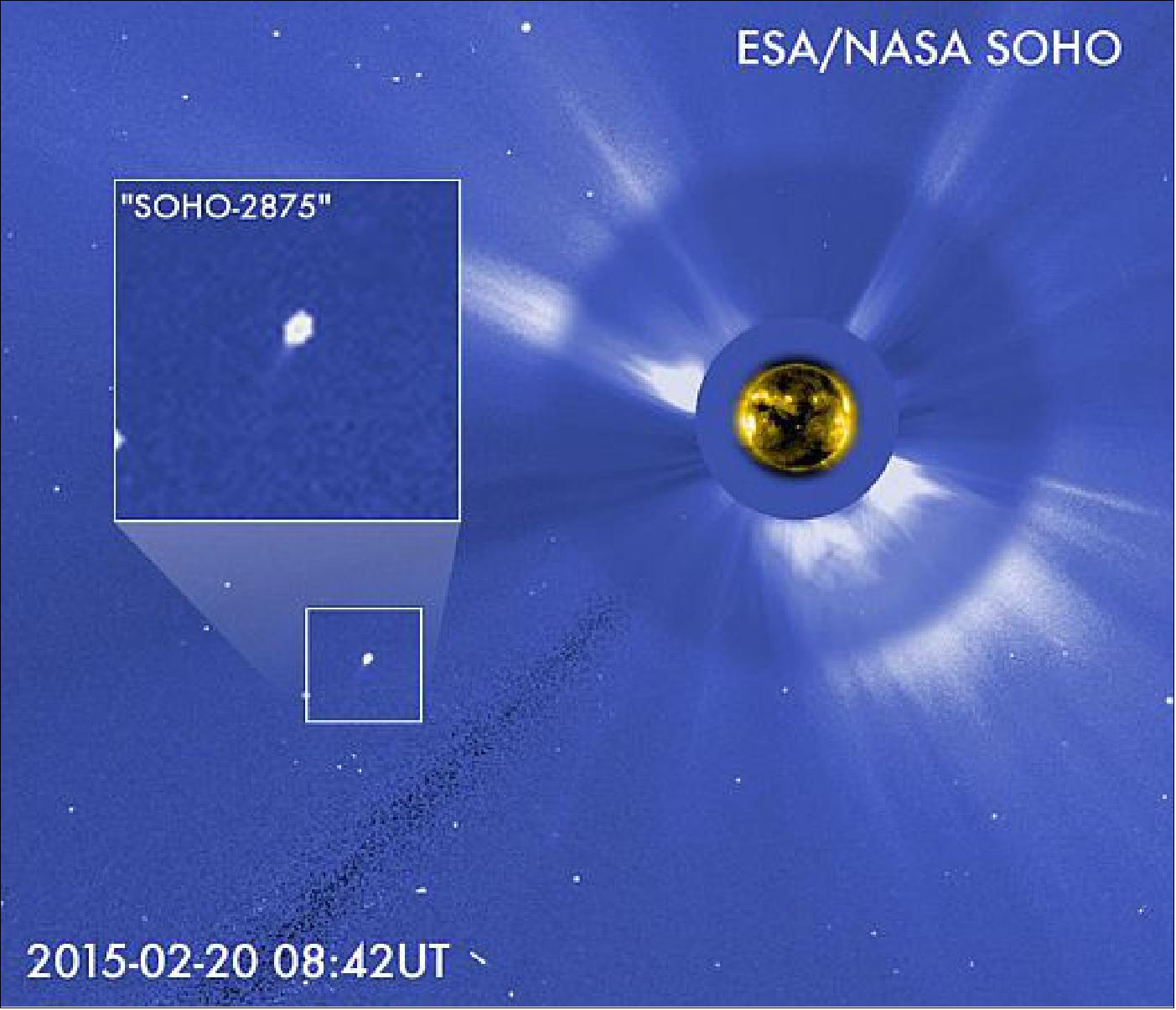
Figure 43: Comet SOHO-2875 survived its close passage of the Sun and may make an appearance in the evening sky soon (image credit: NASA, ESA)
• December 01, 2014: While comet ISON was barely visible for Earth observers, the SOHO spacecraft had a ring-side seat to watch its disintegration. The image of Figure 44 is a montage spanning three days from 28–30 November 2013. Comet ISON enters the image at the lower right, passes round the Sun and exits the frame towards the upper right. The bright star to the lower left is the red supergiant star Antares. 47)
- Astronomers had been tracking the comet for more than a year as it edged closer to the Sun, and by late November it had passed into the field of view of SOHO’s LASCO C3 camera. It was to skim the Sun, just 1,165,000 km above the fiery surface. This is approximately 50 times closer to the Sun than innermost planet Mercury, and the comet was officially termed a ‘sungrazer’. If it survived the encounter it was expected to become extremely bright and be a well-placed object, visible to the naked eye in Earth’s night sky.
- Calculations based on its orbit show that ISON began its journey towards the Sun about 3 million years ago, dislodged from its distant orbit by a passing star. Now, its fate would be sealed within days.

Figure 44: LASCO C3 camera image of Comet ISON disintegrating in its orbit around the Sun (image credit: ESA, NASA)
- On 27 November, the comet brightened dramatically by a factor of about ten. Yet just before it reached closest approach to the Sun, it began to fade. This was a strong indicator that the heart of the comet, the icy nucleus, had broken up. Many expected it would disperse completely but, at first, it looked as if they were wrong.
- Comet ISON appeared to survive the close approach, emerging on the other side of the Sun. Some still hoped for a bright display in the night skies. But they were to be disappointed. Quickly, the comet began to disappear. A recent analysis of SOHO data showed that the nucleus had indeed disintegrated just before closest approach to the Sun. Nothing appreciable was left of it, just a lot of dust and vapor.
• Nov. 20, 2014: ESA's SPC (Science Program Committee) has given green light for the flotilla of spacecraft to continue their key scientific endeavors for at least another two years. After a comprehensive review by the Science Program’s advisory structure of the current operational status and likely scientific return of each mission in the future, the SPC agreed to continue funding for six ESA-led missions (Cluster, INTEGRAL, Mars Express, PROBA-2, SOHO and XMM-Newton) for the period 1 January 2015 – 31 December 2016. 48)
• Sept. 15, 2014: A retrospective view is presented of the giant solar flare, the most powerful one on record during the space age so far, which occurred on November 4, 2003 and is featured as 'space science image of the week.' 49)
- The SOHO spacecraft of ESA/NASA monitored the extraordinary solar event in November of 2003, showing a blistering solar flare bursting from active region 10486 at 19:29 GMT. In this process, the energy of up to a thousand billion Hiroshima-sized atomic bombs can be released in just a few minutes. That release is seen in Figure 45. The horizontal white streak is where the UVCS (UV Coronagraph and Spectrometer)camera has been blinded by the brightness of the flare. Things began when a giant sunspot, fully ten times the diameter of Earth, hove into view around the western limb of the Sun in late October. It was followed by another, equally large, spot and together they moved across the face of the Sun generating flares on an almost daily basis. This image shows the second spot’s parting volley. Analysis showed that it clocked in at X28 (estimated), making it 28 times more powerful than an X1. A billion tons or so of the solar atmosphere was propelled into space at a speed of 2300 km/s – a staggering 8.2 million km/h.
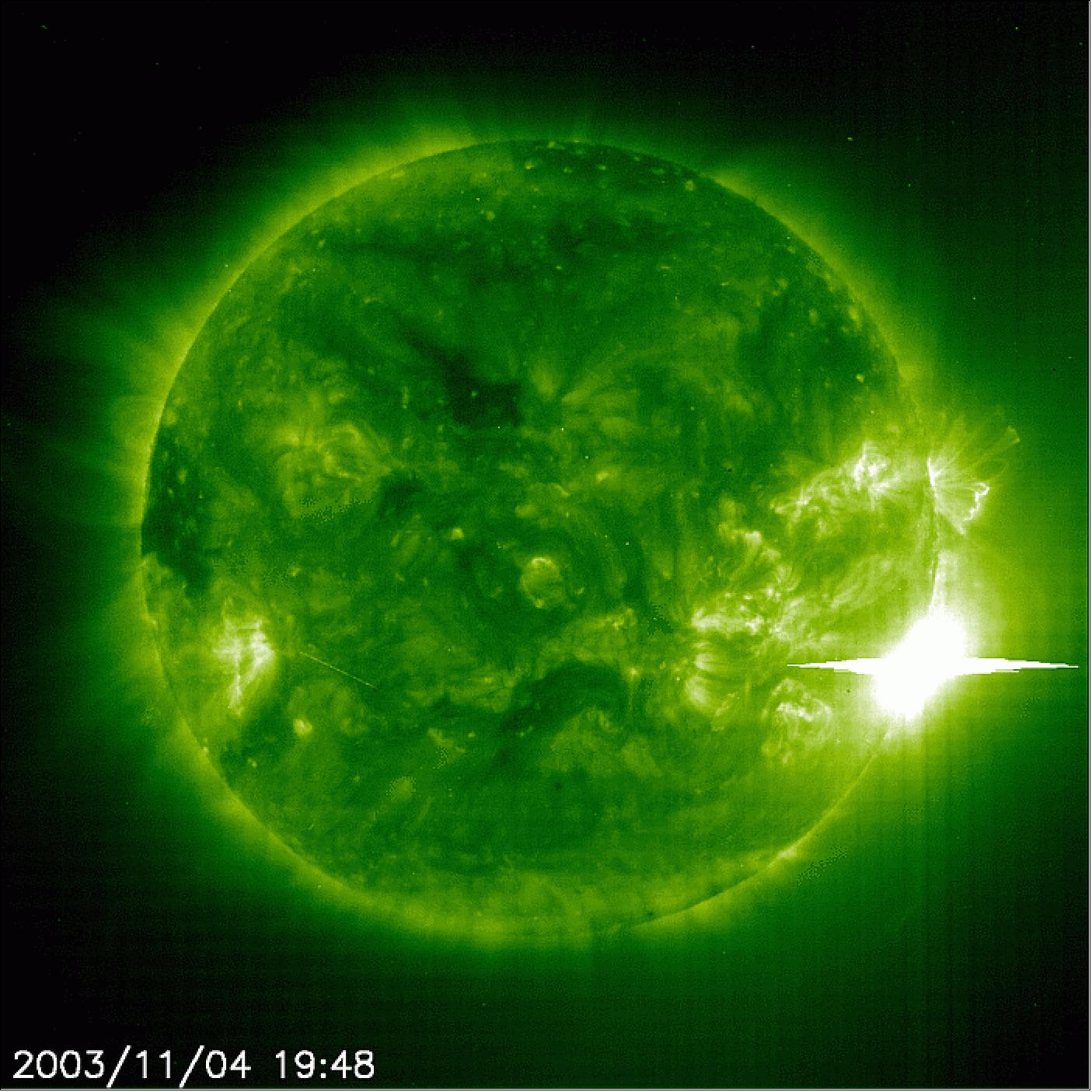
Figure 45: The SOHO spacecraft captured the climax of the giant Halloween solar flare with the UVCS on Nov. 4, 2003 (image credit: ESA, NASA)
- The space weather from these enormous solar storms slammed into Earth's magnetic field from October 19 through November 5. The effects of these storms were so devastating that aircraft controllers had to re-route aircraft, it affected numerous satellite systems and communications, and it also caused a power outage in Sweden for about an hour. - During the height of the solar activity, more than half of the deep space and near-Earth space science missions experienced the effects of the Halloween storms of 2003. The SOHO spacecraft failed temporarily. NASA's ACE (Advanced Composition Explorer) satellite experienced damage, and instruments aboard many spacecraft had to be shut down temporarily. JAXA experienced the loss of the ADEOS-2 spacecraft, which was launched on Dec. 14, 2002.
- The GOES XRS instrument was saturated at the X17 level for 12 minutes during this flare. Using historical flare data and mathematical modeling the peak flux was estimated to be X28. This flare is likely the most intense flare observed since 1976 when GOES X-ray measurements of solar flares began, although there are other flares in the record that also saturated the sensor. 50)
• In July 2014, a study was published by an international research team which analyzed a solar CME event, observed in January 2005, and its impact on space weather. The study is based on measurements from twelve spacecraft operating in the Earth's magnetosphere: ESA's four Cluster spacecraft, one of the Chinese-ESA Double Star spacecraft (TC-1), the ACE (Advanced Composition Explorer), the IMAGE (Imager for Magnetopause-to-Aurora Global Exploration) satellite, and five geosynchronous satellites from Los Alamos National Laboratory. An additional nine low-altitude satellites provided measurements of the effects in the ionosphere and atmosphere. 51)
On January 20, 2005, the ESA/NASA SOHO spacecraft detected an explosion on the Sun which ejected a huge cloud of plasma (electrically charged gas) into space. Subsequent observations indicated that this was one of the fastest coronal mass ejections (CMEs) during solar cycle 23, with a velocity that peaked at perhaps 3000 km/s at a distance of between 3 and 50 solar radii, before slowing to 1000 km/s as it approached Earth. The typical speed of particles in the solar wind is 400-700 km/s. 52)
- As a result, the CME took only 34 hours to cross the 150 million km gulf between the Sun and Earth, compared with the typical time of 3-4 days.
- By the time it reached Earth's vicinity, the CME exhibited two extremely unusual features. First, it was observed to contain a large amount of solar filament material. Filaments are ribbons of dense plasma that are driven upward into the Sun's outer atmosphere – the corona – by strong magnetic fields emerging from active regions. However, despite observations indicating that more than 70% of active region eruptions involve solar filaments, these structures are rarely identifiable in CMEs arriving at Earth.
- Second, the filament material was displaced from its expected position in the CME. As the coronal mass ejection decelerated en route to Earth, the momentum of the massive solar filament caused it to push its way toward the front, eventually making contact with the sheath region.
Case studies such as this- which combine all available satellite observations in regions from Sun to Earth with linked global models – allow the research team to trace chains of processes and their interactions, in order to identify the drivers of the extreme features and track the changes that appear throughout the system as a consequence.
Predicting the impact of the Sun's activity on Earth is one of the major goals of space weather research. Hence, coordinated observations and modelling of unusual events by multiple spacecraft orbiting near Earth are essential to improve our understanding of the Sun-Earth connection.
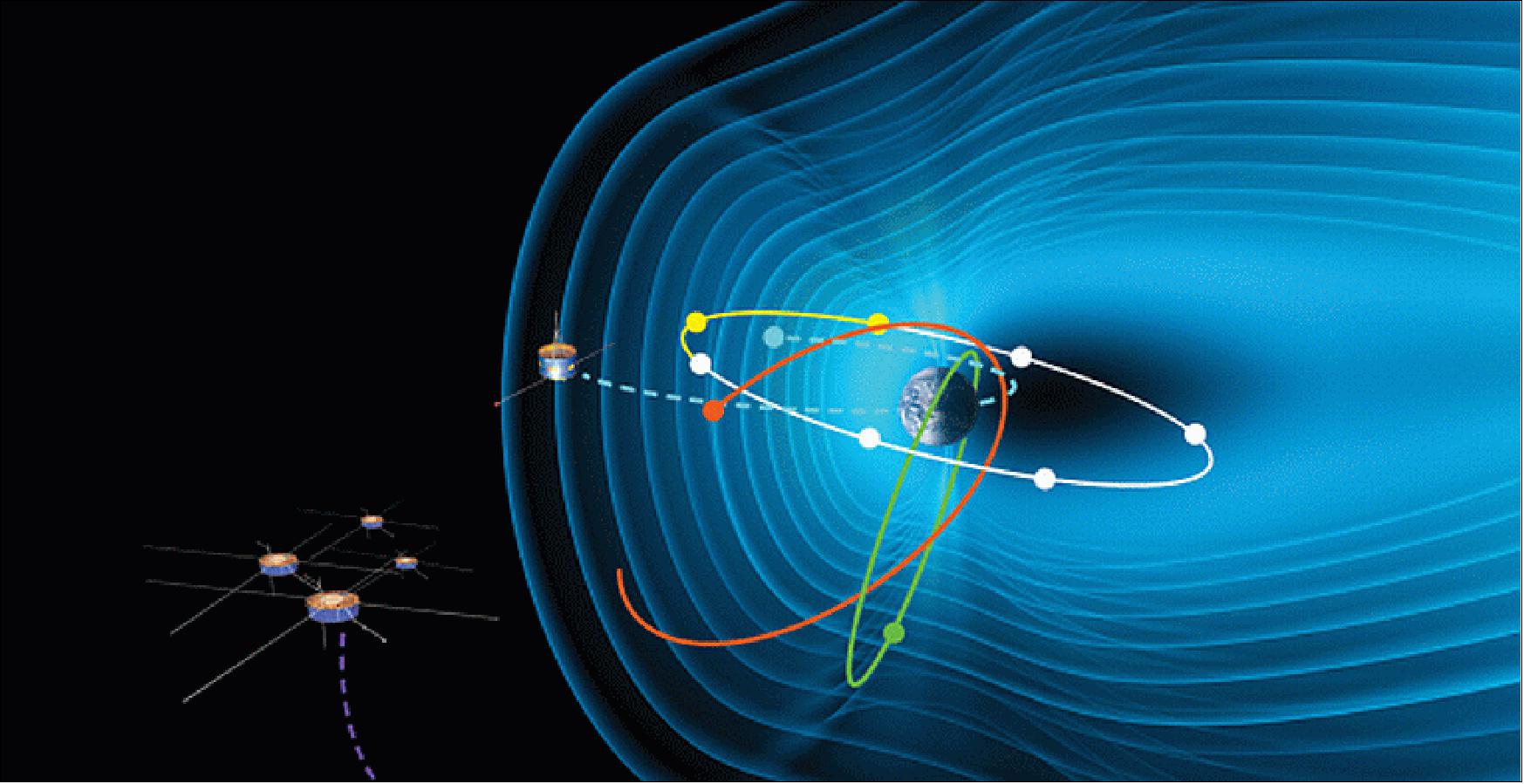
Figure 46: Orbits of 12 spacecraft in Earth's magnetosphere, January 2005 (image credit: ESA/ATG medialab)
• June 16, 2014: Back in 1998, the Sun was behaving as expected. The approximately 11-year cycle of activity was proceeding smoothly, heading towards a peak in 2001. The SOHO spacecraft captured this image of Figure 47 on November 47, 1998 through its ultraviolet telescope, showing radiation from iron atoms bathed in a gas of around a million degrees Celsius. 53)
- This textbook image of solar activity shows two brighter bands circling the Sun at the same latitude in each hemisphere. At visible wavelengths these bright loops and patches are associated with dark smudges known as sunspots. They are produced when loops of magnetism become buoyant and rise from inside the Sun into the atmosphere.
- When the cycle begins, the active regions appear at high latitudes in sparse numbers, disappearing after a few weeks or so. As the cycle proceeds, new and often larger active regions appear more frequently at successively lower latitudes. Many can be larger than Earth in size, and they sometimes persist for months.
- This activity takes place in both hemispheres simultaneously, and about five or six years into the cycle sunspots reach lower latitudes closer to the equator. This is known as solar maximum.
- After this, the number of spots begins to decline until they virtually disappear and the cycle starts again at high latitudes. It is one of the enduring mysteries of the Sun why this cycle happens. Certainly, it is linked to the way the Sun generates magnetism deep inside its gaseous layers but the details remain elusive.
- In recent years, the Sun has deviated from this textbook behavior. The current cycle was about two years late in starting, the hemispheres are behaving differently and the peak of activity is relatively modest. The next cycle is expected to continue in this new vein. It may even be weaker than the current cycle.
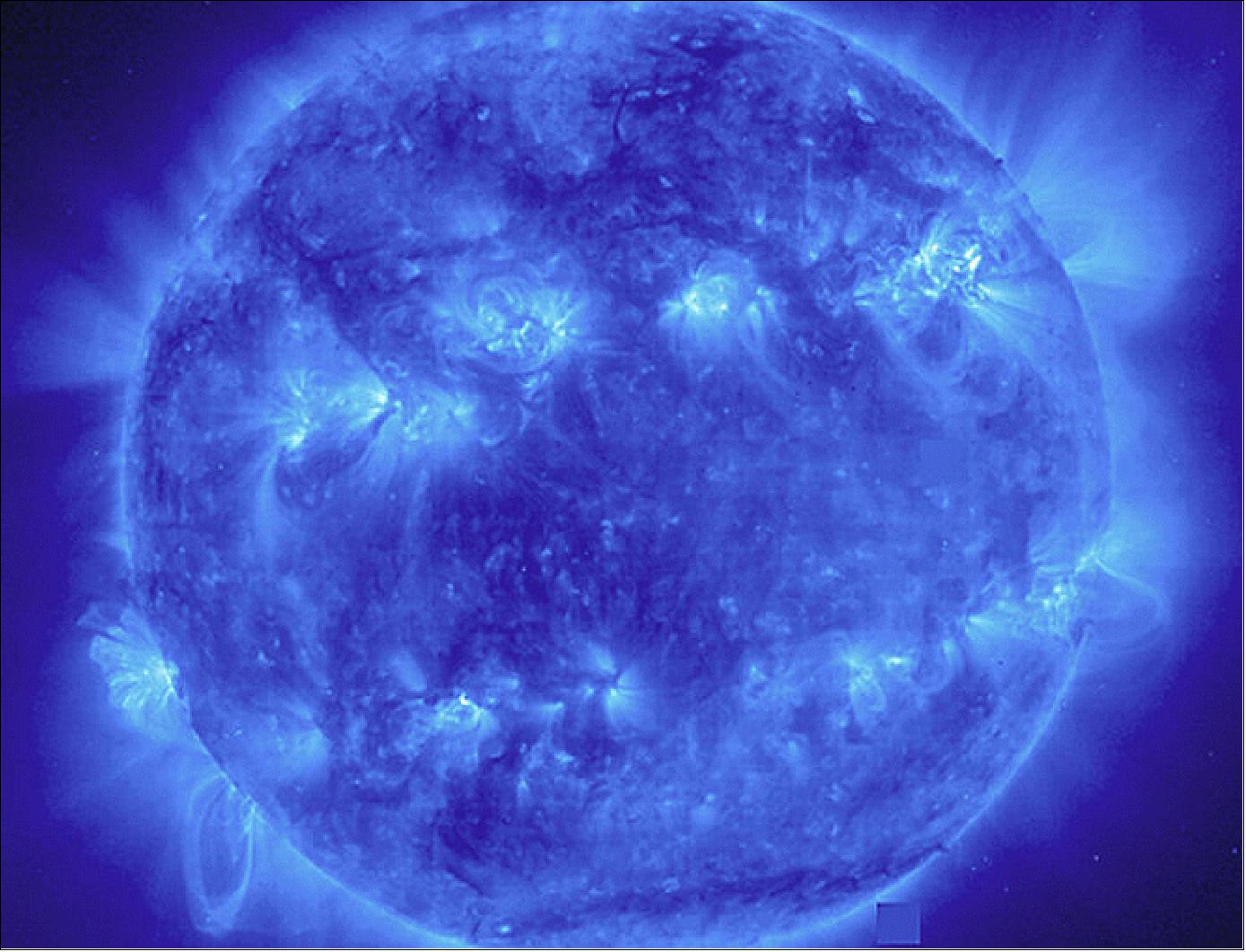
Figure 47: Necklaces of solar activity as observed by SOHO on Nov. 9, 1998 (image credit: ESA, NASA)
• The SOHO mission is operational as of January 2014. - On Dec. 02, 2013, SOHO completed 18 years on-orbit. On 19 June 2013, the ESA Science Program Committee approved a new extension, until 31 December 2016, for SOHO, subjected to mid-term confirmation, in late 2014. 54)
• On Jan. 14, 2014, a CME (Coronal Mass Ejection) is seen, erupting away from the sun in this image from SOHO (Figure 48). The sun is obscured to make the dimmer solar atmosphere more visible. The bright image in the top right is Venus (image credit: ESA,NASA). 55)
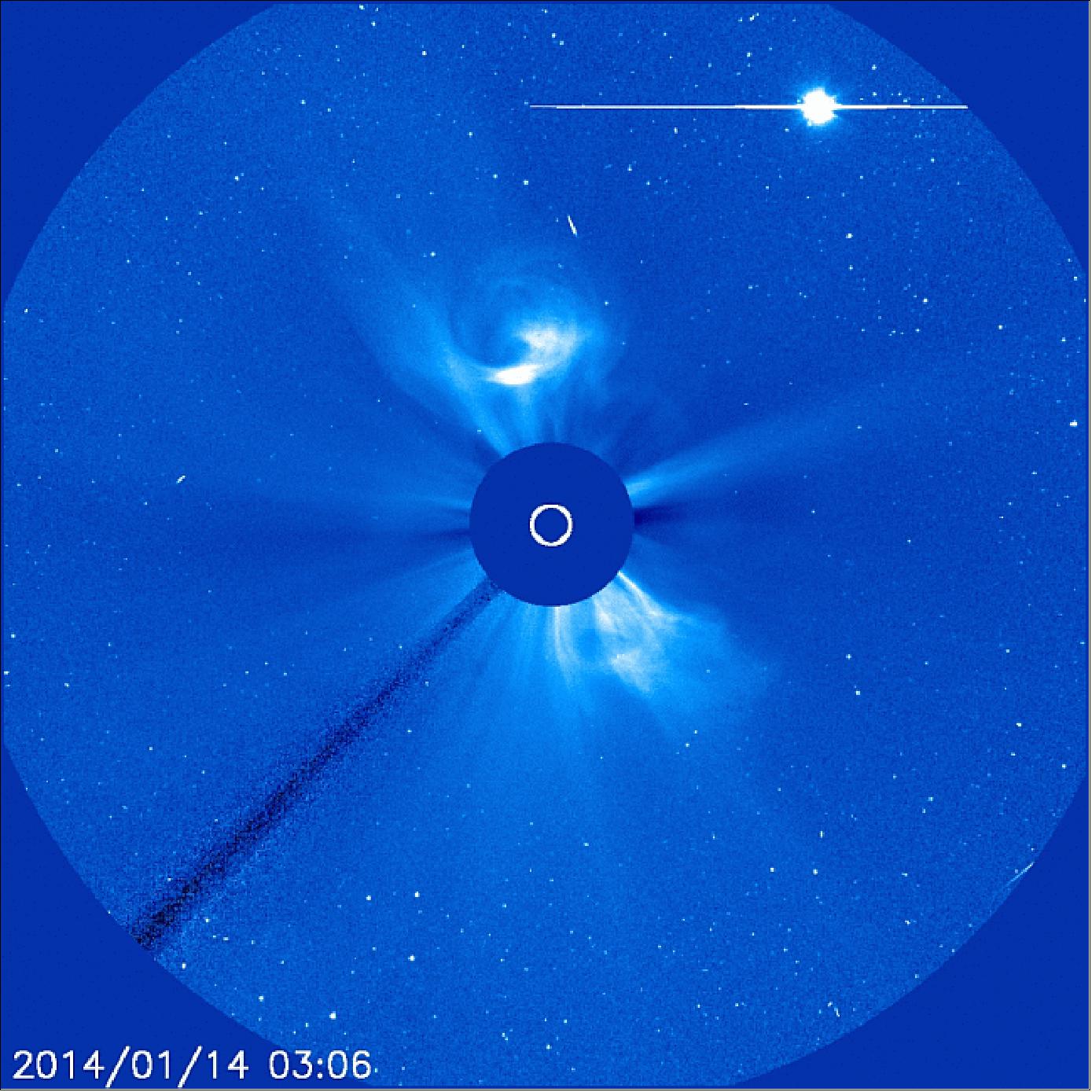
Figure 48: SOHO captures a CME on January 14, 2014 (image credit: NASA, ESA)
• On January 13, 2014, ESA released a SOHO image entitled 'The abstract science of the dynamic Sun,' (Figure 49). The placid appearance of the Sun's surface belies a hot fireball of plasma in constant turmoil. A granular network invisible to the naked eye pervades the solar disc, with cells of hotter and colder plasma popping up, merging and disappearing within only a few hours. 56)
The boundaries between these constantly moving cells are hectic places. Powerful jets of plasma are often launched along the separation lines whenever the cell pattern changes, which may happen as a result of variations in the configuration of the magnetic field – known as ‘magnetic reconnection’.
To learn more about these reconnection jets and the energetic events that cause them, scientists observe the Sun at different wavelengths using a variety of techniques.
This image, which could be mistaken for a piece of abstract art, shows a series of observations performed with the ESA/NASA SOHO (Solar and Heliospheric Observatory) mission to study the evolution of reconnection jets on a small patch of the Sun’s surface.
The image shows 60 frames taken with the ultraviolet spectrometer SUMER on SOHO over 10 minutes. The individual frames were taken every 10 seconds, so each row of snapshots corresponds to almost three and a half minutes of observations.
Each frame shows a spectrum of the light coming from a small patch on the solar disc: the height of each frame measures 84,000 km, which is about a sixteenth of the Sun’s diameter.
The bright red and yellow regions in each frame correspond to boundaries between different cells in the granular pattern of the Sun. In the first few frames of the series, the shape of the central bright region is roughly vertical, a sign that the underlying boundary was in a quiet state.
After only a couple of minutes, however, the situation changed dramatically: towards the end of the first row and at the beginning of the second row, the shape of the bright region appears stretched towards the right. This shift is characteristic of a jet of plasma that is receding from the observed boundary at a speed of about 100 km/s.
The following snapshots report how the same boundary went back to a quiescent state, then underwent the launch of a new jet and became quiet once again. These rapid changes, and the powerful events causing them, indicate the highly dynamic nature of the Sun’s atmosphere.
The data shown in Figure 49 were collected on 28 March 1996 and this image was featured in the series of images “The Sun as Art” published on the SOHO website.

