TSX (TerraSAR-X)
EO
Atmosphere
Ocean
Atmospheric Temperature Fields
TerraSAR-X (TSX) is an Earth observation satellite mission. It provides high-resolution X-band data for use in climatology, environmental and disaster monitoring, hydrology, geology, oceanography and cartography.
Quick facts
Overview
Mission type | EO |
Agency | DLR |
Mission status | Operational (extended) |
Launch date | 15 Jun 2007 |
Measurement domain | Atmosphere, Ocean, Land, Snow & Ice |
Measurement category | Atmospheric Temperature Fields, Multi-purpose imagery (ocean), Multi-purpose imagery (land), Vegetation, Albedo and reflectance, Atmospheric Humidity Fields, Landscape topography, Ocean topography/currents, Sea ice cover, edge and thickness, Snow cover, edge and depth |
Measurement detailed | Ocean imagery and water leaving spectral radiance, Land surface imagery, Vegetation type, Earth surface albedo, Atmospheric specific humidity (column/profile), Atmospheric temperature (column/profile), Ocean surface currents (vector), Land surface topography, Sea-ice cover, Snow cover, Sea-ice type, Glacier motion, Glacier cover |
Instruments | X-Band SAR, GPSRO (Terra-SAR) |
Instrument type | Imaging microwave radars, Atmospheric temperature and humidity sounders |
CEOS EO Handbook | See TSX (TerraSAR-X) summary |
Related Resources
Summary
Mission Capabilities
The TSX SAR instrument is an active phase array X-band antenna system that provides multispectral SAR imagery in numerous operational modes such as ScanSar, Spotlight and Stripmap. Each distinct mode of TSX SAR functions as a radar system that builds reconstructions of desired landscapes for Earth observation purposes.
TSX also includes a Tracking, Occultation and Ranging payload (TOR), which functions as a dual-frequency GPS receiver IGOR (Integrated GPS Occultation Receiver) that collects atmospheric radio occultation (RO) data to be used in the improvement of weather forecasting, climate change studies and space weather monitoring. The IGOR GPS receiver also has tracking capabilities that allow for precise orbit determination (POD) and works in conjunction with the Laser Retro Reflector (LRR) to evaluate GPS-based orbital data independently.
Additionally, the TOR payload involves support modes that are able to provide processing of additional GPS occultation missions, including TOR-SAR, which focuses on precise science orbit (PSO) and SAR instrumentation, TOR-RO, which is responsible for ultra-rapid science orbit (USO), atmospheric excess phases, occultation tables, and ionospheric profiles, and TOR-POD, which focuses on PSO and USO.
TSX also has a communication function which is built into the Laser Communication Terminal (LCT) that provides bidirectional communications for binary digital data transfer between satellites in different orbits (lower Earth orbit to mid-Earth orbit) or between a satellite and its constituent ground segment.
Performance Specifications
TSX-SAR has numerous imaging modes that allow for superior quality when compared to predecessor technology. Spotlight (SL) and Spotlight High Resolution (HS) are based on a phased array beam that steers toward an azimuth direction for an overall increase in illumination. The image size for SL is restricted to 10km x 10km at a spectral resolution of 2m, whilst HS mode has an image size of 5-10km x 5km at 1m spectral resolution. There is also an experimental mode which produces a coverage of 5 x 10 and a spatial resolution of 1m.
Alternative modes include the StripMap mode (SM) which produces superior spatial resolutions of 3m with an image size of ≤1500km x 30km, and ScanSAR (SC) mode which provides 16m spatial resolution with a standard image size of ≤1500km x 100 km. TSX has a revisit period of 11 days, equating to 167 orbits within a revisit period or roughly 15 orbits per day.
Space and Hardware Components
The TSX bus has a total height of about 5m with a diameter of roughly 2.4m and includes the SAR instrument, the solar array and the DNepr-1 launcher fairing (for payload protection). The SAR antenna is mounted upon the hexagonal side of the spacecraft, which in flight points toward 33.8° off the nadir.
The Laser Retro Reflector (LRR) is maintained by a global network of 40 ground stations of the International Laser Ranging Service (ILRS) and functions as an optical device for accurate satellite tracking.
The DLR bases are composed of DLR/GSOC (Communication and Ground Station) which provide spacecraft operations support, DLR/HR (Microwaves and Radar Institute) that functions as an Instrument Operation and Calibrations Segment (IOCS), Payload Ground Segment (PGS) which involves DLR data processing, analysis and simulation, and a DLR Ground Segment that provides main functions of space and ground segment planning, orbital control, telemetry, command and product generation of user services.
TSX (TerraSAR-X) MissionSpacecraft Launch Mission Status Sensor Complement Secondary Payloads Ground Segment References
TerraSAR-X1 (also referred to as TSX or TSX-1) is a German SAR satellite mission for scientific and commercial applications (national project). The project is supported by BMBF (German Ministry of Education and Science) and managed by DLR (German Aerospace Center). In 2002, EADS Astrium GmbH was awarded a contract to implement the X-band TerraSAR satellite (TerraSAR-X) on the basis of a public-private partnership agreement (PPP). In this arrangement, EADS Astrium funded part of the implementation cost of the TerraSAR-X system.
In exchange, EADS Astrium/Infoterra received exclusive commercial exploitation rights for the TerraSAR-X data. The satellite is owned and operated by DLR, and the scientific data rights remain with DLR. The satellite has a design life of at least five years. TerraSAR-X is of SIR-C/X-SAR (1994) and SRTM (2000) heritage - DLR SAR instruments flown on Shuttle missions. 1) 2) 3) 4) 5) 6) 7) 8) 9) 10) 11)
The science objectives are to make multi-mode and high-resolution X-band data available for a wide spectrum of scientific applications in such fields as:
- hydrology,
- geology,
- climatology,
- oceanography,
- environmental and disaster monitoring,
- cartography (DEM generation) making use of interferometry and stereometry.
The science potential of the mission is given by:
- The high geometric and radiometric resolution (experimental 300 MHz Mode for very high range resolution)
- The single, dual and quad polarization mode capability
- The capability of multi-temporal imaging
- The capability of repeat-pass interferometry
- The capability of ATI (Along-Track Interferometry)
The business goal in this venture is to establish a commercial EO (Earth Observation) market by Infoterra on a sustainable service concept to its customer base. Infoterra, a subsidiary of EADS Astrium, is comprised of Infoterra Ltd. in Farnborough, UK, and Infoterra GmbH in Friedrichshafen, Germany (a subsidiary of EADS Astrium GmbH). Infoterra has established a global distribution network with a range of service options for its customers.
A commercial goal is also to provide monitoring services for the European initiative GMES (Global Monitoring for Environment and Security). 12) 13) 14)


Spacecraft
The TerraSAR X-band satellite, built by Airbus Defence and Space Geo-Intelligence/Infoterra GmbH (formerly EADS Astrium GmbH, Friedrichshafen, Germany) employs a mission-tailored AstroSat-1000 bus (successor of Flexbus and LEOSTAR due to industrial merger - initially AstroSat-1000 was referred to as AstroBus) concept with a heritage of CHAMP and GRACE missions.
The hexagonal outer shape of the spacecraft, with a total height of about 5 m and a diameter of about 2.4 m, is mainly driven by the accommodation of the SAR instrument, the body-mounted solar array, and the geometrical limitations given by the Dnepr-1 launcher fairing.
The S/C bus design features a central hexagonal CFRP structure as the main load-carrying element. The cross-sectional view of Figure 6 illustrates the mounting concept of the radiator, solar array, and SAR antenna elements. Three sides of the hexagon are populated with electronic equipment, while the sun-facing side is additionally carrying the solar array.
The SAR antenna is mounted on one of the hexagon sides, which in flight attitude points 33.8º off the nadir. The other nadir-looking side is reserved for the accommodation of an S-band TT&C antenna, a SAR data downlink antenna - carried by a deployable boom of 3.3 m length in order to avoid RF interferences during simultaneous radar imaging and data transmission to the ground - and a Laser Retro Reflector to support precise orbit determination. The deep-space-looking surface is used for the LCT (Laser Communication Terminal) and as a thermal radiator. The total wet mass of the satellite is about 1230 kg.