Figure 49: The abstract science of the dynamic Sun (image credit: ESA/NASA/SOHO/The SUMER team, Max Planck Institute for Solar System Research)
• Oct. 31, 2013: Several large, active regions on the Sun burst out with about 20 eruptions between 25 and 28 October 2013. Some were flares; some were coronal mass ejections, and at least one was a prominence eruption. 57)
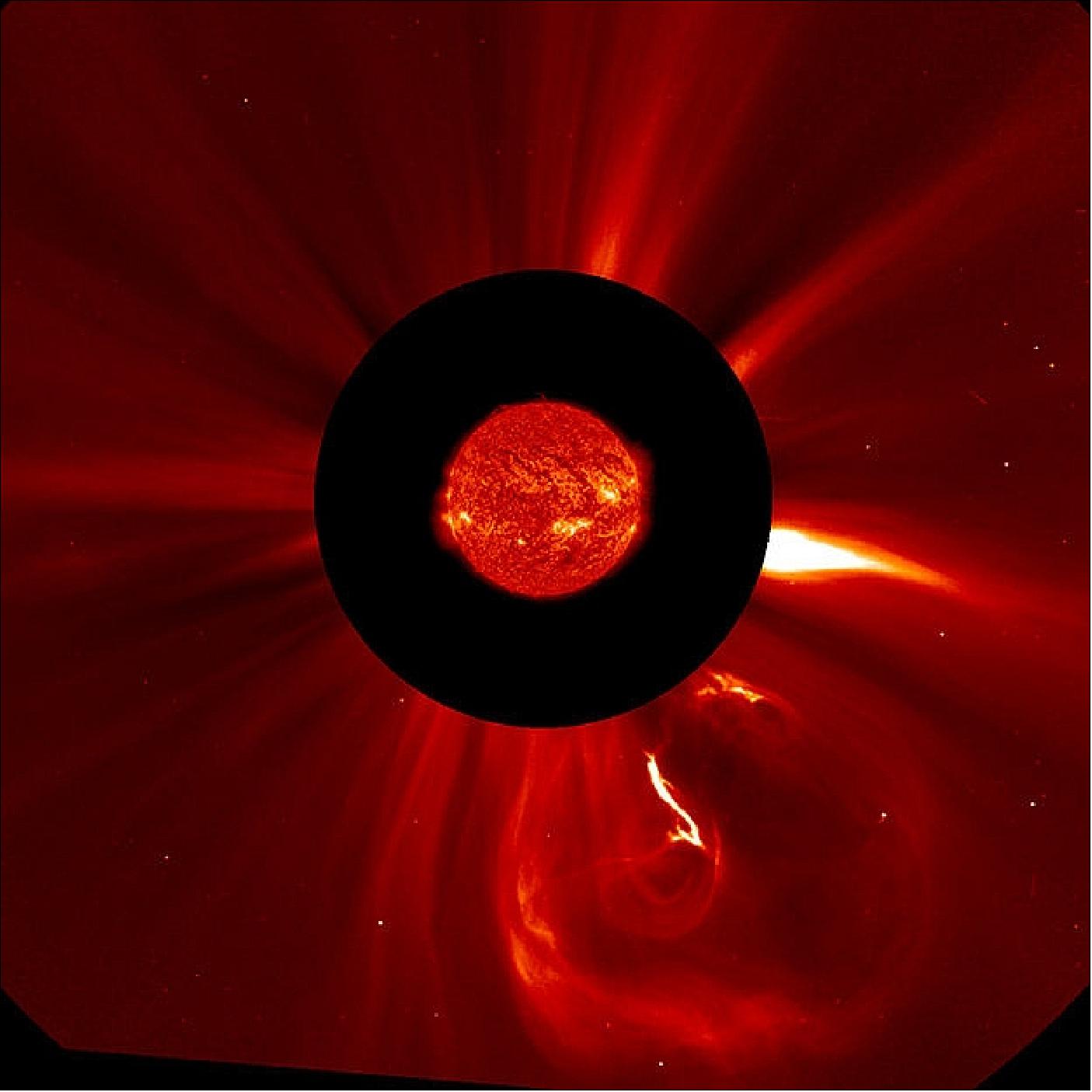
Figure 50: Image of the solar corona acquired with LASCO's C2 coronagraph on Oct. 26, 2013 (image credit: ESA, NASA)
Legend to Figure 50: This is an image of the stormy Sun in extreme UV light from the Solar Dynamic Observatory superimposed on a visible-light image of the solar corona obtained with LASCO's C2 coronagraph of SOHO. The Sun is about at its maximum level of activity in its 11-year solar cycle, so stormy stretches like this one are to be expected.
• On July 18,2013, the SOHO spacecraft mission of ESA and NASA captured an image of a gigantic coronal hole hovering over the sun’s north pole (Figure 51). Coronal holes are dark, low density regions of the sun’s outermost atmosphere, the corona. They contain little solar material, have lower temperatures, and therefore, appear much darker than their surroundings. 58) 59)
Coronal holes are a typical feature on the sun, though they appear at different places and with more frequency at different times of the sun’s activity cycle. The activity cycle is currently ramping up toward what is known as solar maximum, predicted for late 2013. During this portion of the cycle, the number of coronal holes decreases. During solar max, the magnetic fields on the sun reverse and new coronal holes appear near the poles with the opposite magnetic alignment. The coronal holes then increase in size and number, extending further from the poles as the sun moves toward solar minimum again. At such times, coronal holes have appeared that are even larger than this one.
The holes are important to our understanding of space weather, as they are the source of a high-speed wind of solar particles that stream off the sun, some three times faster than the slower wind elsewhere. While it’s unclear what causes the coronal holes, they correlate to areas on the sun, where magnetic fields soar up and away, failing to loop back down to the surface, as they do elsewhere.
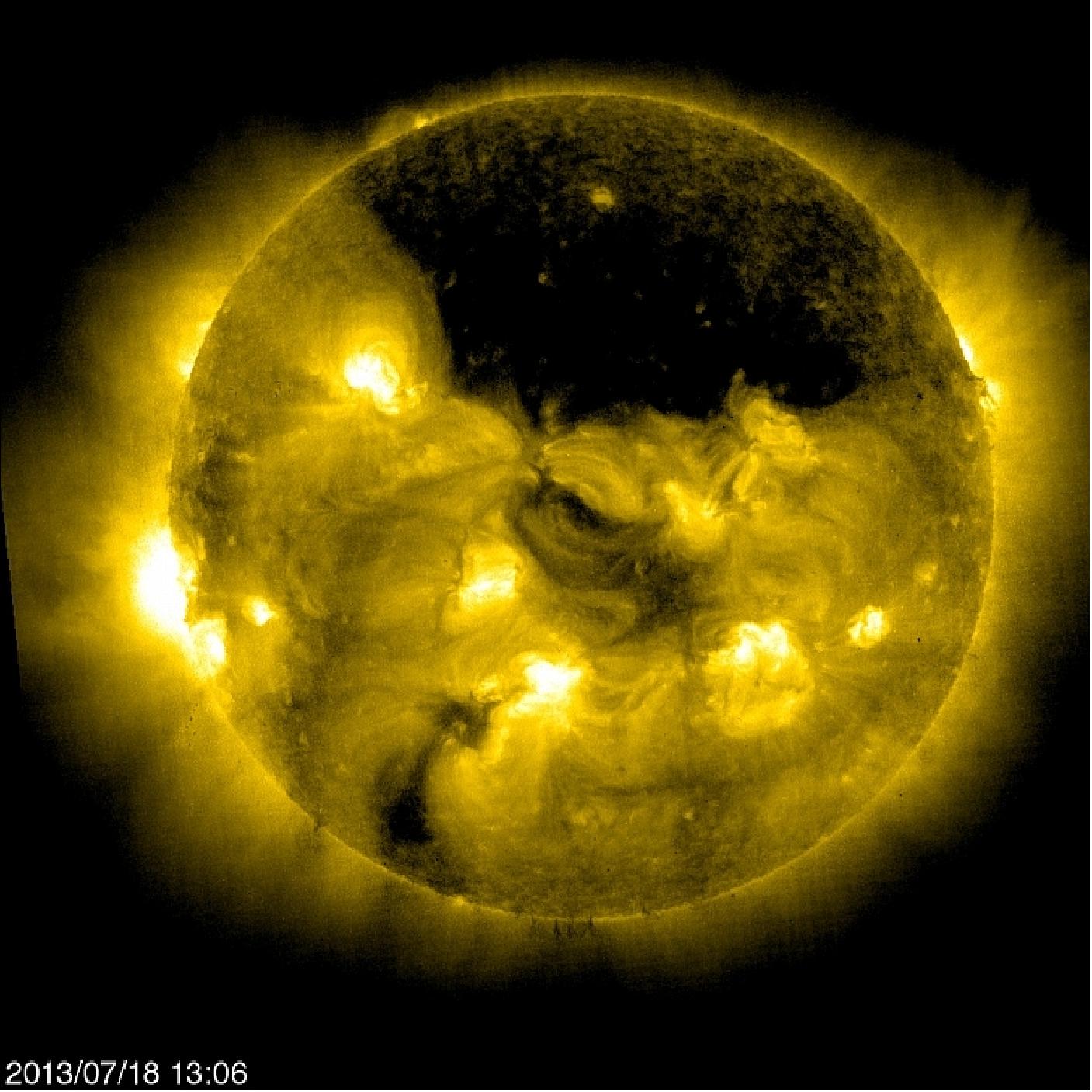
Figure 51: SOHO image of the sun with an extended coronal hole in the north pole region (image credit: ESA, NASA)
• Mission operations extension: On June 19, 2013, ESA's SPC (Science Program Committee) approved an extension of the SOHO mission until the end of 2016 - consistent with previous cycles, these are subject to mid-term confirmation, in late 2014. 60)
• On May 1, 2013, the the Sun blasted out a CME (Coronal Mass Ejection). In a gigantic rolling wave, this CME shot out about a billion tons of particles into space, traveling at over a 1.6 million km/hr. This CME is not headed toward Earth. 61)

Figure 52: Imagery from SDO, SOHO and its LASCO instrumentation of the May 1, 2013 coronal mass ejection (image credit: NASA/ESA)
Legend to Figure 52: This image shows three views of the CME from three different instruments. Left is the SDO (Solar Dynamics Observatory) image, taken at 02:40 UT. Center is from the SOHO spacecraft, looking through their coronograph instrument. The “mushroom” cloud of plasma leaving the Sun is visible. On the right is the LASCO C2 (red) and C3 (blue) instruments on SOHO, which use a disk to block out the Sun. Visible are the solid occulter disk, used to create a false eclipse; the “pylon”, which is an arm that holds the occulter disk in place; a representation of the Sun in the form of a white disk drawn on the occulter during the image processing and then one can see background stars and the cloud of plasma leaving the Sun (Ref. 61).
• In 2013, the SOHO mission is operational (the mission is extended to Dec. 31, 2014). 62) 63)
- ESA/NASA MOU (Memorandum of Understanding) to extend the mission to Dec. 31, 2016.
• In 2012, the SOHO mission is operational in its 17th year on orbit. The mission is extended to the end of 2014, subject to a mid-term review in 2012 (Ref. 65).
• On June 7, 2011, the Sun unleashed one of the most spectacular prominence eruptions ever observed. Based on real-time LASCO data, space weather forecasters could determine within hours that Earth would receive only a glancing blow from the associated CME (Coronal Mass Ejection), not a major space weather-induced event as hyped in the media. The event originated from the almost spotless active region 11226 and was associated with a moderate M2-class X-ray flare. The CME and associated shockwave produced an S1-class radiation storm, which shows up as speckles in the LASCO images. This event is not only one of the most spectacular ever recorded, but also one of the best observed, with complementary data from several spacecraft and different vantage points (SDO, SOHO, STEREO, PROBA-2). 64)