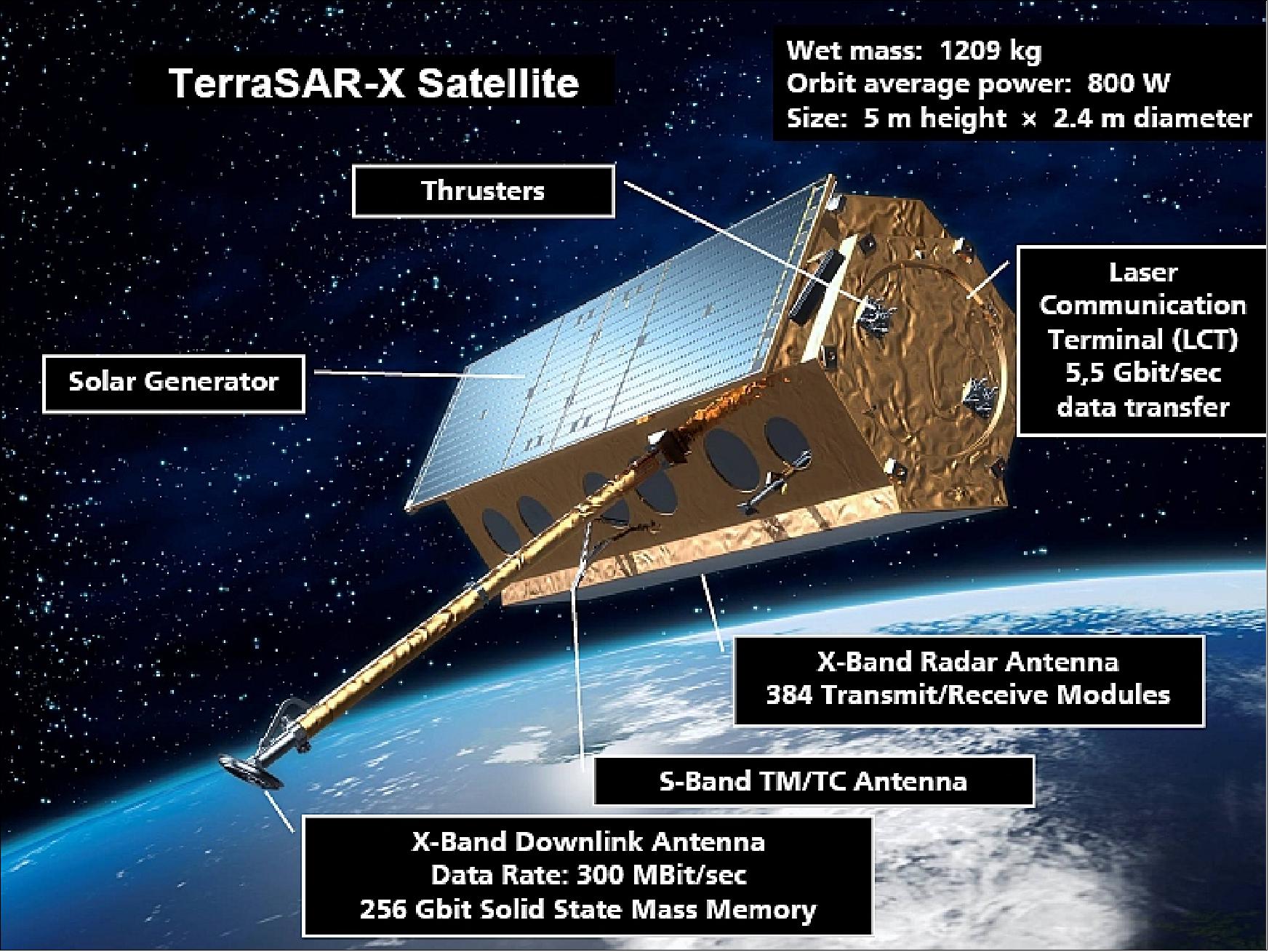
The solar array is of size 5.25 m2, and triple-junction GaAs type solar cells provide an average orbital power of 800 W EOL. The attitude control system is based on reaction wheels for fine-pointing, with magnetorquers for desaturation, and a propulsion system also capable of attitude control in order to achieve rapid rate damping during initial acquisition.
Attitude measurement is performed with a GPS/Star Tracker system (MosaicGNSS) during nominal operation and a CESS (Coarse Earth and Sun Sensor) in safe mode situations, initial acquisition respectively (CESS is of CHAMP and GRACE heritage). A combination of an IMU (Inertial Measurement Unit) and a magnetometer serve to support rate measurements in all mission phases. In fine pointing mode, a pointing accuracy of 65 arcsecs is achieved (3 σ). Nominal attitude control follows a novel “total zero Doppler steering” law developed by DLR.
Precise orbit determination is performed with a dual-frequency GPS receiver and raw data post-processing on ground, permitting orbit restitution accuracies in the cm range. A set of high torque reaction wheels enables rapid rotation into the so-called SSL (Sun Side Looking) orientation which is used to acquire high-priority imaging targets. To point the SAR antenna in the SSL direction, a roll movement of 67.6º is required which is achieved in < 180 s.

The MosaicGNSS receiver of EADS Astrium represents a fully space-qualified receiver that is specifically designed for high robustness and long-term use in a space environment. The receiver comprises a main electronic unit, a single L1 GPS patch antenna and an external low-noise amplifier. The signal correlation is performed in software and up to eight satellites can be tracked simultaneously with the current hardware configuration. A navigation filter ensures a smooth and continuous navigation solution even under restricted GPS visibility.
In the upcoming TerraSAR-X and TanDEM-X missions, the MosaicGNSS receiver supports the onboard timing and provides the basic orbital information for aligning the spacecraft with the ground track and nadir direction. MosaicGNSS navigation solutions will also be transmitted via an inter-satellite link between both spacecraft to support autonomous formation flying and collision avoidance. For precise orbit determination and baseline reconstruction, both spacecraft are equipped with dedicated dual-frequency GPS receivers IGOR (Integrated Geodetic and Occultation Receiver).
Furthermore, the MosaicGNSS receiver serves as an alternative for precise orbit determination in case the IGOR receiver would fail to work. To support this task, a full set of raw measurements is made available in the housekeeping telemetry in addition to the real-time navigation solution. The comprehensive measurement set and the availability of a geodetic grade reference receiver offer a unique opportunity to characterize the in-flight performance of the MosaicGNSS receiver. 17)
The spacecraft is equipped with a monopropellant hydrazine blow-down mode propulsion system for orbit maintenance and safe mode attitude control. A propellant mass of 78 kg is considered sufficient for almost 10 years of orbit maintenance support.
Onboard data handling: The newly developed ICDE (Integrated Control and Data System Electronics) system is being used as the central component for all avionics services. The ICDE core consists of two redundant 32-bit processor modules, implementing the ATMEL ERC32SC (Embedded Real‐time computing Core ‐ 32-bit Single Chip) processor, giving it a processing performance of more than 18 MIPS and enough memory capacity to handle full AOCS and data handling software tasks, leaving sufficient margins in performance and memory capacity for future extensions and redundancy concepts. A dedicated, hot-redundant reconfiguration module provides all necessary surveillance, reconfiguration, command and telemetry functions. The ICDE modules are cross-coupled, providing a fully redundant unit.
The ICDE provides the spacecraft and payload interfaces with the following standard link protocols: MIL-1553 bus, HDLC and SpaceWire. An optional GPS receiver module with optional star sensor processing fits seamlessly into the architecture. It is capable of acquiring and independently tracking up to eight GPS satellites and provides position, velocity and time.
The ICDE uses full duplex UART (Universal Asynchronous Receiver/Transmitter) interfaces to all ”intelligent” onboard equipment, except for the LCT experiment, where a MIL-STD-1553B bus is being used. The ICDE has a mass budget of 12-18 kg and a power demand of 15-30 W, depending on the configuration selected.
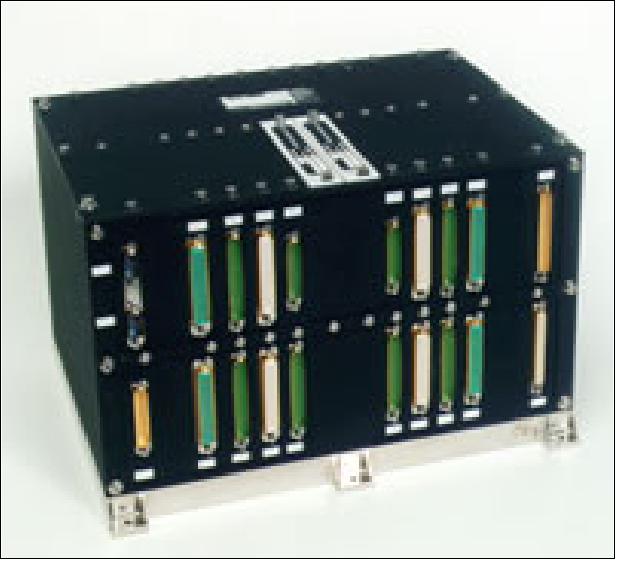
The spacecraft design life is 5 years for operations with a goal of 6.5 years (de-orbiting is planned at the end of the useful lifetime).
Orbit: Sun-synchronous circular dawn-dusk orbit with a local time of ascending node at 18:00 hours (± 0.25 h) equatorial crossing, average altitude = 514.8 km (505-533 km), inclination = 97.44º, nominal revisit period of 11 days (167 orbits within revisit period, 15 2/11 orbits per day). The ground track repeatability is within ± 500 m per revisit period (repeat cycle). Due to its flexibility, TerraSAR-X can cover any point on Earth within a maximum of 4.5 days, and 90% of the surface within 2 days.
Spacecraft reentry (ESA requirement): At the end of its operational life the spacecraft orbit will be lowered to about 300 km (perigee) resulting eventually in enough air drag for a reentry (and a complete fragmentation and destruction of the S/C in the atmosphere).
RF communications: A standard S-band TT&C system with 360º coverage in uplink and downlink is used for satellite command reception and telemetry transmission. The uplink path is encrypted. Generated payload (SAR) data are stored onboard in an SSMM (Solid State Mass Memory) unit of 256 Gbit EOL capacity prior to transmission via the XDA (X-band Downlink Assembly) at a data rate of 300 Mbit/s. The X-band downlink is encrypted. The onboard SAR raw data are compressed using the BAQ (Block Adaptive Quantization) algorithm, a standard SAR procedure. The compression factor is selectable between 8/6, 8/4, 8/3 or 8/2 (more efficient techniques can only be applied to processed SAR imagery).
Both communication links are designed according to the ESA CCSDS Packet Telemetry Standard.
- The X-band antenna is mounted on a deployable boom 3.3 m in length (the only deployable item on the S/C) to prevent interference with the X-band SAR instrument. This arrangement enables simultaneous SAR observations and X-band downlink.
In preparation for the TanDEM-X mission, where the TerraSAR-X satellite will fly in close constellation with TanDEM-X (an identical S/C) for interferometric observations, the TerraSAR-X instrument is furnished with all necessary features for PRF and synchronization between the two spacecraft. In particular, there are 6 sync horns for the omnidirectional emission and reception of radar sync pulses.