Figure 53: CME as recorded by SOHO’s LASCO instrument a few hours after the prominence eruption from the Sun’s surface on June 7, 2011 (image credit: NASA, ESA)
• The SOHO mission is operational in 2011. 65)
• On Dec. 2, 2010, the SOHO mission of ESA and NASA celebrated 15 years in orbit. During this time, SOHO has revolutionized what we know about the solar atmosphere and violent solar storms produced by the sun. 66)
• In November 2010, ESA's Science Program Committee (along with NASA) approved an extension of the SOHO mission for 4 years up to the end of 2014, subject to confirmation in late 2012 on the regular two-year cycle. The extension for SOHO will ensure that our Sun is closely watched during the rise to its next peak of magnetic activity, expected in 2013. 67)
• Since August 1, 2010, the EIT (Extreme-Ultraviolet Imaging Telescope) instrument has reverted back to its original observing mode, taking images of the Sun only twice per day. For almost 15 years, the EIT camera on board SOHO transmitted a picture of the solar corona every 12 minutes, providing ground-breaking observations of the Sun that changed our perception and understanding of our star. 68)
- This telescope was originally intended as a mere viewfinder, to set the context for data collected by the two spectrographs. As such, EIT was supposed to take images of the corona twice a day. Only after having seen the Sun with EIT did the project fully realize the enormous scientific potential of the instrument. This is why it was decided shortly after launch to increase the cadence of the snapshots to one every 12 minutes. EIT is probably SOHO's most popular workhorse, having taken over 500,000 snapshots of the Sun.
- EIT’s observations have strongly influenced the evolution of solar physics, paving the way for NASA's SDO (Solar Dynamics Observatory) mission, launched on Feb. 11, 2010. Thanks to substantial improvements in technology, the AIA (Atmospheric Imaging Assembly) instrument with an array of ultraviolet telescopes on board SDO is able to monitor the Sun at much higher cadence and at much greater resolution than SOHO's EIT. After a few months of joint operations to cross-calibrate the instruments, it was time for EIT to pass the baton to its successor (Ref. 68).
• The SOHO mission is operating nominally in 2010 (in its 15th year on orbit). On Oct. 7, 2009, ESA's Science Program Committee approved the extension of the SOHO mission operations to Dec. 31, 2012. This mission extension will enable to cover the totality of a solar cycle. 69) 70)
• The SDO (Solar Dynamics Observatory) of NASA was launched on Feb. 11, 2010. SDO is considered to be the next generation mission and successor to SOHO. It is expected that the SOHO mission will be continued at least as long until the SDO mission has become operational so that a period of cross calibration between the instruments of both spacecraft can be obtained.
• On Nov. 23, 2009, ESA's new SOHO science archive became online allowing for seamless access to the complete archive of science data sets from the 12 instruments as soon as the data are processed. The new facility improves access to SOHO data as it provides additional capabilities to search and visualize data. 71)
• On Dec. 2, 2007, SOHO completed its 12th year in orbit. The satellite has witnessed the sun change through almost a complete solar cycle - from quiet to stormy, and back again. The solar cycle normally lasts about 11 years. In late 1996, shortly after its launch, SOHO was able to observe the last minimum of the 11-year activity cycle. The minimum was followed by a rapid rise in solar activity, peaking 2001 and 2002. - Activity levels have slowly declined since then, but we haven't reached solar minimum yet, despite passing 11.1 years since the last minimum - the average length of a solar cycle. 72)
• Originally, SOHO was meant to operate only until 1998; but the mission was so successful that ESA and NASA decided to prolong its life until 2007 (the 1st mission extension was until 2003). This will allow SOHO to operate for a full 11 year solar cycle, from a minimum (a few dark sunspots) through a maximum to minimum again. A key objective of this SOHO Solar Cycle Mission is to better understand the cyclic activity of the sun, from changes in its interior through its atmosphere and out into interplanetary space.
• At the SPC (Science Program Committee) meeting in May 2006, a further extension of the SOHO mission was approved pushing back the mission end date from April 2007 to December 2009. 73)
• On Dec. 2, 2005, the SOHO spacecraft was 10 years on-orbit. 74) 75)
Since its launch on December 2, 1995, SOHO has revolutionized our understanding of the Sun. It has provided the first images of structures and flows below the Sun’s surface and of activity on the far side. SOHO has revealed the Sun’s extremely dynamic atmosphere, provided evidence for the transfer of magnetic energy from the surface to the outer solar atmosphere, the corona, through a ‘magnetic carpet’, and identified the source regions of the fast solar wind. It has revolutionized our understanding of solar-terrestrial relations and dramatically improved our space weather-forecasting by its continuous stream of images covering the atmosphere, extended corona and far side. The findings are documented in an impressive number of scientific publications: over 2500 papers in refereed journals since launch, representing the work of over 2300 individual scientists. At the same time, SOHO’s easily accessible, spectacular data and fundamental scientific results have captured the imagination of the space science community and the general public alike. As a byproduct of the efforts to provide real-time data to the public, amateurs now dominate SOHO’s discovery of over 1100 Sun-grazing comets.
• For two weeks in October-November 2003, the Sun featured three unusually large sunspot groups, which gave rise to 11 X-class flares – the most energetic class of flare – including the strongest ever recorded (Ref. 38).

Figure 54: Imagery of the erupting Sun in October 2003 (image credit: ESA, NASA)
Legend to Figure 54: First left: giant sunspot groups seen by MDI on 28 October 2003 in visible light. Second: MDI magnetogram on the same day, illustrating the magnetic complexity of these active regions. Third: EIT image at the time of an X-ray flare, seen as the bright emission just below the center of the disk. The linear horizontal feature is an artefact due to saturation of the CCD detector.
Fourth: LASCO C2 image at minutes after the flare, with a ‘halo CME’ completely surrounding the occulting disc. Fifth: LASCO C3 image of the expanding halo CME, where energetic particles hitting the CCD detector appear like ‘snow‘. The flare location and the halo were a clear indication that the CME was heading towards Earth.
• In May 2003, the SOHO team recorded signs of a possible breakdown in the east-west pointing mechanism of the high-gain antenna (HGA) [the Z-axis (azimuth) mechanism of the HGA is no longer working in the nominal operational mode]. The project feared that the mission was again in danger. After a long and careful analysis of all options, the team once more found a solution. The project decided to 'park' the antenna in an ideal position (where data losses are minimized), by rotating the spacecraft 180º every three months. In addition, the team established new procedures and the use of larger ground antennae (when available) to all but eliminate the impacts to normal science operations.
• SOHO mission recovery: Operational control of the SOHO spacecraft was lost on June 25, 1998 (when SOHO spun out of control and communication was lost). The first successful attempts to regain control were conducted on Sept. 16, 1998. The S/C was directed to turn its face and the solar power panels fully towards the sun (in addition, failure of three S/C gyros during the recovery period). Instrument re-commissioning started on Oct. 5, 1998 with SUMER and ended with CELIAS on Oct. 24, 1998. Final recovery from ESR (Emergency Sun Re-acquisition mode) and full S/C operation of SOHO was regained Feb. 1, 1999. A gyroless mode of operation was devised and installed with a new software patch for a modification of the AOCS (Attitude and Orbit Control Subsystem), making SOHO the first three-axis-stabilized S/C of ESA to be operated without a gyroscope. 76) 77) 78)
Prior to 1999, a gyro-based roll control mode was used when a SEU (Single Event Upset) occurred. Now the roll controller input is changed to one of the other stars still being tracked, taking into account the difference in position on the CCD between the original and the new guide star. With this new approach the spacecraft has survived all major solar flares during the last solar maximum without any fall backs to the new CRP (Coarse Roll Pointing) mode. This would have been very unlikely with the original design. 79)
The needed roll measurement in the new CRP mode is now implemented using the so-called CRS (Coarse Roll Sensor). It uses the on-board wheel momentum computed from the measured wheel speeds. The principle is illustrated in Figure 55 and is based on the conservation of angular momentum. Since the spacecraft rates around the pitch (Y) and yaw (Z) axes are being controlled to be zero using the FPSS, the spacecraft momentum in the transverse Y-Z plane is coming from the wheels. If the spacecraft is rotating slowly around the roll (X) axis then the transverse component of the inertial momentum vector will rotate in the opposite direction in the spacecraft Y-Z plane. By computing the component of the wheel momentum in the Y-Z plane from the measured wheel speeds and the known wheel inertia the evolution of the roll angle can be followed from the initial reference measurement (Ref. 79).
Before 1998 thruster maneuvers (station keeping and momentum management) were performed in a specific mode with the thrusters providing both attitude control and ΔV. The ΔV thruster pair (only use of the sunward and anti-sunward ΔV) was operated with off-modulation using a 75% duty cycle. The other thrusters were operated in on-modulation for attitude control. Momentum management was performed by commanding the wheels to their desired speeds using a simple wheel speed control loop and letting the thrusters counteract the attitude disturbances caused by the changing wheel momentum. Obviously this was not going to work without a gyro.
Now, the project uses a wheel based mode with the FPSS and SSU as sensors and command the thrusters in open loop using pulse trains with a very low duty cycle. The duty cycles are chosen such that the disturbance rates stay within the capability of the SSU. The SSU has a roll rate limit of 40 arcsec/sec. Also the pauses between the individual pulses have to be long enough for the controller to recover from the thruster pulse disturbance. For station keeping a duty cycle of approximately 5% is used with a pulse length of a little less than 1 sec and a period of 20 sec. For momentum management, an axis by axis approach is employed where a single thruster pulse train is fired causing a disturbance which is then counteracted by the wheel based control, driving the wheel speeds towards their targets. This is exactly the opposite from the pre-gyroless momentum management. Of course, the duration of the maneuvers is longer than before 1998 due to the low duty cycles. However when doing regular station keeping to maintain the semi-stable halo orbit, only a very small ΔV is required.
The total hydrazine fuel used during 2008 is less than 1 kg. The current (January 2009) fuel estimate is 118 kg of hydrazine (Ref. 79).
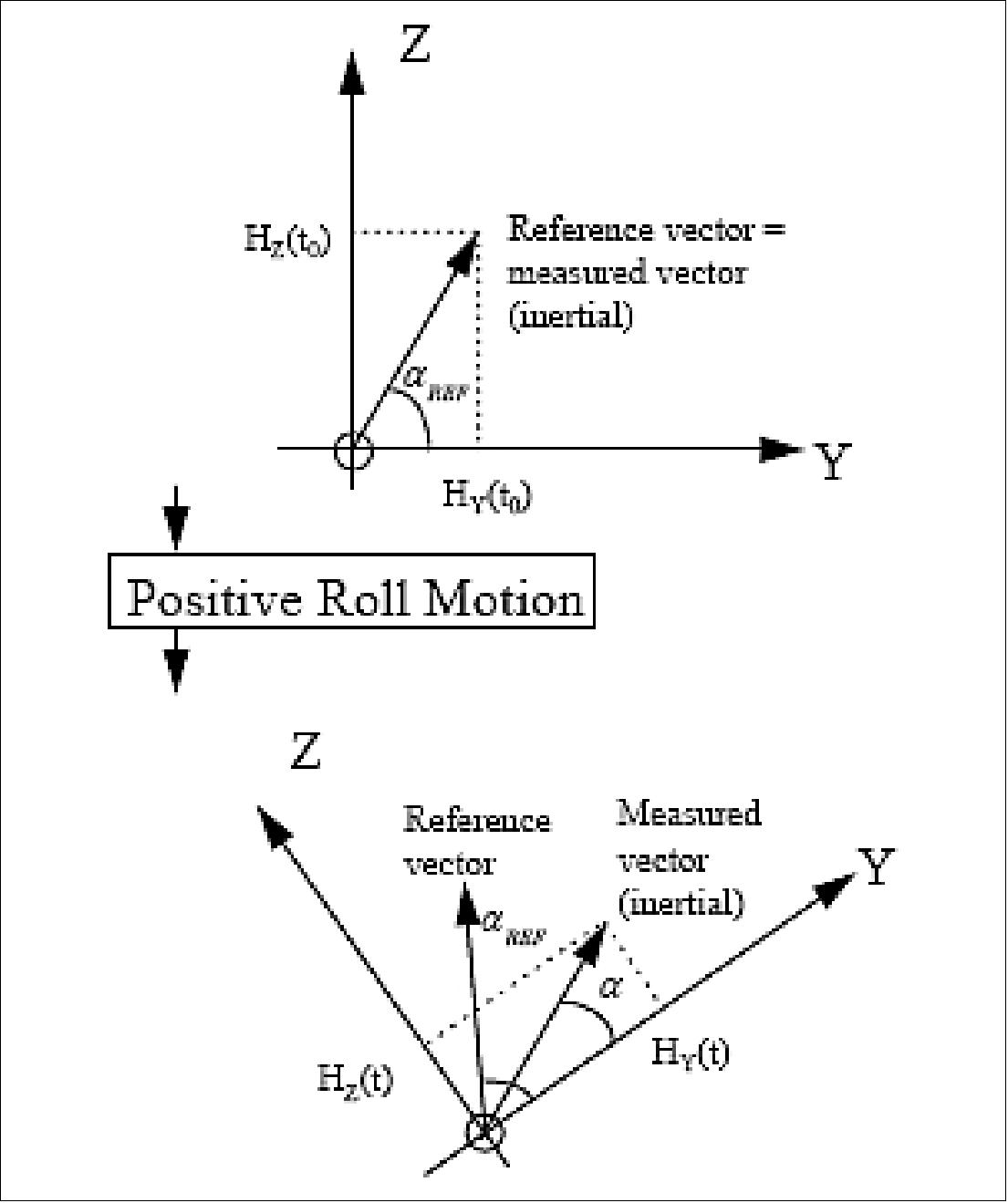
Figure 55: Illustration of the CRS principle (image credit: ESA, NASA)
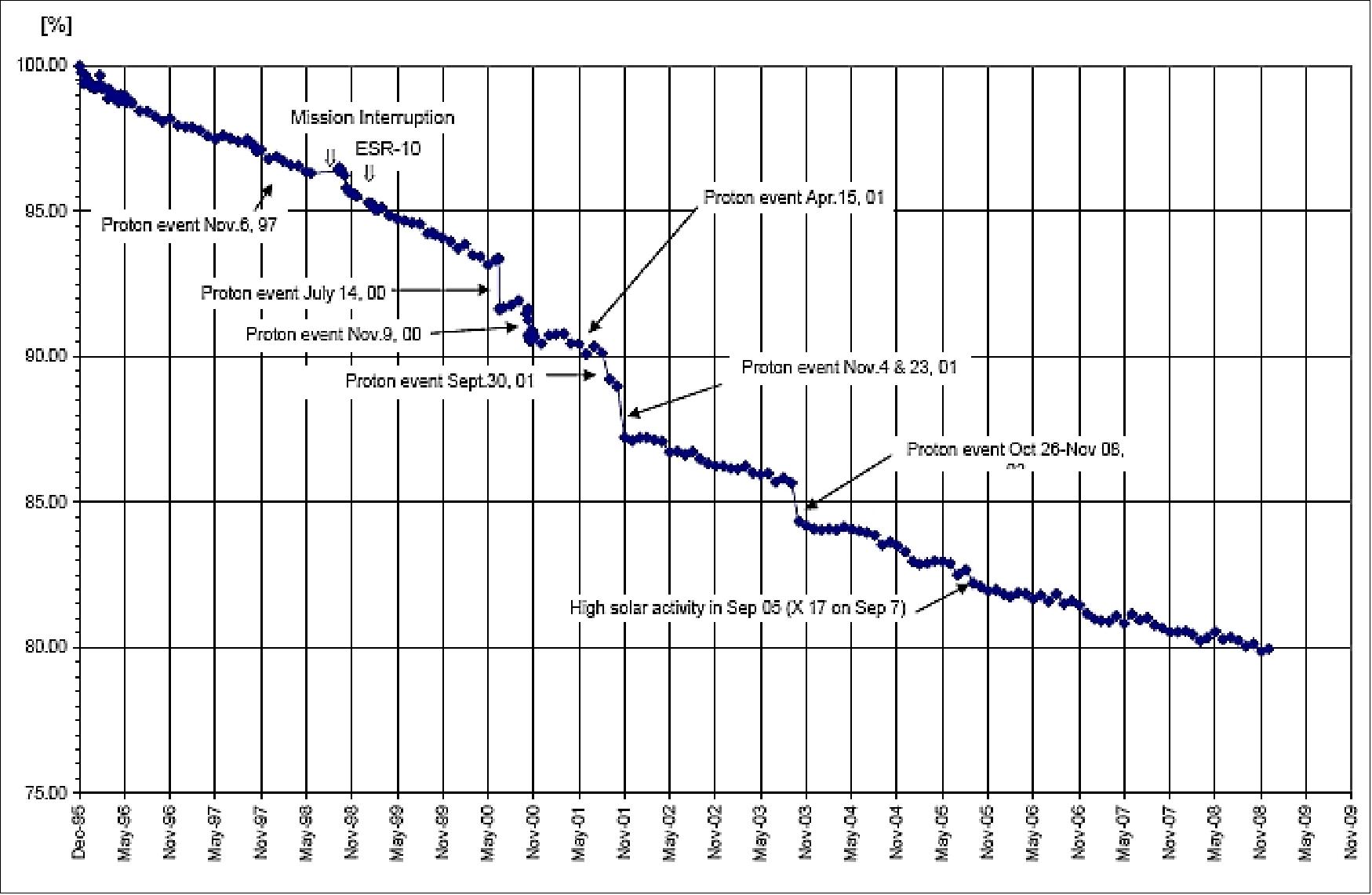
Figure 56: SOHO solar array degradation based on the average of two section currents (image credit: ESA, Ref. 79)
• SOHO was launched in December 1995 by an Atlas Centaur rocket; it arrived at its L1 orbit in February 1996 and became operational in March 1996.
Sensor complement
The scientific payload of SOHO comprises 12 complementary instruments, developed and furnished by 12 international consortia involving 29 institutes from 15 countries. Nine consortia are led by European scientists, the remaining three by US scientists. More than 1500 scientists in countries all around the world are either directly involved in SOHO's instruments or have used SOHO data in their research programmes. 81) 82) 83)
Instrument/Experiment | PI (Principal Investigator) | Objective |
Solar atmosphere remote sensing instrument package | ||
SUMER (Solar UV Measurement of Emitted Radiation) | K. Wilhelm, MPAe, Lindau, Germany | Plasma flows, temperature and density in the solar atmosphere |
CDS (Coronal Diagnostic Spectrometer) | R. A. Harrison, RAL, Chilton, UK | Density, temperature and flows in the solar atmosphere |
EIT (Extreme UV Imaging Telescope) | J. P. Delaboudinière, IAS/CNRS, Orsay, France | Evolution of low coronal structure and activity |
UVCS (UV Coronagraph and Spectrometer) | J. L. Kohl, SAO, Cambridge, MA, USA | Density and temperature in the corona |
LASCO (Large Angle Spectrometer Coronagraph) | G. E. Brueckner, NRL, Washington DC, USA | Structure and evolution of the corona |
SWAN (Solar Wind Anisotropies) | J.-L. Bertaux, CNRS, Paris, France | Solar wind mass flux anisotropies |
Solar wind “in situ particle” instrument package | ||
CELIAS (CHarge, Element, Isotope Analysis) | D. Hovestadt, MPE, Garching, Germany | Ion composition in the solar wind |
COSTEP (Suprathermal & Energetic Particle Analyzer) | H. Kunow, University of Kiel, Kiel, Germany | Ion and electron composition in the solar wind |
ERNE (Energetic and Relativistic Nuclei and Electron experiment) | J. Torsti, University of Turku, Finland | To detect charged particles in the solar atmosphere produced in various solar energy release processes |
Helio-seismology instrument package (study of the sun's interior) | ||
GOLF (Global Oscillations at Low Frequencies) | A. Gabriel, IAS/CNRS, Orsay, France | Global sun velocity and magnetic field oscillations |
VIRGO (Variability of Solar Irradiance) | C. Fröhlich, WRC, Davos, Switzerland | Global and low resolution imaging of oscillating and solar constant |
MDI (Michelson Doppler Imager) | P. H. Scherrer, Stanford University, Stanford, CA, USA | High resolution imaging of velocity oscillations |
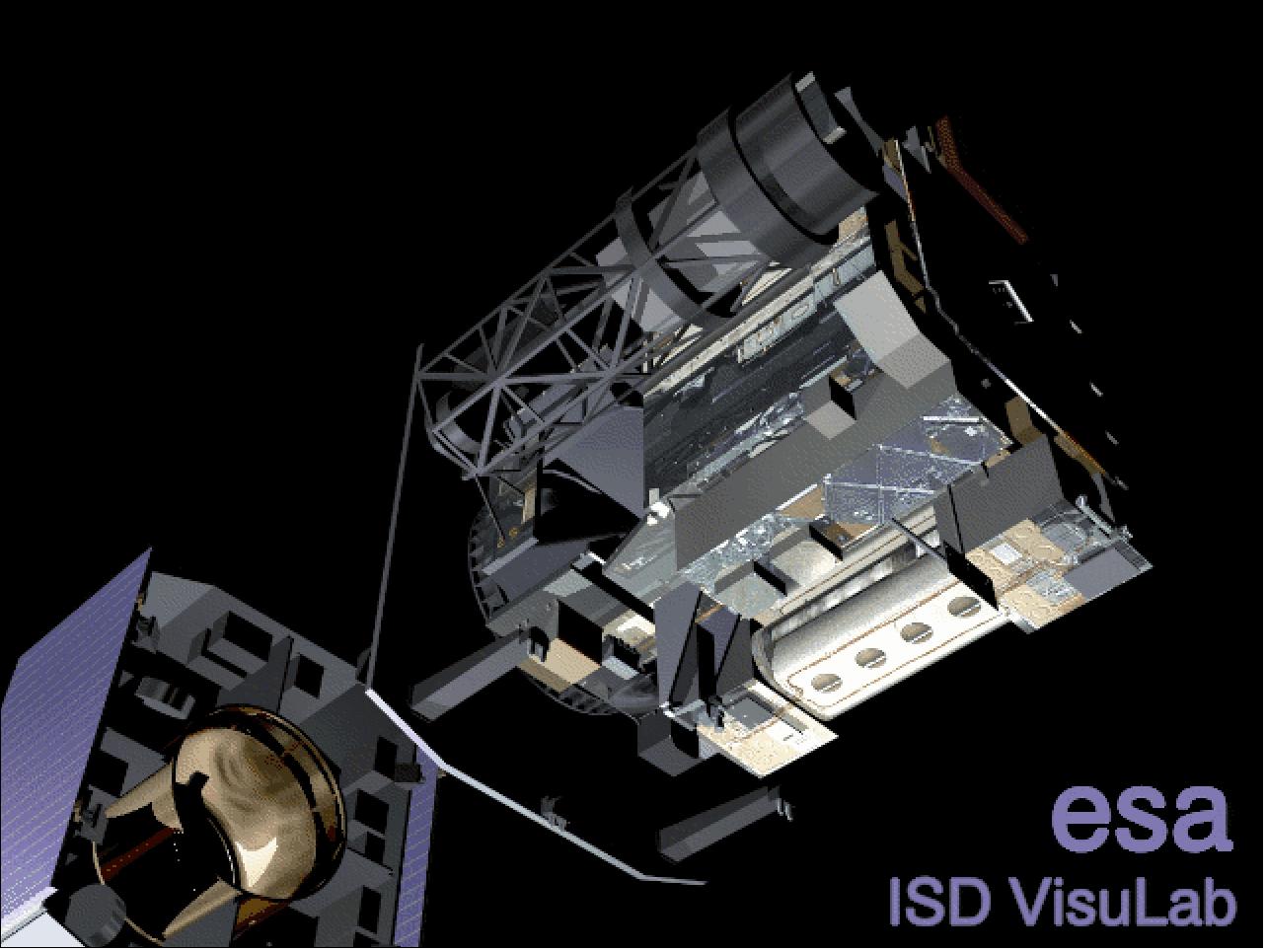
SUMER (Solar Ultraviolet Emitted Radiation)
SUMER PI: K. Wilhelm, MPAe, Katlenburg-Lindau, Germany (international cooperation of MPAe, IAS, NASA/GSFC, ETH Zürich, AIT Tübingen, PTB Berlin, SSL/UCB, ESA). Study of plasma flow characteristics (turbulent motions, waves. temperatures, and densities of the plasma in the upper atmosphere of the sun). SUMER consists of a UV telescope and a spectrometer, taking images of the sun in EUV (Extreme Ultraviolet) light with high resolution in space, wavelength and time. Note: In 2004 MPAe changed its name to MPS (Max Planck Institute for Solar Research).
The instrument characteristics are: 84)
• Single parabolic mirror telescope. Pointing and scanning is being performed by a mirror mechanism, which has a 2-D scan capability of ± 32 arcmin. One step is equivalent to 0.76 arcsec; half steps are possible. The east-west scan mechanism has to be operated in high-current mode (provided by the redundancy concept of SUMER) since autumn 1996. In order to maintain this capability, east-west raster scans are no longer permitted with SUMER. Pointing and rotation compensation procedures, however, can be employed without restrictions.
• Normal incidence spectrometer with the grating in a Wadsworth mount. Wavelength range: 500-1610 Å (or 50-161 nm). Scans can be performed within 21 s over the full range.
• Two 2-D microchannel plate detectors with cross delay-line anodes in photon-counting mode; KBr and bare microchannel plate photocathode.
• Spatial resolution: 1.2-1.5 arcsec; the pixel size corresponds to approximately 1 arcsec.
• Spectral resolution: lambda/delta lambda = 19,000 to 40,000, where delta lambda corresponds to the pixel size. Sub-pixel accuracy is achievable for the determination of line shifts and widths.
• Typical minimum sampling times: 3 to 10 s.
• Doppler velocities down to 1 km/s can be measured separately for each spatial resolution element.
• Rear slit camera for monitoring the slit position on the solar disk.
Data: on-line profiles, shifts and broadenings, ratios of temperature- and density-sensitive EUV emission lines in the range between 104 and 2 x 106 K.