S/C wet mass | 1230 kg (bus=549 kg, payload=394 kg, propellant of 78 kg) |
S/C dimensions | 5 m x 2.4 m |
SAR antenna dimensions | 5 m x 0.80 m |
S/C power | 800 W of orbit average power (EOL), 1.8 kW of peak power (BOL); energy storage of 108 Ah capacity of Lithium-Ion battery |
Power distribution | 35-51 V unregulated power bus; converter to 28 V and converter to 115 V 30 kHz AC for TSX-SAR front end |
S/C pointing accuracy | 65 arcsec (3σ) |
RF communications | X-band of 300 Mbit/s link of payload data downlink with DQPSK modulation; S-band uplink of 4 kbit/s (2025-2110 MHz), BPSK modulation; S-band downlink of 32 kbit/s to 1 Mbit/s (2200-2400 MHz), BPSK modulation |
Launch: The successful launch of TerraSAR-X took place on June 15, 2007, from the Russian Cosmodrome, Baikonur, Kazakhstan, on a Russian/Ukrainian Dnepr-1 launch vehicle with a 1.5 m long fairing extension. Launch provider: ISC Kosmotras, Moscow.
- The launch, originally planned for Oct. 31, 2006, had to be shifted several times after an unsuccessful launch of a rocket of the same type in the summer of 2006. The single cause of this launch mishap was discovered and properly corrected.


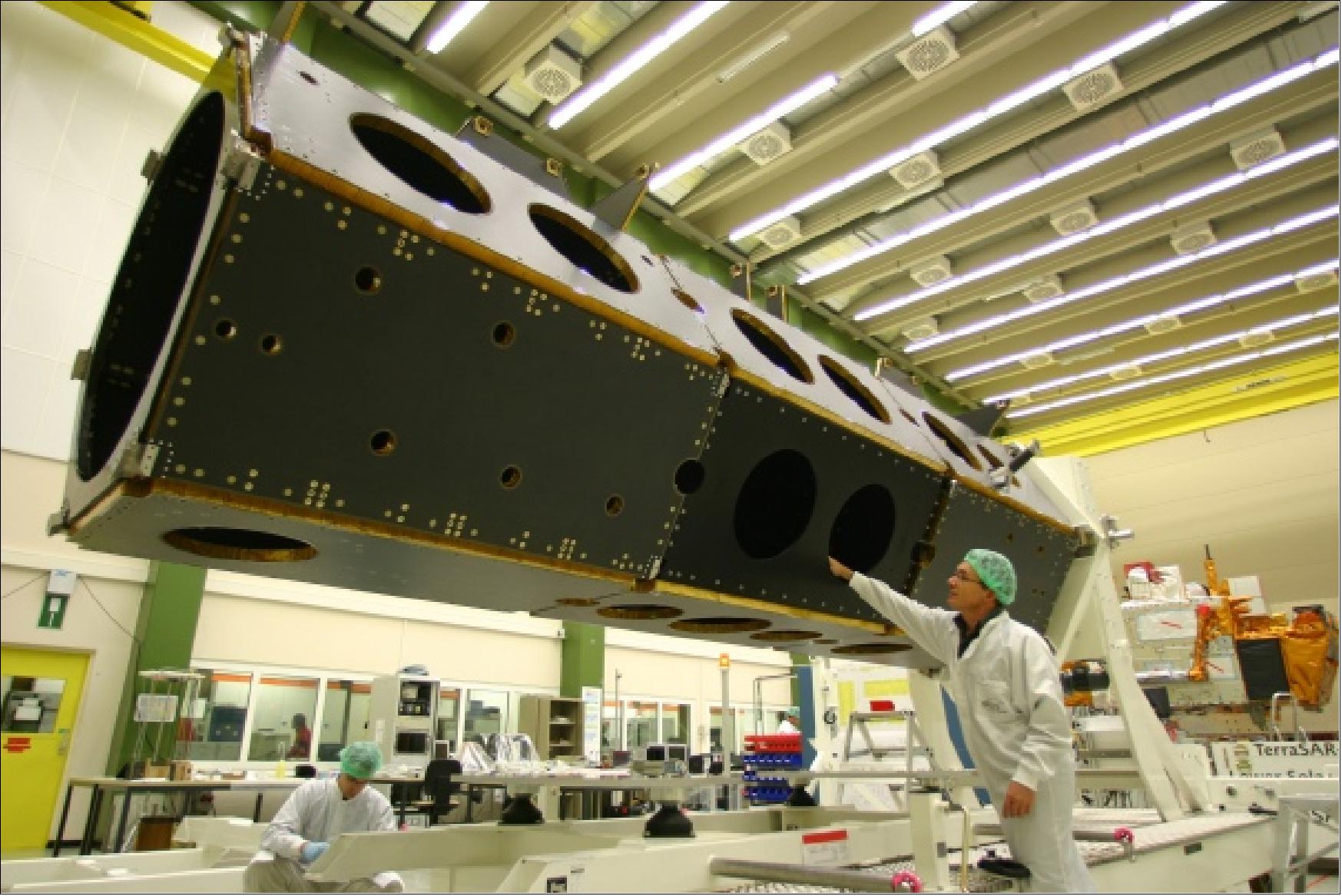
Figure 8: The TerraSAR-X spacecraft bus in the manufacturing process at EADS Astrium (image credit: EADS Astrium)
A Data Repository and Research Subject
- Over its lifetime, TerraSAR-X has acquired more than 400,000 radar images, collecting 1.34 petabytes (1.34 x 1015 bytes) of data in the process. This is equivalent to 1,340,000 gigabytes or the streaming of around 270,000 high-definition feature films, which would take around 60 years to run through.