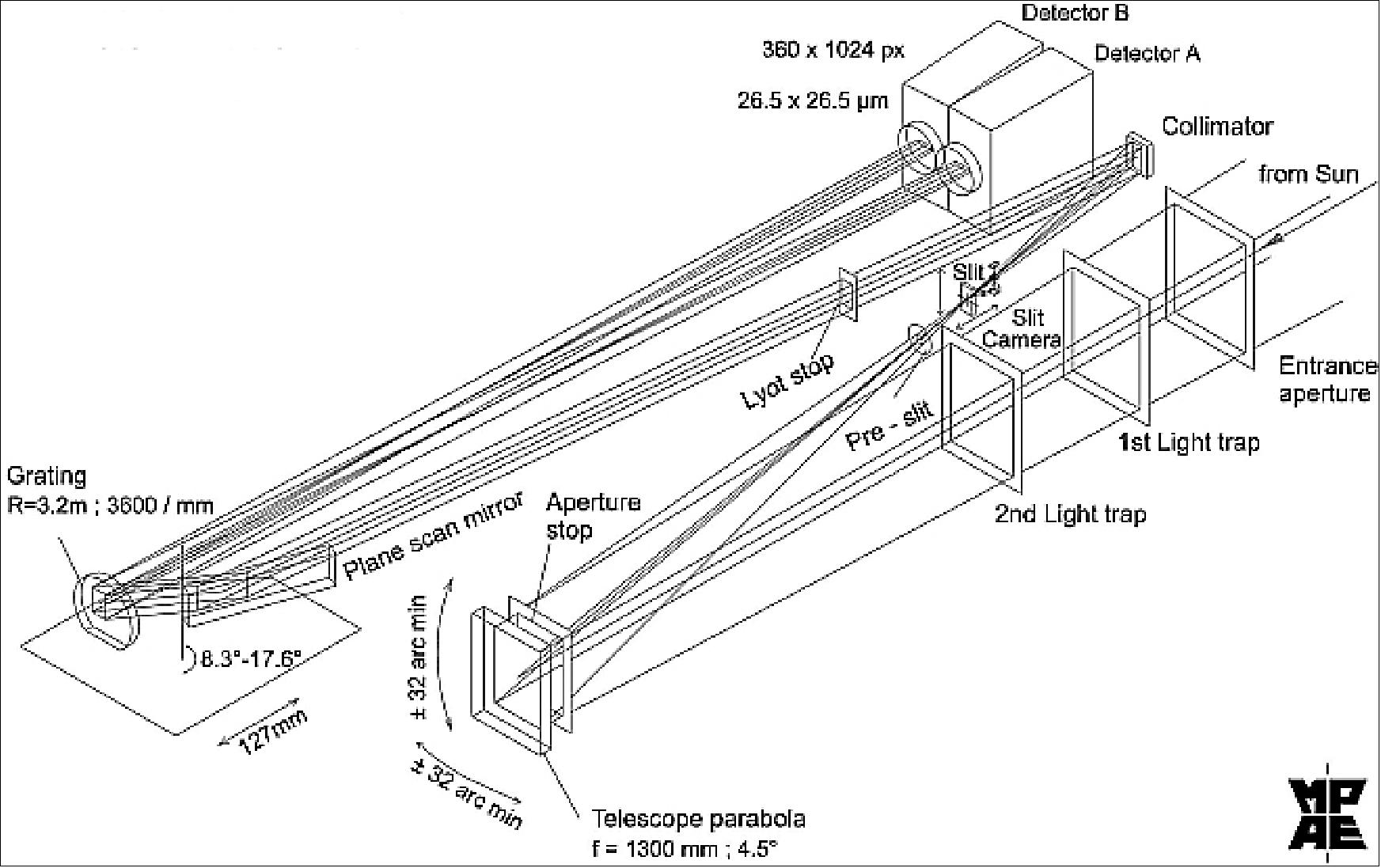
CDS (Coronal Diagnostic Spectrometer)
CDS PIs: R. A. Harrison, A. Fludra, RAL, Chilton, UK. Study of the transition region and corona temperature and density (intensity ratios of selected EVU line pairs, with spatial and temporal scales appropriate to the fine-scale features of the solar atmosphere). There is close collaboration and correlation with SUMER. 85) 86) 87) 88) 89) 90) 91)
The instrument features a Wolter-Schwarzschild type II grazing incidence telescope [designed by MPE Garching, outer f-number = 9.48, focal length = 257.83 cm, FOV = 4 arcmin, on-axis resolution = 2 arcsec (FWHM)], a normal incidence spectrometer (NIS) with a wavelength = 2 bands in 240 - 800 Å range, and a grazing incidence spectrometer (GIS) with a wavelength = four bands in the 150 - 800 Å range.



EIT (Extreme-Ultraviolet Imaging Telescope)
EIT PI: J. P. Delaboudinière, IAS (Institut d'Astrophysique Spatiale)/CNRS, France [international collaboration, NASA/GSFC, NRL, ROB (Royal Observatory), Belgium, IOTA, CSL, Belgium, LAS, France, etc.]. Study of the evolution of chromospheric and coronal structures (identification and interpretation of the spatial and temperature fine structures of the solar atmosphere). EIT provides full disk images in emission lines formed at temperatures that map the solar structures. Images in four narrow band passes are obtained using normal incidence multilayered optics deposited on the quadrants of a Ritchey-Chrétien telescope. Temperature range of solar structures to be measured: 6 x 104 - 3 x 106 K. Resolution = 1 arcsec (uniform over FOV = 50 x 50 arcmin). EIT is able to measure the solar transition region and inner corona in four, selected bandpasses in the extreme ultraviolet (EUV): 92)
Wavelength | Ion | Peak temperature | Observational objective |
304 Å | He II | 8.0 x 104 K | Chromospheric network, coronal holes |
171 Å | Fe IX-X | 1.3 x 106 K | Corona7transition region boundary, structures inside coronal holes |
195 Å | Fe XII | 1.6 x 106 K | Quiet corona outside coronal holes |
284 Å | Fe XV | 2.0 x 106 K | Active region |
Using either full-disk or subfield images, the EIT can image active regions, filaments and prominences, coronal holes, coronal “bright points,” polar plumes, and a variety of other solar features. EIT was designed to be used in conjunction with other instruments, in particular LASCO, SUMER and CDS.


Telescope | Mirrors | - Multilayer-coated Zerodur |
CCD detector (cooled to ~-80ºC) | Architecture | - Three-phase, multiphase pinned, back-illuminated,enhanced EUV sensitivity |
Filters | Entrance filter | - 1500 Å Al/700 Å cellulose/1500 Å Al |
Telemetry | Normal average rate | - 1 kbit/s |
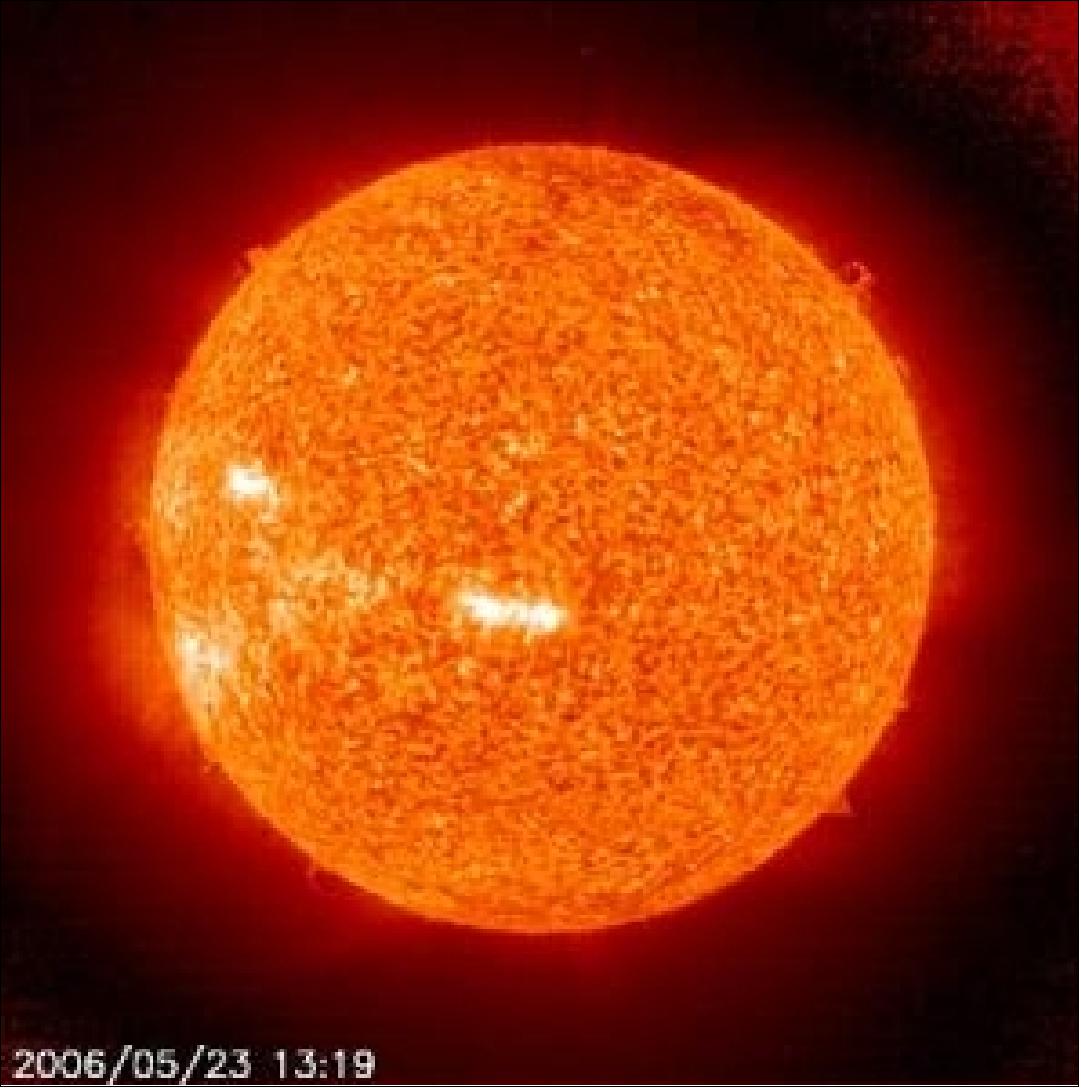
EIT is the first long-duration instrument to use normal incidence multilayer coated optics to image the sun in extreme ultraviolet. This portion of the spectrum is extremely difficult to reflect, as most matter absorbs the light very strongly. Conventionally these wavelengths have been reflected either using grazing incidence (as in a Wolter telescope for imaging X-rays) or a diffraction grating (as in the infamous overlappograph flown on Skylab in the mid-1970s.
UVCS (Ultra-Violet Coronograph Spectrometer)
UVCS PI: J. L. Kohl, SAO (Harvard-Smithsonian Astrophysical Observatory), Cambridge, MA, USA. Study of electron and ion temperatures, densities and velocities in the corona (for interpretation of coronal heating, solar wind acceleration, and solar wind composition). Spectroscopic measurements of the solar corona out to 10 solar radii from the sun's center (detailed description of the extended solar corona that can be used to address a broad range of scientific questions regarding the nature of the solar corona and the generation of the solar wind. 93) 94)
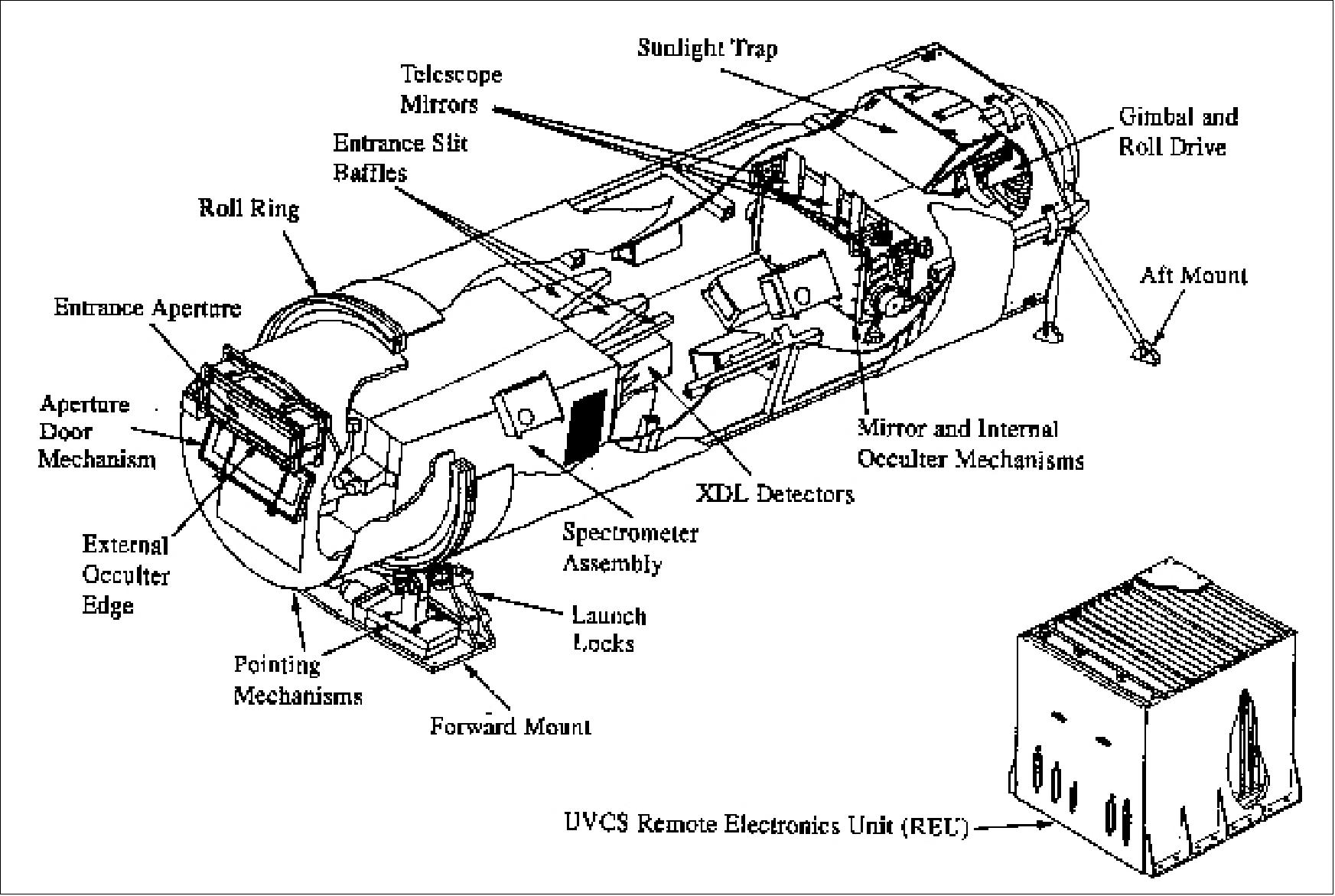
The UVCS instrument consists of an occulted telescope and a high resolution spectrometer assembly. Three off-axis paraboloidal mirrors focus co-registered images of the extended corona onto the three entrance slits of the spectrometer assembly. The spectrometer has three sections. One section is optimized for line profile measurements of H I Lyman-α; its wavelength range is 1148 - 1248 Å. Another section is optimized for line intensity measurements of O VI lambda 1032; it is also used to observe Si XII lambda 499 and 521 in second order. The first order range is 932 - 1068 Å. The Mg X doublet at lambda 610 and lambda 625 may be observed in first order. The third section is used to measure polarized radiance of the visible corona.
Telescope: off-axis parabolic mirror, focal length = 750 mm, image scale = 0.218 mm/arcmin, FOV = 42' (tangential) x 141'.
Spectrometer: gratings = 105 (disp) x 70 mm; nominal radius = 750 mm; radius ratio = 1.0259 (1216 Å), = 1.0215 (1032 Å, 625Å), = 1.0040 (visible); ruling frequency = 2400 mm-1; reciprocal dispersion = 5.63 Å/mm (1st order), = 2.82 Å/mm (2nd order).
Spectral Line | Observed Quantity | Spectral Resolution | Spatial Resolution |
H I 1216 | Profile | 0.2 | 10” x 10” |
H I 1216 | e- Profile | 2.0 | 1.6' x 1' |
Fe XII 1242 | Intensity | 1.23 | 1' x 1' |
Fe XII 1242 | Profile | 0.14 | 10” x 5' |
O VI 1032/37 | Intensity | 1.23 | 1' x 1' |
O VI 1032 | Profile | 0.14 | 10” x 5' |
Si XII 499/521, 2nd order | Intensity | 0.61 | 1' x 1' |
Si XII 499, 2nd order | Profile | 0.07 | 7” x 5' |
Mg X 610/625, 2nd order | Intensity | 0.61 | 1' x 2.5' |
MG X 610, 2nd order | Profile | 0.07 | 7” x 5' |
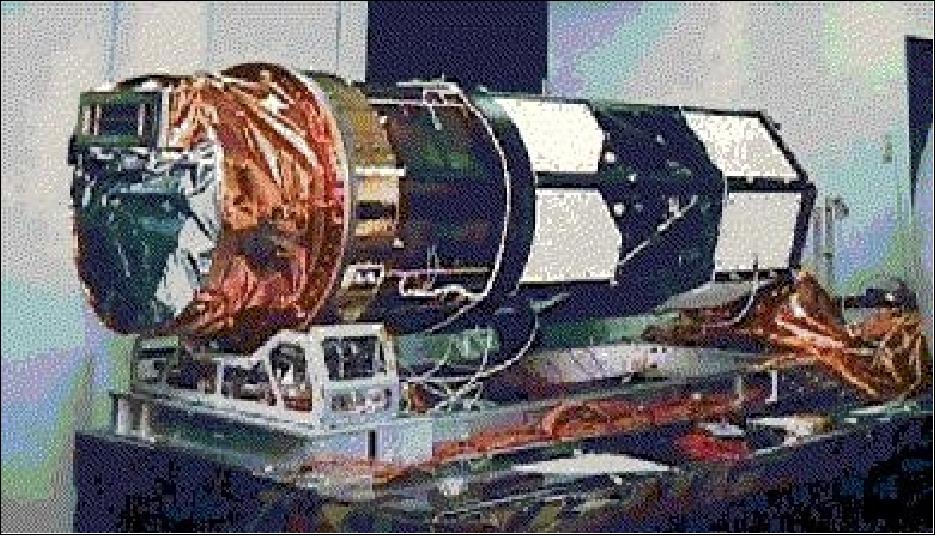
LASCO (Large-Angle and Spectrometric Coronograph)
LASCO PI: G. E. Brueckner, NRL, Washington DC, USA. LASCO was jointly developed by NRL, MPS, Katlenburg-Lindau, Germany, the LAS/CNRS (Laboratoire d'Astronomie Spatiale), Marseille, France, and the University of Birmingham, Birmingham, UK. Objectives: Study of structure evolution, mass, momentum and energy transport in the corona. Measurement of electron column densities from just above the limb, at 1.1 Rsun (solar radii - the solar radius is about 700,000 km), out into deep heliospheric space, at 30 Rsun. Spectral analysis of the inner corona with a high resolution scanning. imaging interferometer. Measurement of spectral profiles of three emission lines and one Fraunhofer line for each pixel (providing temperatures, velocities, turbulent motions and volume densities). Direction of coronal magnetic fields via polarization analysis. The LASCO instrument consists of the coronograph optical system (with three optical systems and CCD cameras) and the instrument control system. 95) 96)
LASCO takes white light and spectral images of the corona by blocking light from the sun with an occulting disk, mimicking an eclipse. The three telescopes, comprising LASCO, are designated: C1 (Coronagraph 1), with coverage from 1.1 to 3.0 Rsun, C2, with coverage deliberately overlapping parts of both C1 and C3, and extending from 2.0 to 6.0 Rsun, and the C3, which spans the outer corona from about 3.7 to 32 Rsun.
The inner corona instrument, C1, is a newly developed mirror version of the classic Lyot internally occulted coronagraph (Bernhard Lyot, 1897-1952, French astronomer, inventor of the coronagraph in 1930), while the C2 and C3 are externally occulted instruments. In addition, the C1 is fitted with an imaging Fabry-Perot interferometer, making possible spatially resolved high-resolution coronal spectroscopy in selected spectral emission and absorption lines, between 1.1 and 3.0 Rsun. High definition CCD cameras in each telescope provide detailed images with exceptional dynamic range, while large digital memories and a high-speed microprocessor support extensive onboard image processing and image data compression by large factors, that allow transmission of up to 10 full coronal images per hour.

Telescope | FOV R/Rsun | Occulter type | Spectral bandpass | Objective element | CCD array (pixels) | Pixel size | Brightness temperature range (TB) |
C1 | 1.1-3.0 | Internal | Fabry-Perot | Mirror | 1024 x 1024 | 5.6 arcsec | 2x10-5-2x10-8 |
C2 | 2.0-6.0 | External | Broadband | Lens | 1024 x 1024 | 11.4 arcsec | 2x10-7-5x10-10 |
C3 | 3.7-32 | External | Broadband | Lens | 1024 x 1024 | 56.0 arcsec | 3x10-9-1x10-11 |
SWAN (Solar Wind Anisotropies)
SWAN PI: J. L. Bertaux, CNRS, France). SWAN is a collaboration between Finnish Meteorological Institute (FMI) and Service d'Aeronomie (SA). Study of solar wind mass flux anisotropies and temporal variations. The SWAN instrument is a Lyman photometer which maps the sky's interplanetary hydrogen emission almost entirely every other day. From these sky maps, the latitude distribution of the solar wind mass flux from the equator to the pole can be measured, as well as the variation of this distribution (time scale≈one month). - SWAN is the only remote sensing instrument on SOHO that does not look at the sun. It watches the rest of the sky, measuring hydrogen that is 'blowing' into the solar system from interstellar space. By studying the interaction between the solar wind and this hydrogen gas, SWAN determines how the solar wind is distributed. As such, it can be qualified as SOHO's solar wind 'mapper'. 97) 98)
The instrument consists of two identical sensor units placed on opposite sides of the S/C and driven by a common electronic box. Each sensor consists of a two-mirror scanning system, a light baffle (to keep out stray light), a hydrogen cell in which a tungsten filament is alternately heated to produce Lyman-alpha absorbing hydrogen atoms and cooled to produce the reference signal. Resolution of the Lyman-alpha light distribution is 1º.
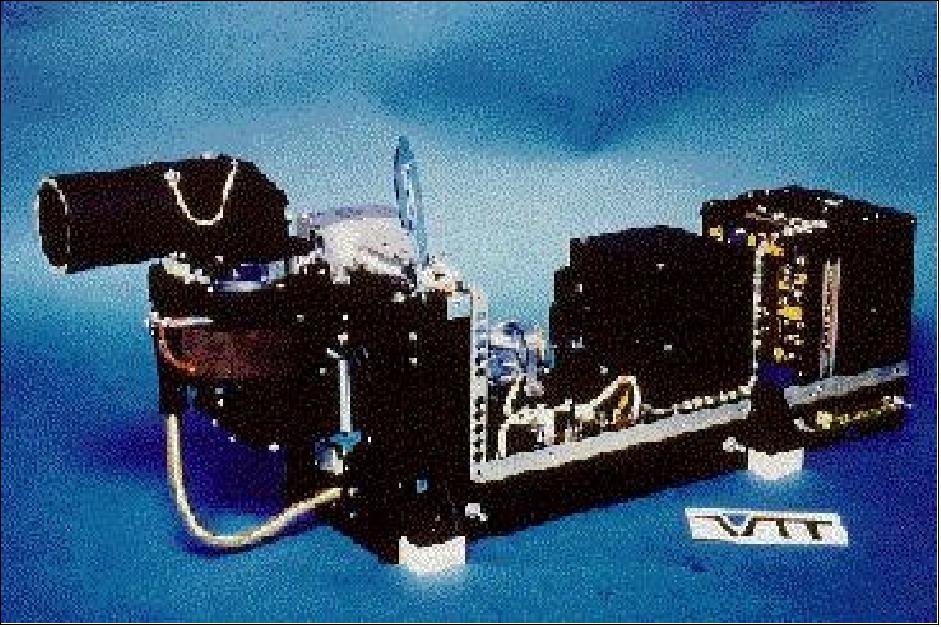
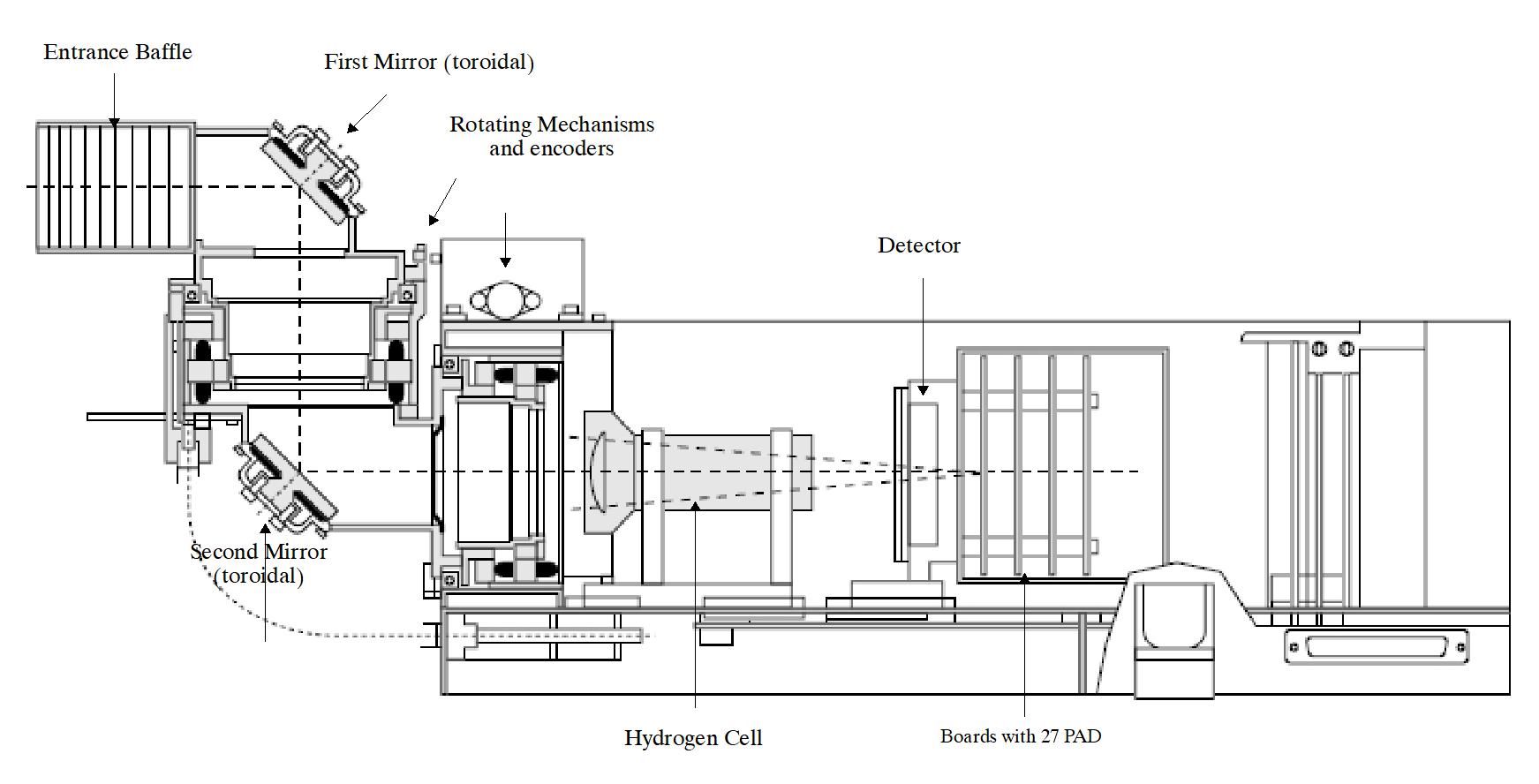
Total instrument mass, electronic unit mass | 13.25 kg, 2.82 kg |
Mass of one sensor unit | 4.815 kg |
Average power consumption | 11 W |
Source data rate | 200 bit/s |
Total FOV (Field of View) | > 2π sr for each sensor unit (2 mirror periscope) |
IFOV (Instantaneous Field of View) | 25 pixels of 1º x 1º = 5º x 5º |
Wavelength range | 115-180 nm |
Photometric sensitivity | 0.75 counts /s /Rayleigh /1º pixel |
Type of detector | Solar blind MgF2 / CsI Cathode - Multi-anode MCP |
Count rate | From 200/s (IP) to 104 /s (solar corona) per pixel |
Relative accuracy | Better than 1% for 45 s counting time on IP signal |
Absolute accuracy | Checked at regular time intervals with stellar calibrations |
Hydrogen cell | Pyrex vessel (MgF2 lens windows-distance 76 mm) |
CELIAS (Charge, Element and Isotope Analysis)
CELIAS PI: D. Hovestadt, MPE, Garching, Germany, Universität Bern, Switzerland. Study of ionic energy distribution and composition of the solar wind. Measurement of the mass, ionic charge and energy of the low and high speed solar wind, of suprathermal ions, and of low energy flare particles. 99) 100) 101) 102)
Sensor | Area, Geometric Factor | ΔE/E | Efficiency of the TOF unit |
CTOF (Charge Time-of-Flight) | 0.08 cm2 | 0.04 | 0.25 - 0.65 |
MTOF (Mass Time-of-Flight) | 0.013 cm2 | 0.03-5 | 0.03 - 0.1 |
PM (Proton Monitor) | 7 x 10-5 cm2 | 0.05 | - |
STOF (Suprathermal Time-of-Flight) | 0.1 - 0.2 cm2 sr | 0.1 | 0.2 - 0.8 |
CELIAS includes three mass- and charge-discriminating sensors based on the time-of-flight technique: CTOF (Charge Time-of-Flight) for the elemental, charge and velocity distribution of the solar wind; MTOF (Mass Time-of-Flight) for the elemental and isotopic composition of the solar wind, MTOF also includes a proton monitor (PM); and STOF (Suprathermal Time-of-Flight) for the mass, charge and energy distribution of suprathermal ions. Mass resolution: M/ΔM > 100 MTOF; charge resolution: ΔQ ≈0.3 - 1, 4 <M<60 (typical for CTOF and STOF)].
In addition there is SEM (Solar EUV Monitor), a highly stable photodiode spectrometer which measures the full solar disk absolute photon flux at the prominent and scientifically important He II 30.4 nm line, as well as the absolute integral flux between 17 and 70 nm.
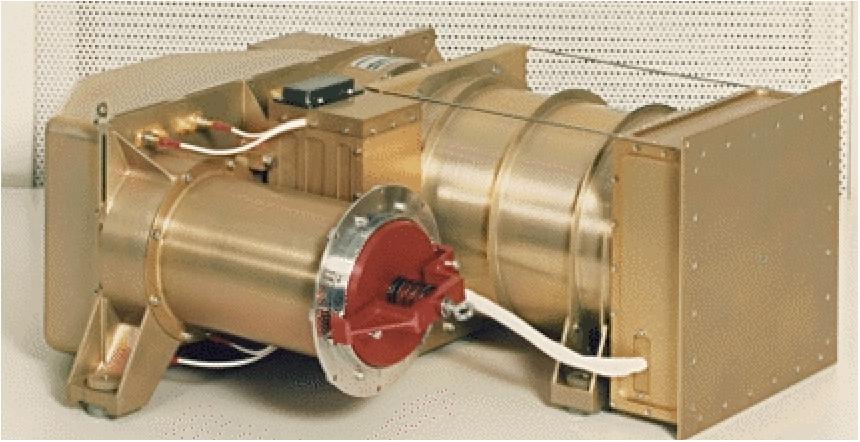
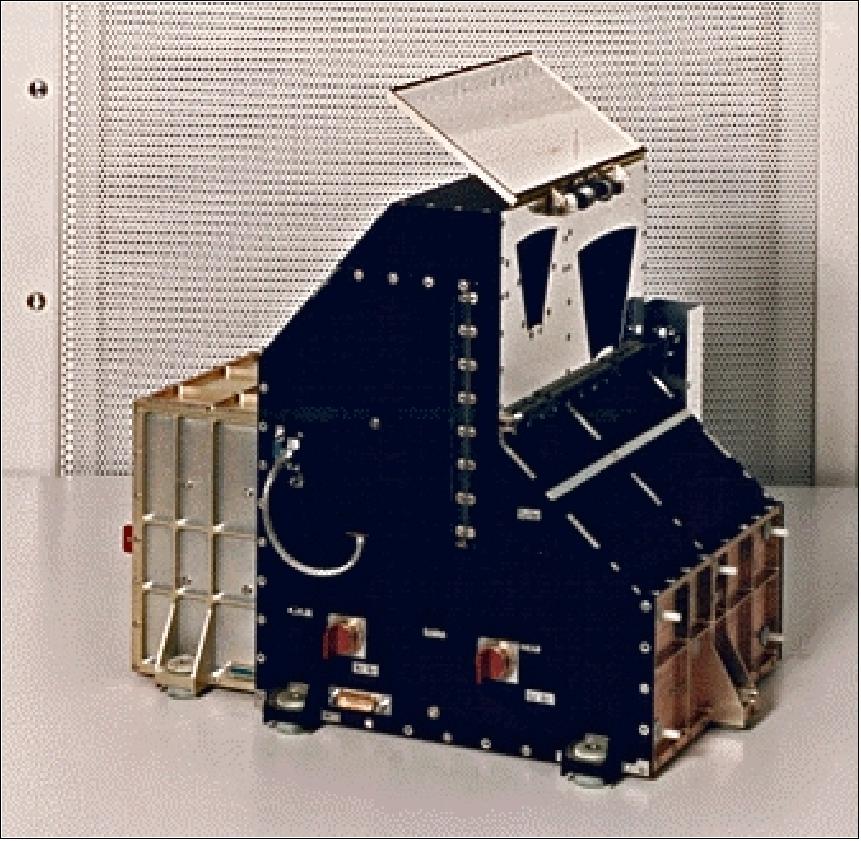
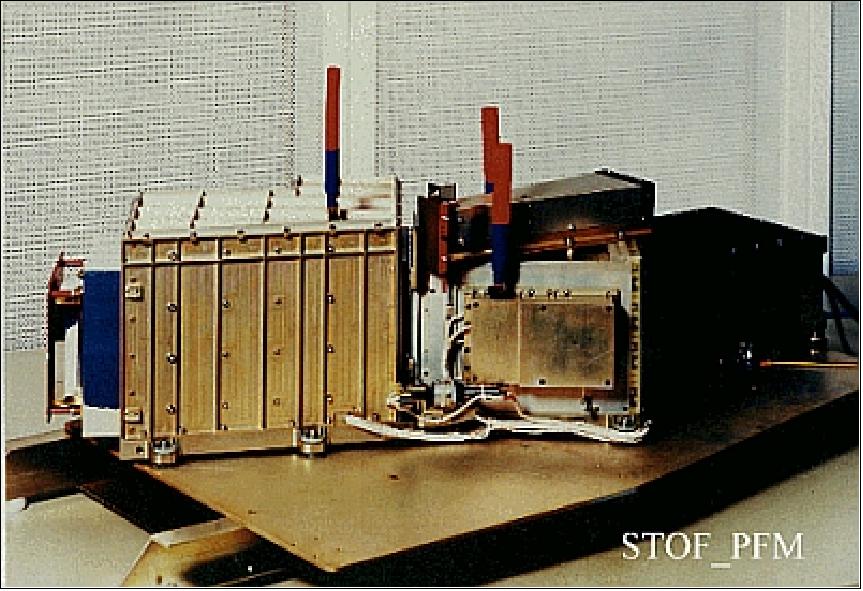