The satellite’s legacy is becoming more comprehensive, more valuable and more widely used every day. More than 1100 leading researchers from 64 countries are now drawing upon and processing its data as part of 1875 ongoing research projects. Not only does the range of applications encompass the full spectrum of geosciences, including geology, glaciology, oceanography, meteorology and hydrology, but the radar data are also essential for environmental research, land use mapping, vegetation monitoring, and urban and infrastructure planning. Cartography, navigation, logistics, crisis management and defence and security also rely on TerraSAR-X data.
- The satellite itself is the subject of research and development as well, particularly in the field of radar technology. With its flexible design, the radar system enables experiments to be conducted using new imaging modes such as a 'super wide angle' and 'super zoom', similar to a camera being fitted with different lenses. Officially referred to as 'WideScanSAR' and 'Staring Spotlight Mode', these were introduced during the course of the mission and then made available to users. The satellite continues to conduct radar experiments to test new techniques that might be used on future radar missions.
A Third Dimension with TanDEM-X
- Since 21 June 2010, TerraSAR-X has been accompanied by the TanDEM-X satellite, which is almost identical in design. TanDEM-X and TerraSAR-X form the first reconfigurable Synthetic Aperture Radar (SAR) interferometer in space, recording precise height information for creating digital elevation models.
This means that TerraSAR-X is now being used for two missions – the original TerraSAR-X mission and the TanDEM-X mission for three-dimensional mapping of Earth’s surface.
- Despite its unprecedented longevity, the day will eventually come when TerraSAR-X is no longer able to fulfil its tasks. Resources such as propellant and battery capacity are steadily being depleted. However, if there are no major incidents, TerraSAR-X could remain in operation until the end of the 2020s.
Environmental Observation in Future – Tandem-L
- With the TerraSAR-X and TanDEM-X satellite missions, DLR has set new standards in radar remote sensing. Its experts are already working on the next generation of radar satellites for climate research and environmental monitoring, in the form of Tandem-L, a highly innovative radar satellite mission. This could see Germany provide a system for the objective recording of the environment and the observation of environmental changes all over the world.
The goal is to provide critical information to tackle highly relevant issues. For example, the latest report by the Intergovernmental Panel on Climate Change (IPCC) calls for the development of climate protection measures and the review of measures taken on a global scale, as a matter of urgency.
- With Tandem-L, it would be possible to record a large number of dynamic processes in the biosphere, geosphere, cryosphere and hydrosphere with unprecedented quality and resolution. The new satellite constellation could provide up-to-date 3D imaging of Earth's entire landmass on a weekly basis and measure seven essential climate variables simultaneously.
In doing so, Tandem-L would make a significant contribution towards a better understanding of processes that are now seen as drivers of local and global climate change.
Big Data for Earth Observation
- The satellite has already delivered 303,714 images. The data is received via a global network of ground stations and processed and evaluated by experts at the DLR Earth Observation Center (EOC). Even the first analyses document indisputable details of climate change, including the retreat of glaciers across the globe. Approximately 1000 scientists from more than 50 countries are now using the data for their research – and demand is on the rise.
Global radar images are of particular value to environmental and climate research. DLR ensures access to the images in the long term in the German Satellite Data Archive in Oberpfaffenhofen.
- During this time, GSOC (German Space Operations Center) sent more than 1.85 million commands to TerraSAR-X, and an additional 1.4 million commands to control the orbiting TanDEM-X satellite. A particular challenge, both during the development and in operation, was and is the 'double-helix dance' of the two radar satellites.
The tightest flight formation between TerraSAR-X and TanDEM-X was at a distance of 120 m distance perpendicular to the direction of flight – at an average speed of 7.6 km/s. The exceptional performance and success of the mission are not least down to the close interdisciplinary collaboration within DLR. In Oberpfaffenhofen, almost 100 staff from four DLR institutes have combined their expertise such that they have mastered the entire process chain of the TerraSAR-X and TanDEM-X mission for 10 years now.
The future
- The exceptional life of the satellite has been possible thanks to careful operation and robust construction. Only about half of the fuel supply has been consumed and the performance level of the batteries is approximately 72 per cent, so the experts expect TerraSAR-X will continue to operate for another five years.
The twin satellite TanDEM-X also shows no signs of fatigue, meaning that more high-resolution elevation images will be generated and the global data set will be enhanced by autumn 2017. The focus is on areas undergoing strong processes of change, and are therefore of particular scientific interest. These include the coastal regions of the Antarctic, Greenland and the permafrost regions, and the Amazon rainforest.
With regard to the extent and effect of climate change, Tandem-L could provide important information that is still lacking – for improved scientific forecasts and the social and political recommendations for action that are based on this. The concept builds on the experience and exceptional success of the TerraSAR-X and TanDEM-X missions. If the mission proposal gets the 'green light', Tandem-L will take radar remote sensing into the next era of technology and applications in 2022.