COSTEP (Comprehensive Suprathermal and Energetic Particle Analyzer)
COSTEP PI: H. Kunow, University of Kiel, Germany. The science objectives of COSTEP are related to the study of: a) steady state processes in the solar atmosphere, b) energy release and particle acceleration in the solar atmosphere, c) samples of solar atmospheric material, and d) interplanetary medium. COSTEP consists of two instrument units: EPHIN and LION. 103) 104)
• EPHIN (Electron Proton Helium Instrument), a newly developed telescope for the measurement of energy spectra. The EPHIN sensor is a multi-element array of solid state detectors with anticoincidence to measure energy spectra of electrons in the range 250 keV to > 8.7 MeV, and of hydrogen and helium isotopes in the range 4 MeV/n to > 53 MeV/n.
• LION (Low Energy Ions and Electrons). The LION device is a stack of semiconductor detectors to measure energetic particles. The instrument consists of two sensor heads, each containing a double telescope, which together provide the capability to measure particle spectra in the range 44 keV to 6 MeV for protons and 44 keV to 300 keV for electrons. A channel for Z>1 particles, mainly alphas in the range 7-26 MeV, is also provided. 105)

ERNE (Energetic and Relativistic Nuclei and Electron)
ERNE PI: J. Torsti of the University of Turku, Finland. The objective is to detect charged particles in the solar atmosphere produced in various solar energy release processes. The instrument, ERNE Sensor Unit (ESU), consists of two energetic particle sensors: LED (Low Energy Detector) and HED (High Energy Detector), and an all analog and digital electronics as well as the Data Processing Unit (ESU-DPU) of these sensors are within this unit. 106) 107) 108)
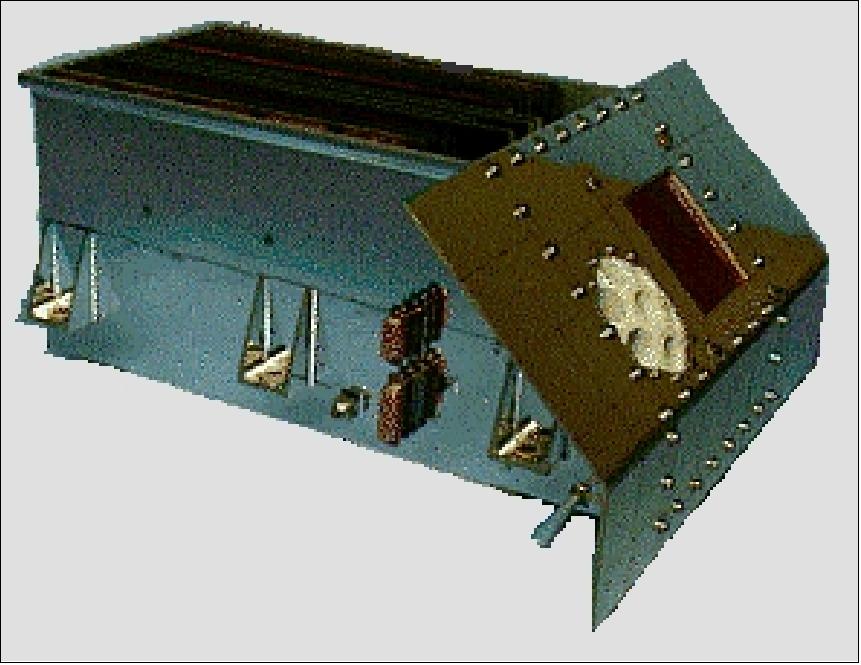
The specific ERNE objects consists of:
• Energy spectra of ions and electrons above 1 MeV/nucleon
• Elemental and isotopic abundance H to Fe
• Particle acceleration in the solar atmosphere and interplanetary shocks
• Particle propagation in the solar corona and in the interplanetary space
• Study both large and small scale properties of the IMF
• Short-period temporal variations of particle flux
• Flux anisotropy within the apparatus viewing cone
• Flux of protons penetrating the instrument (even decay solar neutrons).
ERNE measurements represent the first systematic survey of SEP (Solar Energetic Particle) isotopic abundance, with high sensitivity, high resolution sensors which cover a broad energy range.
The combined COSTEP and the ERNE instruments result in the so-called CEPAC (COSTEP-ERNE Particle Analyzer Collaboration) experiment aboard SOHO. CEPAC address a wide range of scientific topics including:
1) Energy releases and particle acceleration in the solar atmosphere (long duration events, impulsive events, non-flare associated particle events
2) Analysis of samples of solar atmospheric material (large solar particle events, elemental abundances, isotopic abundances, small 3He rich flares, impulsive kilovolt electron events);
3) Steady state processes in the solar atmosphere
4) Particle propagation in the interplanetary medium (travelling shock events, co-rotating interaction regions, small scale plasma events).
GOLF (Global Oscillations at Low Frequencies)
GOLF PI: A. Gabriel, IAS/CNRS, France. Study of the internal structure of the sun by measuring the spectrum of free global oscillations [global velocity and magnetic field oscillations (low degree modes)]. GOLF measures both p and g mode oscillations, with the emphasis on the low order long period waves which penetrate the solar core (frequencies between 10-7 and 6 x 10-2 Hz, with a sensitivity of 1 mm/s). The method involves an extension to space of the ground-based technique for measuring the mean line-of-sight velocity of the viewed solar surface. A sodium vapor resonance scattering filter is used in a longitudinal magnetic field to sample the two wings of the solar absorption line. 109) 110)

Light from the sun passes through a filter which isolates a 17 Å band centered on the Na D lines. It is then brought to a focus by the lens L1 (see Figure 82). Lens L2 serves to produce a near parallel beam to traverse the vapor cell, while at the same time imaging the entrance pupil of the system at the center of the cell. After traversing the cell, the beam is effectively absorbed in a light trap. Light scattered from the vapor in the cell at 90º is collected by two photomultiplier tubes in a symmetrically opposed configuration, operating in photon counting mode. The cell is placed between the poles of a permanent magnet, giving a 5000 gauss longitudinal magnetic field through the sodium vapor. An additional longitudinal field of ± 100 gauss is applied by means of the small coils. The incident light is circularly polarized by a combination of the linear polarizer P1 and the quarter-wave plate QP2. A change of relative orientation of P1 and QP2 by 90º results in a change in the sense of the circularly polarized light transmitted. This change provides the measurement of the intensity ratio in the above equation, which is the direct indicator of the solar velocity vector. The addition of the second quarter-wave plate QP1 facilitates the measurement of the longitudinal component of the average solar magnetic field.
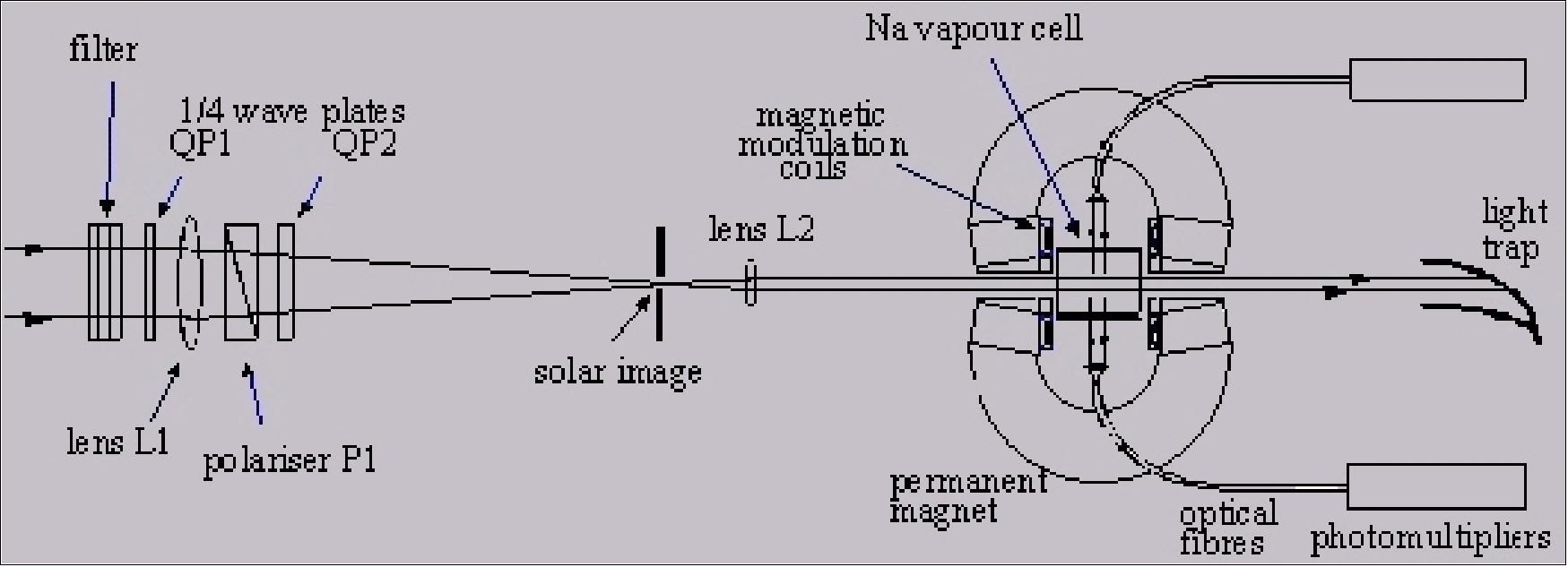
VIRGO (Variability of Solar Irradiance and Gravity Oscillations)
VIRGO PI: C. Froehlich, WRC (World Radiation Center), Davos, Switzerland; ESA/ESTEC. Study of irradiance oscillations (low degree modes) and the solar constant. The total irradiance is measured with active cavity radiometers PMO6-V and DIARAD (Differential Absolute Radiometer), the spectral irradiance by three-channel Sunphotometers (SPM), and the radiance with 12 resolution elements on the solar disk using LOI (Luminosity Oscillations Imager). A scientific objective is probing the solar interior by helioseismology with p- and g-mode solar oscillations determined from spectral irradiance (SPM) and radiance (LOI) variations to the mission time on time scales of minutes. 111) 112) 113) 114)
VIRGO consists of a number of subunits. The optical system of the VIRGO assembly consists of a Ritchey-Chrétien type telescope with a 1300 mm focal length, diameter of 55 mm, and central obstruction 25 mm. Detector: Deep diffused silicon photodiode with 12 scientific and 4 guiding pixels.
• PMO6-V (Physikalisch-Meteorologisches Observatorium, 6th radiometer - VIRGO), built by WRC of Davos, is an active cavity radiometer (absolute instrument) measuring continuously the degradation of the active instrument. Some parameter specifications are: life variation: 30 ppm/year, absolute accuracy: 0.17%, sampling rate: 1 solar total irradiance/2 minutes, duty cycle: 17%, resolution: 50 ppm.
• DIARAD (Differential Absolute Radiometer), built by IRMB, Brussels. An active cavity radiometer. It is composed of two cylindrical cavities coated inside with diffuse black and mounted next to each other on the same heat sink. Some parameter specifications are: lifetime variation: 30 ppm/year, absolute calibration: 0.15%, sampling rate: 1 solar total irradiance/3 minutes, duty cycle: 11%.
• SPM (Sunphotometer), built by WRC. There are two instruments to measure to measure the spectral irradiance at 335, 500, and 865 nm with a bandwidth of 5 nm.
• LOI (Luminosity Oscillations Imager), built by ESA. Measurement of the sun diameter variations with a precision of about 0.3 arcseconds for a 1.5 hour integration time. The LOI wavelength and bandpass is chosen to be the same as the 500 nm channel of SPM. This allows the instruments to be calibrated to each other.
The Total Solar Irradiance (TSI) is being observed with active cavity radiometers (PMO6 and DIARAD) which are traceable to the SI scale of irradiance through direct comparison with cryogenic radiometers on the ground and in vacuum for the PMO6, and thorough characterization in the laboratory for the DIARAD. SSI (Spectral Solar Irradiance) are carried out by SPM (Sunphotometers). - Over the long time period of SOHO mission observations, the VIRGO measurements indicate, that TSI is being increased by 0.1% at solar maximum as compared to the average values at solar minimum periods.

MDI (Michelson Doppler Imager)
MDI PI: P. H. Scherrer, Stanford University, Stanford, CA, USA). The key objective is the Solar Oscillations Investigation (SOI), an international project to study the interior structure and dynamics (velocity oscillations) of the sun's surface. The surface oscillations are an indication of the sun's interior behavior. Measurement of line-of-sight velocity by Doppler shift, transverse velocity by local correlation tracking, line and continuum intensity, and line-of-sight magnetic fields with both 4 and 1.4 arcsec resolution (2 and 0.7 arcsec pixels, respectively).
MDI provides velocity maps by sampling the solar Ni I 6768 Å line profile at four points and finding the Doppler shift by determining the phase of the first Fourier coefficient of the line shape with respect to a reference wavelength. 115) 116) 117)
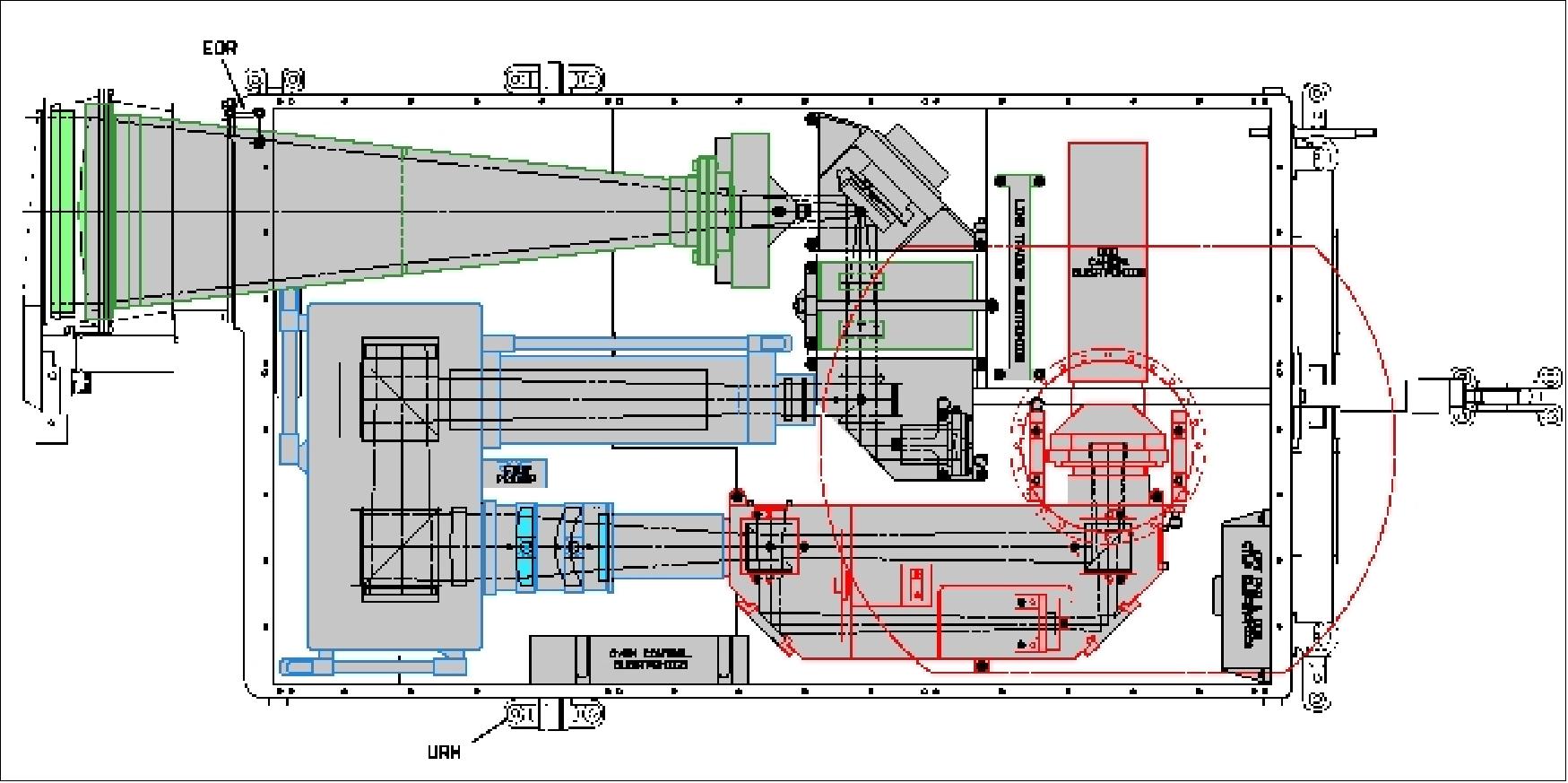
The MDI takes advantage of the Doppler effect, which shifts the sun's light to shorter wavelengths (making it appear more blue) when oscillating material moves toward SOHO and to longer wavelengths (red) when it is moving away. The oscillations on the sun are created by sound waves that bounce around within the sun, making it reverberate like a ringing bell. The effects of the sun's magnetic field are traced by observing it in different colors or wavelengths that reflect different temperatures. The magnetic field at the surface of the sun can be measured by looking at the sun with polarized filters that are tuned to very narrow spectral pass bands (color ranges).

SOHO Data: On-board recording capability of 1 Gbit of data (requirement of 48 h autonomous operation without ground contact)on a tape recorder and 2 Gbit in solid-state memory. Science data transmission via S-band (2.245 GHz). Downlink date rate at 40 kbit/s (160 kbit/s when MDI is in high rate mode).
Achievements of SOHO Observations
Since SOHO began its mission in 1995, the observatory has provided an unparalleled wealth of information about the sun, from its interior, through the hot and dynamic atmosphere, out to the solar wind. Only a few items are listed here. 118) 119) 120)
• Global structure and dynamics of the solar interior: The unprecedented accuracy of helioseismic data from SOHO's MDI, GOLF and VIRGO instruments has enabled substantial improvement in models of the solar interior, and has even shown the importance of considering mixing effects, who in turn solve existing riddles in the isotopic composition of the sun.
• Transition-region dynamics: Several types of transient events have been detected in the quiet sun. Explosive events have been studied using SUMER instrument data.
• Corona: LASCO and EIT are principal instruments. These instruments observed coronal mass ejections (CMEs) that generated subsequent disturbances (the first ever images of the turbulent outer shell of the sun and of the structure below sunspots). These disturbances were observed by other spacecraft to establish the cause-and-effect relationship for a solar system event that extended from the sun to the solar wind to Earth's magnetosphere and ionosphere. EIT has discovered large-scale transient waves (Coronal Moreton Waves) in the corona that propagate outward from the active regions below CMEs.
• Solar wind: The first tracing of the slow-speed solar wind near the equatorial current sheet.
• Solar wind composition: The first detection of elements and isotopes in the solar wind (composition of calcium and nitrogen) observed from data of the CELIAS/MTOF instruments.
• The first image of the convection zone of a star.
• Monitoring the total solar irradiance (the 'solar constant') as well as variations in the extreme ultra violet flux, both of which are important to understand the impact of solar variability on Earth's climate.
• Revolutionizing our ability to forecast space weather, by giving up to three days notice of Earth-directed disturbances, and playing a lead role in the early warning system for space weather.
• One of the most surprising discoveries in the last years that regard the sun is that our star ”sings”. The notes, whose frequencies are too low to be heard by the human ear, cannot propagate in interplanetary space. The solar physics specialists, however, succeed to reveal a rhythmic movement produced at the surface of the sun, generated by the reverberation of the sound waves within. The consequent oscillations have precise frequencies, just like musical notes, and their analysis casts new light on the structure of the sun.
• The SOHO instruments MDI, SWAN, and LASCO have demonstrated the ability to track active regions across the invisible disk in new ways, benefiting forecasters and users of space weather information. SWAN can indirectly monitor the activity on the far side of the sun as it maps the whole sky in ultraviolet light. A huge cloud of interstellar hydrogen that bathes the entire solar system interacts with the solar wind, and lights up in the Lyman-alpha spectral line when it is hit by UV radiation from the sun. Since active regions on the sun are brighter in Lyman-alpha light, the part of the sky facing an active region is brighter.
The scientific achievements of the continuing SOHO mission are the result of a concerted, multi-disciplinary effort by a large international community of solar scientists. The analysis of the SOHO data has revolutionized the way scientists think about the sun and how it might affect the Earth's environment.
As of 2006, more than 2500 papers, representing the work of more than 2300 scientists, have been published based on SOHO data. With SOHO still going strong, the success story is set to continue.
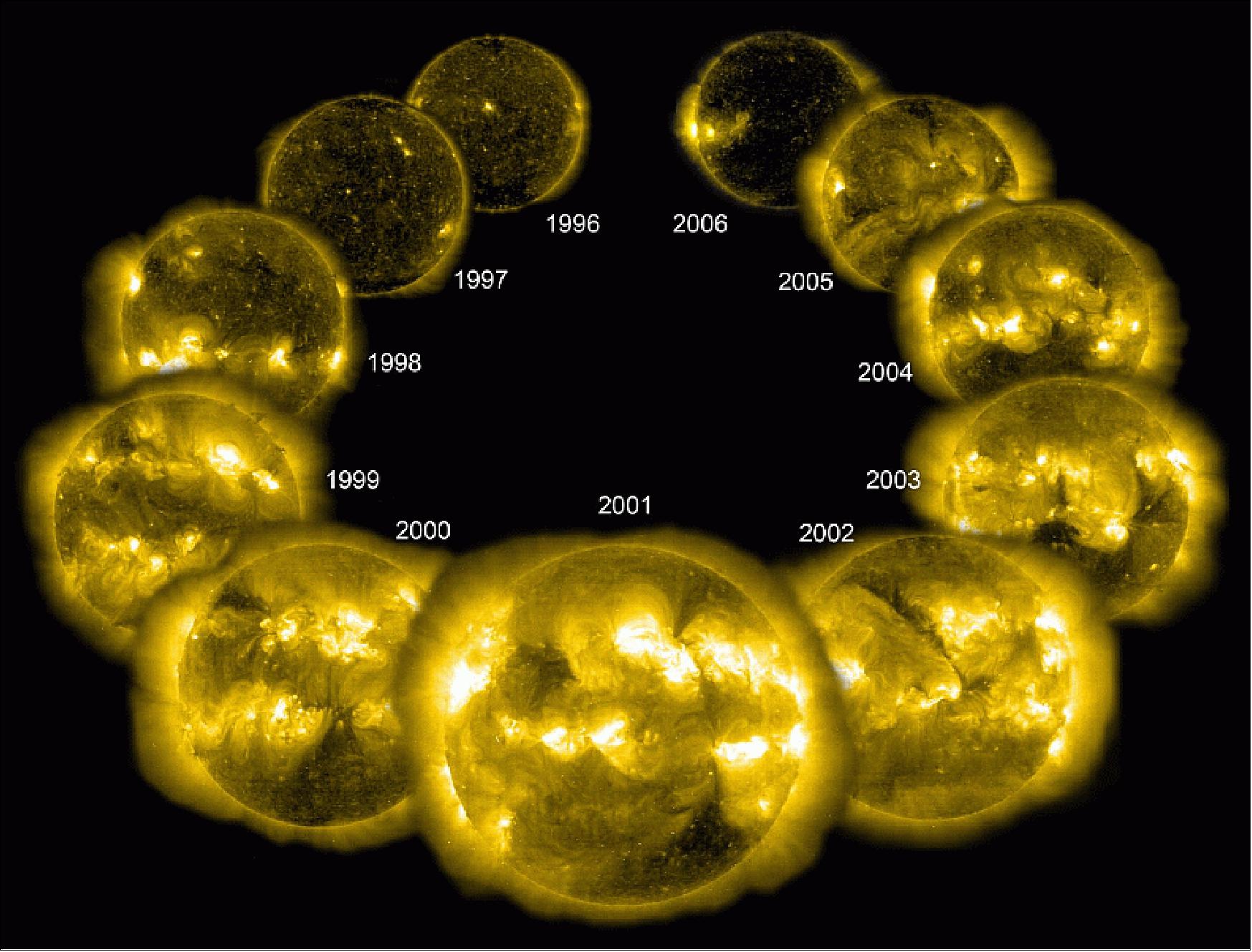
Legend to Figure 86: The image comes from data of the EIT (Extreme UV Imaging Telescope) instrument in the 284 Å wavelength of extreme UV light from each year of nearly an entire solar cycle.
• The image of Figure 86 was released again on March 31, 2014 showing the changing sun, observed by SOHO, over a period of 10 years (nearly an entire solar cycle). From Earth’s surface, we are treated to a biased view. Every day our world is bathed in the Sun’s light and heat, and at these visible and infrared wavelengths our luminary shines to within a fraction of a percent of the same energy every day. 122)
At ultraviolet and X-ray wavelengths, this is not true. Launched in 1995, SOHO has been continuously monitoring the Sun since then, in part to study this variation. Back in 2006, one image for each year of the mission until then was chosen and displayed in this montage (Figure 86). The bright parts of these images correspond to gas in the Sun’s atmosphere at a temperature of about 2 million degrees Celsius.
Unlike visible light, the intensity of the ultraviolet radiation from the sun varies greatly. This variation becomes more pronounced the shorter the wavelength, especially in the X-ray region of the spectrum. This is governed by solar activity, which runs in an approximately 11-year cycle. It is linked to the generation of the Sun’s magnetic field although our precise understanding of this mechanism remains elusive.
The waxing and waning of cycle-23, counted since 1755 when systematic record-taking began, can be seen clearly in this image. At its peak in 2001, the Sun was a maelstrom of activity, releasing about 10 times more ultraviolet light than at the minimum periods that can be seen in 1996 and 2006. - Now in cycle-24 (2014), the Sun is again at a peak of activity, although it is milder than that of 2001.
SOHO the comet hunter
• December 3, 2018: Besides watching the Sun, SOHO has become the most prolific discoverer of comets in astronomical history: as of late 2018, more than 3,400 comets have been found by SOHO, most of them by amateurs accessing SOHO real-time data via the Internet.
• July 30, 2015: In August 2015, SOHO is expected to discover its 3,000th comet. Prior to the SOHO launch, only a dozen or so comets had ever even been discovered from space, and some 900 had been discovered from the ground since 1761. 123)
- "SOHO has a view of about 12 and a half million miles beyond the sun," said Joe Gurman, the mission scientist for SOHO at NASA's Goddard Space Flight Center in Greenbelt, Maryland. "So we expected it might from time to time see a bright comet near the sun. But nobody dreamed we'd approach 200 a year."
- The overwhelming bulk — some 85 percent — of SOHO's comet discoveries are what's called Kreutz comets. Scientists think a single extremely large sungrazing comet broke up thousands of years ago, leading to thousands of leftover fragments, which continue to follow the same Kreutz path. On average, a new member of the Kreutz family is discovered every three days. Unfortunately, the long journey for these fragments invariably ends as they pass the sun. If they're close enough to the sun to be seen by SOHO, they're too close to survive.
- SOHO's great success as a comet finder is, of course, dependent on the people who sift through SOHO's data – a task open to the world as the data is publicly available online in real time. A cadre of volunteer amateur astronomers dedicate themselves to searching the data via the NASA-funded Sungrazer Project. While scientists often search the imagery for very specific events, various members of the astronomy community choose to comb through all the imagery in fine detail. The result: 95 percent of SOHO comets have been found by these citizen scientists.
• August 2005: Besides watching the sun, the instruments on SOHO are very prolific comet hunters - a byproduct of the SOHO information on Internet and the painstaking search by a large community of interested people. The imagery of NRL's LASCO instrument is the most suitable for this purpose. Most of the comets have been found by amateur astronomers coming from all over the world and using the SOHO near-real-time imagery on the Internet. 124)
- On Feb. 4, 2000, SOHO discovered its 100th comet.
- On Aug. 25, 2000, astronomers announced that SOHO had recorded its 200th sun-grazing comet.
- As of May 2003, more than 620 comets had been found by the SOHO spacecraft.
- On June 11, 2004, 800 comets were discovered by SOHO instruments.
- In July 2005, ESA announced that 989 comets have been discovered by SOHO. So far, the SOHO spacecraft has accounted for almost half of all comet discoveries for which orbits have been computed in the history of astronomy.
- On Aug. 5, 2005, SOHO achieved an incredible milestone - the discovery of its 1000th comet! The 1000th comet was a Kreutz-group comet spotted in images from the C3 coronagraph on SOHO's LASCO instrument by Toni Scarmato, from Calabria, Italy.
- Also in 2005, the LASCO imagery revealed for the first time a rare type of comet called a periodic comet (which flies by the sun at regular intervals). While many SOHO comets are believed to be periodic, this is the first one that has been conclusively proven and officially declared as such.
Astronomers have seen thousands of comets but classified only around 190 as periodic. Many more are proposed to be periodic, but they only gain this classification officially if they are seen to follow their orbits around the sun more than twice, and have orbital periods of less than two hundred years. The most famous periodic comet is Halley's comet, returning every 76 years, with its last close pass to the sun taking place in 1986.
SOHO's new catch has a much smaller orbit, taking approximately four years to travel once around the Sun. It was first seen in September 1999, and then again in September 2003. In 2005, German PhD student Sebastian Hoenig realized that the two comets were so similar in orbit that they might actually be the same object. 125)
- About 85% of the SOHO comets discovered so far belong to the Kreutz group of ”sungrazing” comets, so named because their orbits take them very close to the sun. The Kreutz sungrazers come within 800,000 km of the sun's visible surface.
- As of May 2006, over 1100 sun-grazing comets have been discovered from SOHO data.
- As of Oct. 11, 2007 the SOHO comet count reached 1373.
- As of June 25, 2008, the SOHO comet count is 1500. 126)
- In February 2010, the SOHO comet count is 1683. 127)
- On December 26, 2010, the SOHO comet hunt reached 2000. 128)
Comet ISON (C/2012 S1) was discovered on Sept. 21, 2012 by Vitali Nevski and Artyom Novichonok of Russia (using a telescope). C/2012 S1 will come to perihelion (closest approach to the sun) on 28 November 2013 at a distance of 0.012 AU (~1.1 million km above the solar surface), classifying it as a sungrazing comet. There exists a potential for comet ISON to be one of the brightest comets of the past century. NASA foormed a team of experts to coordinate an observing campaign for this comet, under the assumption that it will become easily-visible by the latter part of 2013. 129)