- With the X-band SAR family, Germany has developed a globally recognized expertise and a unique selling point for decades. In order to ensure this leadership role in the future, the continuation of the X-Band family is being carried out at the DLR Space Administration. The future lies in an even higher resolution with wider observation swaths. This is intended to continuously provide the scientific, governmental and commercial stakeholders with data.
Mission Status
• June 14, 2022: The German radar satellite TerraSAR-X celebrates its 15th anniversary in space on 15 June 2022. TerraSAR-X allows researchers worldwide to document and better understand the changes on our planet. TerraSAR-X serves two missions — the TerraSAR-X mission and the TanDEM-X mission for three-dimensional mapping of Earth's surface. Experts are already working on the next generation of radar satellites for climate research and environmental observation — Tandem-L. 18)After 15 years in operation, TerraSAR-X has completed 83,050 orbits of Earth, having travelled approximately 3.59 billion kilometres.


• January 25, 2022: The TerraSAR-X mission had been operating nominally providing monostatic SAR imagery in its 15th year on orbit. Despite being well beyond its mission design life, the satellite was fully functional; it had enough consumables for a mission life until at least 2026. 19)
• April 22, 2021: The TerraSAR-X ESA archive collection consists of TerraSAR-X and TanDEM-X products requested by ESA-supported projects over their areas of interest around the world. The dataset regularly grows as ESA collects new products over the years. 20) TerraSAR-X/TanDEM-X Image Products can be acquired in six image modes with flexible resolutions (from 0.25m to 40m) and scene sizes. Thanks to different polarimetric combinations and processing levels the delivered imagery can be tailored specifically to meet the requirements of the application. The following list delineates the characteristics of the SAR imaging modes that are disseminated under ESA Third Party Missions (TPM): StripMap, SpotLight, Staring SpotLight, High-Resolution SpotLight, ScanSAR, WideScanSAR. The following list briefly delineates the available processing levels for the TerraSAR-X dataset: SSC (Single Look Slant Range Complex) in DLR-defined COSAR binary format, MGD (Multi Look Ground Range Detected) in GeoTiff format, GEC (Geocoded Ellipsoid Corrected) in GeoTiff format, EEC (Enhanced Ellipsoid Corrected in GeoTiff format.
• February 1, 2019: The Thwaites Glacier, one of the most fragile glaciers in western Antarctica, is melting inexorably into the Amundsen Sea at an ever-increasing rate. Until now, it has been responsible for approximately four per cent of the global rise in sea level and will cause the oceans to rise by over 65 centimetres in future as its remaining ice melts. With the German radar satellites TerraSAR-X and TanDEM-X, it was possible to observe Thwaites Glacier and other polar regions at regular intervals, with high resolution and in three dimensions. Scientists from DLR ( German Aerospace Center) have generated special TanDEM-X elevation models to better understand and predict the melting processes and changes occurring on Thwaites Glacier. The results of the NASA-led study have now been published in the scientific journal Science Advances. 21) 22)

- There is a gigantic, 350-meter cavity in the floor of the Antarctic glacier, with the penetrating seawater continuously eating further into the ice. Experts have long suspected that Thwaites is not firmly attached to the bedrock beneath it, but the size of the cavity and the formation of subglacial channels was as surprising as it was alarming. Satellite data acquired by the partners from the United States, Germany and Italy revealed that a total of 14 billion tons of ice have already been washed out, mainly in the last three years. The melt rate was calculated based on TanDEM-X images.
- In addition, the TanDEM-X elevation models reveal the glacier's special dynamics. The changes in the ice surface elevation were measured with millimetre accuracy, allowing important conclusions to be drawn about the underlying melting processes. Scientists thus discovered that although the glacier surface is rising, the overall thickness of the ice is decreasing. For the detailed time series analyses, the DLR experts ordered a total of 120 TanDEM-X images over the period from 2010 to 2017. A time series of elevation models were created from these using the global TanDEM-X elevation model. The highly accurate determination of the glacier's structure is achieved thanks to high-precision interferometric processing, geocoding and calibration of TanDEM-X images, which was implemented at the DLR Microwaves and Radar Institute. New radar remote sensing technologies and methods make it possible for scientists to conduct more targeted research into critical climate processes and further improve predictive models. The latest findings on the development of Thwaites Glacier provide a valuable guide for climate and environmental research.
• June 2018: The missions TSX (TerraSAR-X) and TDX (TanDEM-X jointly share the same space segment consisting of two almost identical satellites orbiting in close formation. They are operated using a common ground segment, that was originally developed for TSX and that has been extended for the TDX mission. A key issue in operating both missions jointly is the combination of the different acquisition scenarios: TSX requests are typically single scenes for individual scientific and commercial customers, whereas the global DEM as well as science products require a global mapping strategy. Thus the TSX mission goal is retained and served by both satellites. TSX and TDX reached their nominal lifetime at the end of 2012 and 2015, respectively. Therefore it is worth having a look at the status of the irretrievable on-board resources. Especially the propellant and the battery status are crucial factors determining the future progress and remaining duration of the mission.