- In September 2015, the SOHO comet hunt is nearing 3000 comets.The U.S. Naval Research Laboratory’s (NRL) sungrazer website lists the discovery count as 2,987 as of July 31, 2015, with more comets awaiting verification daily. 130)
- On Sept. 14, 2015, the 3000th comet was spotted from the data of the SOHO mission by Worachate Boonplod of Samut Songkhram, Thailand (Ref. 42).
References
1) P. Lo Galbo, M. Bouffard, “SOHO - A Cooperative Scientific Mission to the Sun,” ESA Bulletin, Aug. 1992, pp. 21-25
2) “The Solar-Terrestrial Science Project of the Inter-Agency Consultative Group for Space Science,” ESA SP-1107, November 1990, pp. 21-24
3) J. Credland, F. Felici, M. Grensemann, J. A. Steinz, “Three Missions, Three Launches, Six Spacecraft for Science in 1995,” ESA-Bulletin, No. 82, May 1995, pp. 36-47, URL: http://www.esa.int/esapub/bulletin/bullet82/feli82.htm
4) K. R. Lang, “SOHO Reveals the Secrets of the Sun,” Scientific American, in “Magnificent Cosmos,” Vol. 9, No 1, Spring 1998, pp. 50-55
5) http://sohowww.nascom.nasa.gov/
6) SOHO brochure, ESA, URL: https://web.archive.org/web/20181221003443/http://star.mpae.gwdg.de:80/gallery/Slides99_2.pdf
7) SOHO Overview, last update: April 12, 2012, ESA, URL: http://www.esa.int/Our_Activities/Space_Science/SOHO_overview2
8) F. Felici, O. Meert, “The SOHO Project: An International Challenge,” ESA Bulletin No. 84, Nov. 1995,, URL: http://www.esa.int/esapub/bulletin/bullet84/felic84.htm
9) F. C. Vandenbussche, “The SOHO Spacecraft,” ESA Bulletin No. 84, Nov. 1995, pp. 87-91, URL: http://www.esa.int/esapub/bulletin/bullet84/vdbus84.htm
10) F. C. Vandenbussche, P. Temporelli, “SOHO - The Trip to the L1 Halo Orbit ,” ESA Bulletin, No 88, Nov. 1996, URL: http://www.esa.int/esapub/bulletin/bullet88/vande88.htm
11) http://sohowww.nascom.nasa.gov/gif/halo_orbit.gif
12) ”SOHO’s pioneering 25 years in orbit,” ESA Science & Exploration, 2 December 2020, URL: https://www.esa.int/Science_Exploration/Space_Science/SOHO_s_pioneering_25_years_in_orbit
13) ”A method has been developed to study extreme space weather events,” Skoltech, 14 August 2020, URL: https://www.skoltech.ru/en/2020/08/
a-method-has-been-developed-to-study-extreme-space-weather-events/
14) Jenny M. Rodríguez Gómez, Tatiana Podladchikova, Astrid Veronig, Alexander Ruzmaikin, Joan Feynman, and Anatoly Petrukovich, ”Clustering of Fast Coronal Mass Ejections during Solar Cycles 23 and 24 and the Implications for CME–CME Interactions,” The Astrophysical Journal, Volume 899, No 1, Published: 11 August 2020, https://doi.org/10.3847/1538-4357/ab9e72
15) ”Mystery of solar cycle illuminated — In the convection zone of the star, the plasma currents make a huge turnover that lasts about 22 years,” MPS (Max Planck Institute for Solar System Research), 25 June 2020, URL: https://www.mps.mpg.de/6603277/news_publication_15032287_transferred?c=6775
16) Laurent Gizon, Robert H. Cameron, Majid Pourabdian, Zhi-Chao Liang, Damien Fournier, Aaron C. Birch, Chris S. Hanson, ”Meridional flow in the Sun’s convection zone is a single cell in each hemisphere,” Science, Vol. 368, Issue 6498, pp. 1469-1472, Published: 26 June 2020, https://doi.org/10.1126/science.aaz7119
17) ”Citizen scientist discovers Sun-watcher SOHO’s 4000th comet,” ESA Science & Exploration, 17 June 2020, URL: http://www.esa.int/ESA_Multimedia/Images/2020/06/
Citizen_scientist_discovers_Sun-watcher_SOHO_s_4000th_comet
18) ”The discovery of Comet SWAN by solar-watcher SOHO,” ESA / Science & Exploration / Space Science, 13 May 2020, URL: http://www.esa.int/Science_Exploration/Space_Science
/The_discovery_of_Comet_SWAN_by_solar-watcher_SOHO
19) SOHO’s equinox Sun,” ESA, 25 March 2019, URL: http://m.esa.int/spaceinimages/Images/2019/03/SOHO_s_equinox_Sun
20) ”Earth’s atmosphere stretches out to the Moon – and beyond,” ESA, 20 February 2019, URL: http://m.esa.int/Our_Activities/Space_Science/Earth_s_atmosphere_stretches_out_to_the_Moon_and_beyond
21) I. I. Baliukin, J.‐L. Bertaux, E. Quémerais, V. V. Izmodenov, W. Schmidt, ”SWAN/SOHO Lyman‐α mapping: the Hydrogen Geocorona Extends Well Beyond The Moon,” Journal of Geophysical Research: Space Physics, Volume 0, Issue ja, First published: 15 February 2019, https://doi.org/10.1029/2018JA026136
22) ”Earth's atmosphere stretches out to the Moon — and beyond,” NASA, URL: https://sohowww.nascom.nasa.gov/hotshot/index.html/
23) ”Happy 23rd Birthday! (02 Dec 2018),” ESA Pick of the week, 2 December 2018, URL: https://sohowww.nascom.nasa.gov/pickoftheweek/old/02dec2018/
24) ”Extended life for ESA's science missions,” ESA Science & Technology, 14 November 2018, URL: http://sci.esa.int/director-desk/60943-extended-life-for-esas-science-missions/
25) ”ESA rocks space weather,” ESA, 05 November 2018, URL: http://m.esa.int/Our_Activities/Operations/Space_Situational_Awareness/ESA_rocks_space_weather
26) ”22 years of SOHO,” ESA, Space Science Image of the Week, 26 Feb. 2018, URL: http://m.esa.int/spaceinimages/Images/2018/02/22_years_of_SOHO
27) ”Green light for continued operations of ESA science missions,” ESA, 7 Dec. 2017, URL: http://sci.esa.int/director-desk/59839-green-light-for-continued-operations-of-esa-science-missions/
28) Lina Tran, Rob Garner, ”Return of the Comet: 96P Spotted by ESA, NASA Satellites,” NASA/GSFC, 3 November 2017, URL: https://www.nasa.gov/feature/goddard
/2017/return-of-the-comet-96p-spotted-by-esa-nasa-satellites
29) ”Gravity waves detected in Sun's interior reveal rapidly rotating core,” ESA, Aug. 1, 2017, URL: http://www.esa.int/Our_Activities/Space_Science/
Gravity_waves_detected_in_Sun_s_interior_reveal_rapidly_rotating_core
30) Emily Baldwin, Sarah Frazier, ”ESA, NASA’s SOHO Reveals Rapidly Rotating Solar Core,” NASA, Aug. 1, 2017, URL: https://www.nasa.gov/feature/goddard/
2017/esa-nasa-s-soho-reveals-rapidly-rotating-solar-core
31) E. Fossat, P. Boumier, T. Corbard, J. Provost, D. Salabert, F. X. Schmider, A. H. Gabriel, G. Grec, C. Renaud, J. M. Robillot, T. Roca-Cortés, S. Turck-Chièze, R. K. Ulrich, M. Lazrek,”Asymptotic g modes: Evidence for a rapid rotation of the solar core,” Astronomy and Astrophysics, Volume 604, August 2017, Article No A40, DOI: https://doi.org/10.1051/0004-6361/201730460
32) ”SOHO’s summer solstice Sun,” ESA, 26 June 2017, URL: http://www.esa.int/spaceinimages/Images/2017/06/SOHO_s_summer_solstice_Sun
33) ”Impact of a solar storm, 28 October 2003,” ESA, space image of the week, released Dec. 12, 2016, URL: http://www.esa.int/spaceinimages/Images/2016/12/Impact_of_a_solar_storm_28_October_2003
34) ”Two-year extensions confirmed for ESA's science missions,” ESA, Nov. 22, 2016, URL: http://sci.esa.int/director-desk/58589-two-year-extensions-confirmed-for-esa-s-science-missions/
35) Michael Rast, ”ESA’s Report to the 41st COSPAR Meeting,”(ESA SP-1333, June 2016), Istanbul, Turkey, July-August 2016, ”SOHO Mission Status”, pp: 153-154, URL: http://esamultimedia.esa.int/multimedia/publications/SP-1333/SP-1333.pdf
36) K. L. Yeo, N. A. Krivova, S. K. Solanki, K. H. Glassmeier, ”Reconstruction of total and spectral solar irradiance from 1974 to 2013 based on KPVT, SoHO/MDI, and SDO/HMI observations,” Astronomy & Astrophysics, Vol. 570, A85 (2014), DOI: 10.1051/0004-6361/201423628, URL: https://www2.mps.mpg.de/projects/sun-climate/papers/aa23628-14.pdf
37) ”SOHO celebrates 20 years of discoveries,” ESA, Dec. 2, 2015, URL: http://www.esa.int/Our_Activities/Space_Science/SOHO_celebrates_20_years_of_discoveries
38) David Dickinson, ”The Solar Heliospheric Observatory at 20,” Universe Today, Dec. 1, 2015, URL: http://www.universetoday.com/123564/the-solar-heliospheric-observatory-at-20/
39) ”SOHO - two decades of discoveries,” new ESA brochure BR-328, ISBN 978-92-9221-092-2, ISSN 0250-1589, Dec. 1, 2015, URL: http://esamultimedia.esa.int/multimedia/publications/BR-328/offline/download.pdf
40) Emily Baldwin, Bernhard Fleck, Daniel Müller, ”SOHO- Two decades of observing the Sun,” ESA Bulletin No 163, 3rd quarter 2015, pp: 16-23, URL: http://esamultimedia.esa.int
/multimedia/publications/ESA-Bulletin-163/offline/download.pdf
41) ”Spacecraft Discovers Thousands of Doomed Comets,” NASA Science News, Oct. 25, 2015, URL: http://science.nasa.gov/science-news/science-at-nasa/2015/24oct_doomedcomets/
42) Rob Garner, “ESA/NASA Solar Observatory Discovers Its 3,000th Comet,” NASA, Sept. 15, 2015, URL: http://www.nasa.gov/feature/goddard/esa-nasa-solar-observatory-discovers-its-3000th-comet
43) “SOHO's 3000th Comet,” ESA, Sept. 16, 2015, URL: http://www.esa.int/spaceinimages/Images/2015/09/SOHO_s_3000th_comet
44) “20 SOHO views of the Sun,” ESA, July 13, 2015, URL: http://www.esa.int/spaceinimages/Images/2015/07/20_SOHO_views_of_the_Sun
45) “Protecting Earth from space weather,” ESA, March 19, 2015: URL: http://www.esa.int/Our_Activities/Preparing_for_the_Future
/Space_for_Earth/Energy/Protecting_Earth_from_space_weather
46) “SOHO Comet 2875 (February 20, 2015),” NASA, URL: http://sohowww.nascom.nasa.gov/pickoftheweek/
47) Bob King, “A New Sungrazing Comet May Brighten in the Evening Sky,” Universe Today, Feb. 24, 2015, URL: http://www.universetoday.com/119104/
a-new-sungrazing-comet-may-brighten-in-the-evening-sky-heres-how-to-see-it/
48) “Comet ISON disintegrates,” ESA, 'Space Science Image of the Week', Dec. 01, 2014: URL: http://www.esa.int/spaceinimages/Images/2014/12/Comet_ISON_disintegrates
49) “Working life extensions for ESA's science missions,” ESA, Nov. 20, 2014, URL: http://sci.esa.int
/director-desk/54999-working-life-extensions-for-esas-science-missions/
50) “Giant solar flare,” ESA/NASA, Released on Sept. 15, 2014 (Space science image of the week), URL: http://www.esa.int/spaceinimages/Images/2014/11/Giant_solar_flare
51) Michael Weaver, William Murtagh, Christopher Balch, Doug Biesecker, Larry Combs, Misty Crown, Kent Doggett, Joseph Kunches, Howard Singer, LT David Zezula, “Halloween Space Weather Storms of 2003,” NOAA Technical Memorandum OAR SEC-88, June 2004, URL: http://www.swpc.noaa.gov/Services/HalloweenStorms_assessment.pdf
52) J. U. Kozyra,M. W. Liemohn, C. Cattell, D. De Zeeuw, C. P. Escoubet, D. S. Evans, X. Fang, M.-C. Fok, H. U. Frey, W. D. Gonzalez, M. Hairston, R. Heelis, G. Lu, W. B. Manchester IV, S. Mende, L. J. Paxton, L. Rastaetter, A. Ridley, M. Sandanger, F. Soraas, T. Sotirelis, M. W. Thomsen, B. T. Tsurutani, O. Verkhoglyadova, “Solar filament impact on 21 January 2005: Geospace consequences,” Journal of Geophysical Research: Space Physics, Volume 119, Issue 7, pp: 5401–5448, July 2014
53) “A mixed-up magnetic storm.” ESA, August 28, 2014, URL: http://sci.esa.int
/cluster/54573-a-mixed-up-magnetic-storm/
54) “Necklaces of solar activity,” ESA & NASA Space Science Image of the week , released on June 16, 2014, URL: http://www.esa.int/spaceinimages/Images/2014/06/Necklaces_of_solar_activity
55) “ESA science missions continue in overtime,” ESA, June 20, 2013, URL: http://sci.esa.int
/director-desk/51944-esa-science-missions-continue-in-overtime/
56) “SOHO Captures Jan. 14, 2014 CME,” NASA, Jan. 14, 2014, URL: http://www.nasa.gov/content/goddard/soho-captures-jan-14-2014-cme/#.Uz0sNaKegkA
57) “The abstract science of the dynamic Sun,” ESA, January 13, 2014, URL: http://www.esa.int/spaceinimages/Images/2014/01/The_abstract_science_of_the_dynamic_Sun
58) “Our Stormy Sun,” ESA, Oct. 31, 2013, URL: http://spaceinimages.esa.int/Images/2013/10/Our_stormy_Sun
59) Karen C. Fox, “Large Coronal Hole Near the Sun’s North Pole,” NASA, July 19, 2013, URL: http://www.nasa.gov/content/goddard/large-coronal-hole-near-sun-north-pole/#.UfIsvKzODWI
60) “Large Coronal Holes,” ESA, July 19, 2013, URL: https://web.archive.org/web/20151222230934/http://soho.esac.esa.int/pickoftheweek/
61) “ESA Science Missions continue in overtime,” ESA, June 20, 2013, URL: http://sci.esa.int
/director-s-desk/51944-esa-science-missions-continue-in-overtime/
62) Nany Atkinson, “The Sun Burps Out a Gigantic Rolling Wave,” Universe Today, May 1, 2013, URL: http://www.universetoday.com/101894/the-sun-burps-out-a-gigantic-rolling-wave/
63) SOHO - Science and Technology,” ESA, Feb. 24, 2013, URL: http://planck.esa.int
/science-e/www/area/index.cfm?fareaid=14&secured=-1
64) http://sohowww.estec.esa.nl/
65) “Programmes in Progress: SOHO, Status end of July 2011, “ ESA Bulletin No. 147, August, 2011, p. 66
66) http://sci.esa.int/science-e/www/area/index.cfm?fareaid=14
67) “Solar Observation Mission Celebrates 15 Years,” Space Daily, Dec. 6, 2010, URL: http://www.spacedaily.com/reports/Solar_Observation_Mission_Celebrates_15_Years_999.html
68) “Europe maintains its presence on the final frontier,” Nov. 22, 2010, URL: http://www.esa.int/esaSC/SEMR1MIRPGG_index_0.html
69) “A change of pace for EIT, the ground-breaking Sun-watching camera,” ESA, Sept. 21, 2010, URL: http://sci.esa.int/science-e/www/object/index.cfm?fobjectid=47703
70) “Mission extensions approved for science missions,” ESA, Oct. 7, 2009, URL: http://sci.esa.int
/science-e/www/object/index.cfm?fobjectid=45685
71) http://sci.esa.int/science-e/www/object/index.cfm?
fobjectid=31525&farchive_objecttypeid=30&farchive_objectid=30930&fareaid_2=63
72) “ESA's new SOHO science archive now online,” ESA, Nov. 23, 2009, URL: http://sci.esa.int
/science-e/www/object/index.cfm?fobjectid=45900
73) “SOHO - keeping an eye on the Sun for 12 years,” Dec. 4, 2007, URL: http://www.esa.int/esaSC/SEMSMHSVQ9F_index_0.html
74) http://sci.esa.int/science-e/www/object/index.cfm?fobjectid=39292
75) Bernhard Fleck, Daniel Müller, Stein Haugan, Luis Sánchez Duarte, Tero Siili, Joseph B. Gurman, “10 Years of SOHO,” ESA Bulletin No 126, May 2006, pp. 24-32, URL: https://web.archive.org/web/20150330162549/http://soho.esac.esa.int/publications/ESA_Bull126.pdf
76) “10 Years of SOHO,” Dec. 2, 2005, URL: http://sci.esa.int/science-e/www/object/index.cfm?fobjectid=38381
77) F. C. Vandenbussche, “SOHO's Recovery - An Unprecedented Success Story,” ESA Bulletin No 97, March 1999, URL: http://www.esa.int/esapub/bulletin/bullet97/vandenbu.pdf
78) Craig E. Roberts, “The SOHO Mission L1 Halo Orbit Recovery From the Attitude Control Anomalies of 1998,” Libration Point Orbits and Applications Conference, Parador d’Aiguablava, Girona, Spain, June 10-14, 2002, URL: http://www.ieec.fcr.es/libpoint/papers/roberts.pdf
79) “The SOHO Recovery,” URL: http://sohowww.nascom.nasa.gov/about/Recovery/
80) Ton van Overbeek, Jean-Philippe Olive, “SOHO, 13 years since launch and still going strong,” Proceedings of the 32nd AAS Guidance and Control Conference, Breckenridge, CO, USA, Jan. 31.- Feb. 4, 2009, AAS 09-032
81) http://www.esa.int/esaSC/SEMJFH374OD_0_spk.html
82) The SOHO Mission - Scientific and Technical Aspects of the Instruments, ESA SP-1104, ISSN 0379-6566, Nov. 1988
83) http://sohowww.nascom.nasa.gov/about/instruments.html
84) http://www.mps.mpg.de/projects/soho/sumer/
85) R. A. Harrison, E. C. Sawyer, M. K. Carter, A. M. Cruise, R. M. Cutler, A. Fludra, R. W. Hayes, B. J. Kent, J. Lang, D. J. Parker, J. Payne, C. D. Pike, S. C. Peskett, A. G. Richards, J. L. Culhane, et al., “The Coronal Diagnostic Spectrometer for the Solar and Heliospheric Observatory,” Solar Physics, Vol. 162, No 1-2, Dec. 1995, pp. 233-290
86) K. A. Lidiard, P. F. Gray, “Optical design of the coronal diagnostic spectrometer (an instrument on the Solar and Heliospheric Observatory),” Optical Engineering, Vol. 36, No. 8, Aug. 1997, pp. 2311-2319
87) R. A. Harrison, E. C. Sawyer, M. K. Carter, A. M. Cruise, R. M. Cutler, A. Fludra, et al., “3 The Coronal Diagnostic Spectrometer for the Solar and Heliospheric Observatory,” Solar Physics, Vol. 162, pp. 233-290
88) http://solar.bnsc.rl.ac.uk/
89) C. Foley, “Coronal Diagnostic Spectrometer,” Aug. 2003, URL: http://www.mssl.ucl.ac.uk/www_solar/soho/cds_swnote_54.pdf
90) R. Harrison, “Looking for the CME Onset: A 10 Year CDS Campaign,” 2005, URL: http://solar.bnsc.rl.ac.uk/newsletter/issue13/Richard_Harrison_CME.ppt
91) Eric Sawyer, Jim Long, “A review of the mechanisms on SOHO/CDS after five years in orbit,” Proceedings of the 9th European Space Mechanisms and Tribology Symposium, Sept. 19-21, 2001, Liège, Belgium, URL: http://www.esmats.eu/esmatspapers/pastpapers/pdfs/2001/sawyer.pdf
92) J. P. Delaboudiniere, G. E. Artzner, J. Brunaud, A. H. Gabriel, B. Au, K. P. Dere, R. A. Howard, R. Kreplin, D. J. Michels, J. D. Moses, J. M. Defise, C. Jamar, P. Rochus, J. P. Chauvineau, J. P. Marioge, R. C. Catura, J. R. Lemen, L. Shing, R. A. Stern, J. B. Gurman, W. M. Neupert, A. Maucherat, F. Clette, P. Cugnon, E. L. van Dessel, “EIT: Extreme-Ultraviolet Imaging Telescope for the SOHO Mission,” Solar Physics, Vol. 162, 1995, pp. 291-312
93) J. L. Kohl, R. Esser, L. D. Gardner, S. Habbal, P. S. Daigneau, E. F. Dennis, G. U. Nystrom, .A. Panasyuk, J. C. Raymond, P. L. Smith, L. Strachan, A. A. van Ballegooijen, G. Noci, S. Fineschi, et al. “The Ultraviolet Coronagraph Spectrometer for the Solar and Heliospheric Observatory,” Solar Physics, Vol.. 162, 1995, pp. 313-356
94) http://www.cfa.harvard.edu/uvcs/
95) J. W. Cook, “LASCO Handbook for Scientific Investigations,” Version 1.0, 1994
97) “The SWAN Instrument on board SOHO - Solar Wind Anisotropies,” URL: https://web.archive.org/web/20020604192057/http://www.aerov.jussieu.fr/projet/SWAN/
98) J. L. Bertaux, E. Kyrölä, E. Quémerais, R. Pellinen, R. Lallement, W. Schmidt, M. Berthé, E. Dimarellis, J. P. Goutail, C. Taulemesse, C. Bernard, G. Leppelmeier, T. Summanen, H. Hannula, H. Huomo, V. Kehlä, S. Korpela, K. Leppälä, E. Strömmer, J. Torsti, K. Viherkanto, J. F. Hochedez, G. Chretiennot, R. Peyroux, T. Holzer, “SWAN: A study of Solar Wind Anisotropies on SOHO with Lyman alpha sky mapping,” Solar Physics, Vol. 162, No 1 & 2, Dec. 1995, pp. 403-439,
99) D. Hovestadt, M. Hilchenbach, A. Bürgi, B. Klecker, P. Laeverenz, M. Scholer, H. Grünwaldt, W. I. Axford, S. Livi, E. Marsch, B. Wilken, H. P. Winterhoff, F. M. Ipavich, P. Bedini, M. A. Coplan, A. B. Galvin, G. Gloeckler, P. Bochsler, H. Balsiger, J. Fischer, J. Geiss, R. Kallenbach, P. Wurz, K. -U. Reiche, F. Gliem, D. L. Judge, H. S. Ogawa, K. C. Hsieh, E. Möbius, M. A. Lee, G. G. Managadze, M. I. Verigin, M. Neugebauer, “CELIAS - Charge, Element and Isotope Analysis System for SOHO,” Solar Physics, Vol. 162, No 1-2, December 1995, pp. 441-481
100) D. L. Judge, D. R. McMullin, H. S. Ogawa, D. Hovestadt, B. Klecker, M. Hilchenbach, E. Möbius, L. R. Canfield, R. E. Vest, R. Watts, C. Tarrio, M. Kühne, P. Wurz, “First Solar EUV Irradiances Obtained from SOHO by the Celias/Sem,” Solar Physics, Vol. 177, No 1 & 2, pp. 161-173
101) http://www.space.unibe.ch/soho/
102) http://www.space.unibe.ch/soho/gallery.html#Contents
103) http://www.ieap.uni-kiel.de/et/ag-heber/costep/
104) R. Müller-Mellin, H. Kunow, V. Fleißner, E. Pehlke, E. Rode, N. Röschmann, C. Scharmberg, H. Sierks, P. Rusznyak, S. Mckenna-Lawlor, I. Elendt, J. Sequeiros, D. Meziat, S. Sanchez, J. Medina, L. del Peral, M. Witte, R. Marsden, J. Henrion, “COSTEP - Comprehensive Suprathermal and Energetic Particle Analyzer,” Solar Physics, Vol. 162, No 1 & 2, 1995, pp. 483-504
105) S. M. P. McKenna-Lawlor, I. Elendt, P. Rusznyak, H. Kunow, R. Müller-Mellin, M. Witte, “The LION instrument on SOHO and its scientific objectives,” Annales Geophysicae, Vol. 15, 1997, pp. 1-4
106) J. Torsti, E. Valtonen, M. Lumme, P. Peltonen, T. Eronen, M. Louhola, E. Riihonen, G. Schultz, M. Teittinen, K. Ahola, C. Holmlund, V. Kelhä, K. Leppälä, P. Ruuska, E. Strömmer, “Energetic Particle Experiment ERNE,” Solar Physics, Vol. 162, No 1 & 2, 1995, pp. 505-531
107) J. E. Torsti, T. Valtonen, E. Eronen, M. Hoisko, M. Louhala, M. Lumme, A. Niemenen, P. Peltonen, E. Rahonen, G. Schultz, M. Teitennen, “Energetic particle analyzer ERNE,” Proceedings of First SOHO Workshop, Annapolis, MD, USA, Aug. 25-28, 1992, ESA SP 348, pp. 47-50, 1992.
108) http://www.srl.utu.fi/projects/erne/index_english.html
109) A. H. Gabriel, G. Grec, J. Charra, J.-M. Robillot, T. Roca Cortés, S. Turck-Chièze, R.Bocchia, P. Boumier, M.Cantin, E. Cespédes, B. Cougrand, J. Crétolle, L. Damé, M. Decaudin, P. Delache, N. Denis, R. Duc, H. Dzitko, E. Fossat, J.-J. Fourmond, R. A. García, D. Gough, C. Grivel, J. M. Herreros, H. Lagardère, J.-P. Moalic, P. L. Pallé, N. Pétrou, M. Sanchez, R. K. Ulrich, H. B. Van der Raay, “Global oscillations at low frequencies for the SOHO mission (GOLF),” Solar Physics, Vol. 162, 1995, p. 61-99
110) http://golfwww.medoc-ias.u-psud.fr/
111) C. Froehlich, J. Romero, H. Roth, C. Wehrli, B. N. Andersen, T. Appourchaux, V. Domingo, U. Telljohann, G. Berthomieu, Ph. Delache, J. Provost, T. Toutain, D. Crommelynck, A. Chevalier, A. Fichot, W. Däppen, D. O. Gough, T. Hoeksema, A. Jiménez, M.F. Gómez, J. M. Herreros, T. Roca Cortés, A. Jones, J. Papp & R. Willson., “VIRGO: Experiment for Helioseismology and Solar Irradiance Monitoring,” Solar Physics, Vol. 162, 1995, pp. 101-128
112) http://www.ias.u-psud.fr/virgo/
113) T. Appourchaux, U. Telljohann, D. Martin, J. Fleur, S. Leveque, “The Luminosity Oscillations Imager on Board VIRGO: Design and Performances,” Proceedings of 4th SOHO Workshop: Helioseismology, Pacific Grove, CA; April 2-6, 1995; ESA SP-376 Vol. 2, pp. 359-363, 1995
114) S. Dewitte, D. Crommelynck, A. Joukoff, “Total solar irradiance observations from DIARAD/VIRGO,” Journal of Geophysical Research, Vol. 109, A02102, doi:10.1029/2002JA009694, Feb. 5, 2004
115) P. H. Scherrer, R. S. Bogart, R. I. Bush, J. T. Hoeksema, A. G. Kosovichev, J. Schou, W. Rosenberg, L. Springer, T. D. Tarbell, A. Title, C. J. Wolfson, I. Zayer, and the MDI Engineering Team, ”The Solar Oscillations Investigation - Michelson Doppler Imager,” Solar Physics, Vol. 162, 1995, pp. 129-188
117) I. Zayer, M. Morrison, T. Pope, W. Rosenberg, T. Tarbell, A. Title, J. Wolfson, R. S. Bogart, J. T. Hoeksema, P. Milford, P. H. Scherrer, J. Schou, “Michelson Doppler Imager (MDI) Performance Characteristics,” Astronomical Society of the Pacific Conference Series, Volume 76. GONG '94: Helio- and Astero-Seismology from the Earth and Space, Los Angeles, May 16-20, 1994
118) B. Fleck, D. Müller, S. Haugan, L. Sanchez Duarte, T. Siili, Joseph B. Gurman, “10 Years of SOHO,” ESA Bulletin No 126, May 2006, pp. 24-32, URL: http://www.esa.int/esapub/bulletin/bulletin126/bul126d_fleck.pdf
119) B. Fleck, P. Brekke, S. Haugan, L. Sanchez Duarte, V. Domingo, J. B. Gurman, A. I. Poland, “Four Years of SOHO Discoveries - Some Highlights,” ESA Bulletin, 102, May 2000, pp. 69-86, URL: http://www.esa.int/esapub/bulletin/bullet102/Fleck102.pdf
120) K. R. Lang, “SOHO Reveals the Secrets of the Sun,” Scientific American, March 1997, pp. 40-47
121) “Astronomy Picture of the Day,” NASA, ESA, Dec. 7, 2007, URL: http://antwrp.gsfc.nasa.gov/apod/ap071203.html
122) “Solar cycle,” ESA, March 31, 2014, URL: http://www.esa.int/spaceinimages/Images/2014/03/Solar_cycle
123) Karen C. Fox, ”Nearing 3,000 Comets: SOHO Solar Observatory Greatest Comet Hunter of All Time,” NASA, 30 July, 2015, URL: https://www.nasa.gov/feature/
goddard/soho/solar-observatory-greatest-comet-hunter-of-all-time
124) “History's greatest comet hunter discovers 1000th comet,” Aug.19, 2005, http://www.esa.int/esaCP/SEMCA3908BE_index_0.html
125) http://www.astronomy.com/asy/default.aspx?c=a&id=6046
126) “SOHO discovers its 1500th comet,” ESA June 27, 2008, URL: http://www.esa.int/esaSC/SEMB94SHKHF_index_0.html
127) http://www.comethunter.de/
128) “SOHO Spots 2000th Comet,” NASA, Dec. 28, 2010, URL: http://www.nasa.gov/mission_pages/soho/comet-2000.html
129) “The NASA Comet ISON Observing Campaign,” NASA, URL: http://sungrazer.nrl.navy.mil
/index.php?p=ison
130) David Dickinson, “SOHO Nears 3,000 Comet Discoveries,” Universe Today, Sept. 10, 2015, URL: http://www.universetoday.com/122277/soho-nears-3000-comet-discoveries/
The information compiled and edited in this article was provided by Herbert J. Kramer from his documentation of: ”Observation of the Earth and Its Environment: Survey of Missions and Sensors” (Springer Verlag) as well as many other sources after the publication of the 4th edition in 2002. - Comments and corrections to this article are always welcome for further updates (eoportal@symbios.space).
Spacecraft Launch Mission Status Sensor Complement Mission Achievements SOHO - Comet hunter References Back to Top