- By June 2018 TSX will have exceeded its nominal lifetime by 5.5 years and TDX by 2.5 years. Fuel and battery status are considerably better than predicted. The radar performance and calibration of the individual satellites are still within specification or better and no indication of any degradation is noticeable at the moment. As both satellites were still working very well and had plenty of resources left, they planned to continue the mission beyond 2020 with a focus on selectively updating and improving the global TanDEM-X DEM and generating a global Change DEM as a self-contained product.

• February 9, 2018: The satellite duo, TerraSAR-X and TanDEM-X, continue to orbit in close formation to make bistatic observations for scientific applications. In addition, observations are being made to fill small areas in the DEM and to improve the quality of the data. Furthermore, observations are being conducted to capture topographic changes. 24) Both satellites have used their consumables frugally; each spacecraft spent so far somewhat less than half a tank volume of hydrazine; the batteries are in good operational condition, and the quality of the radar imagery remains excellent since the start of the mission. The project expects a continuation of operational services of the two missions to at least 2020, subject to unforeseen events. A paper has been published providing forest maps on the basis of interferometric TanDEM-X data. 25)

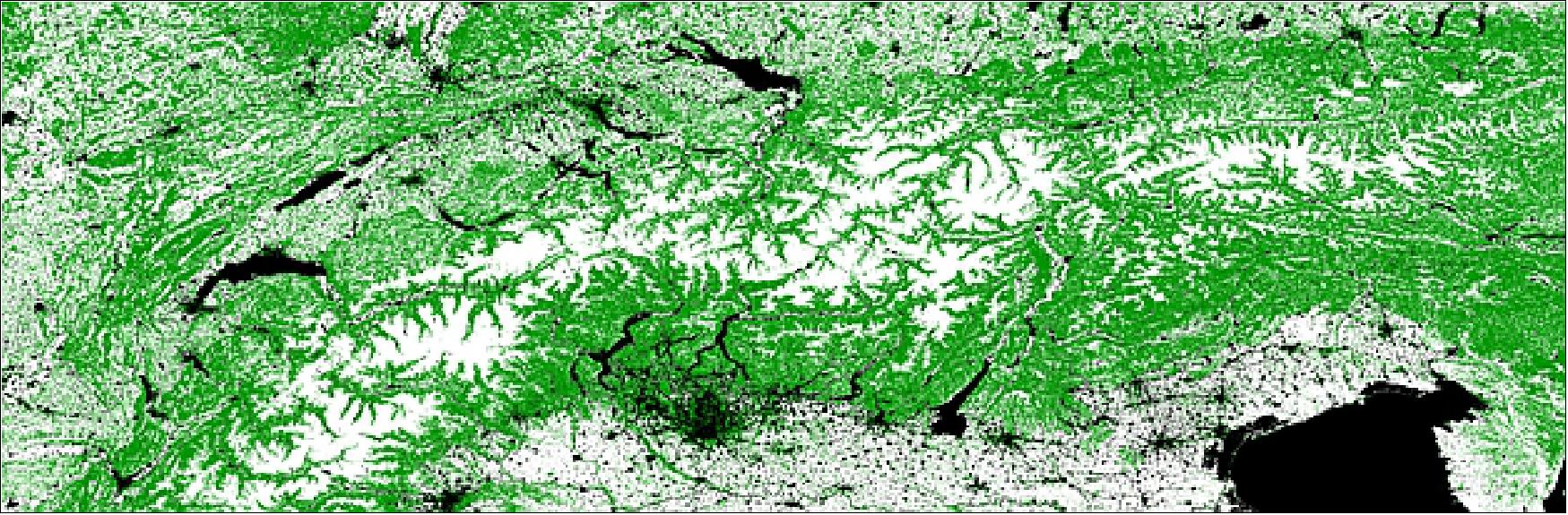
• June 15, 2017: the TerraSAR-X spacecraft and its payload were 10 years on orbit. Designed to return unique images of the Earth for five years, the German radar satellite TerraSAR-X has outdone itself. The satellite has been in operation for twice that time – and there is still no end in sight to its service. Since its picture-perfect launch on 15 June 2007 from the Russian cosmodrome in Baikonur, the German Aerospace Center (Deutsches Zentrum für Luft- und Raumfahrt; DLR) TerraSAR-X mission has exceeded all expectations. 27)
- TerraSAR-X and its twin TanDEM-X, which was launched three years later, have been flying in formation since 2010. Together, they generate the highest-resolution three-dimensional images of the Earth's surface. To this day, the special mission concept of TanDEM-X, the first bistatic SAR interferometer in space, developed at the DLR Microwaves and Radar Institute, is one-of-a-kind. Developed and constructed by Airbus Defence and Space teams from Friedrichshafen for the DLR (German Aerospace Center), the satellite orbits at a height of 514 km and provides radar imagery to a wide variety of scientific and commercial users. 28)
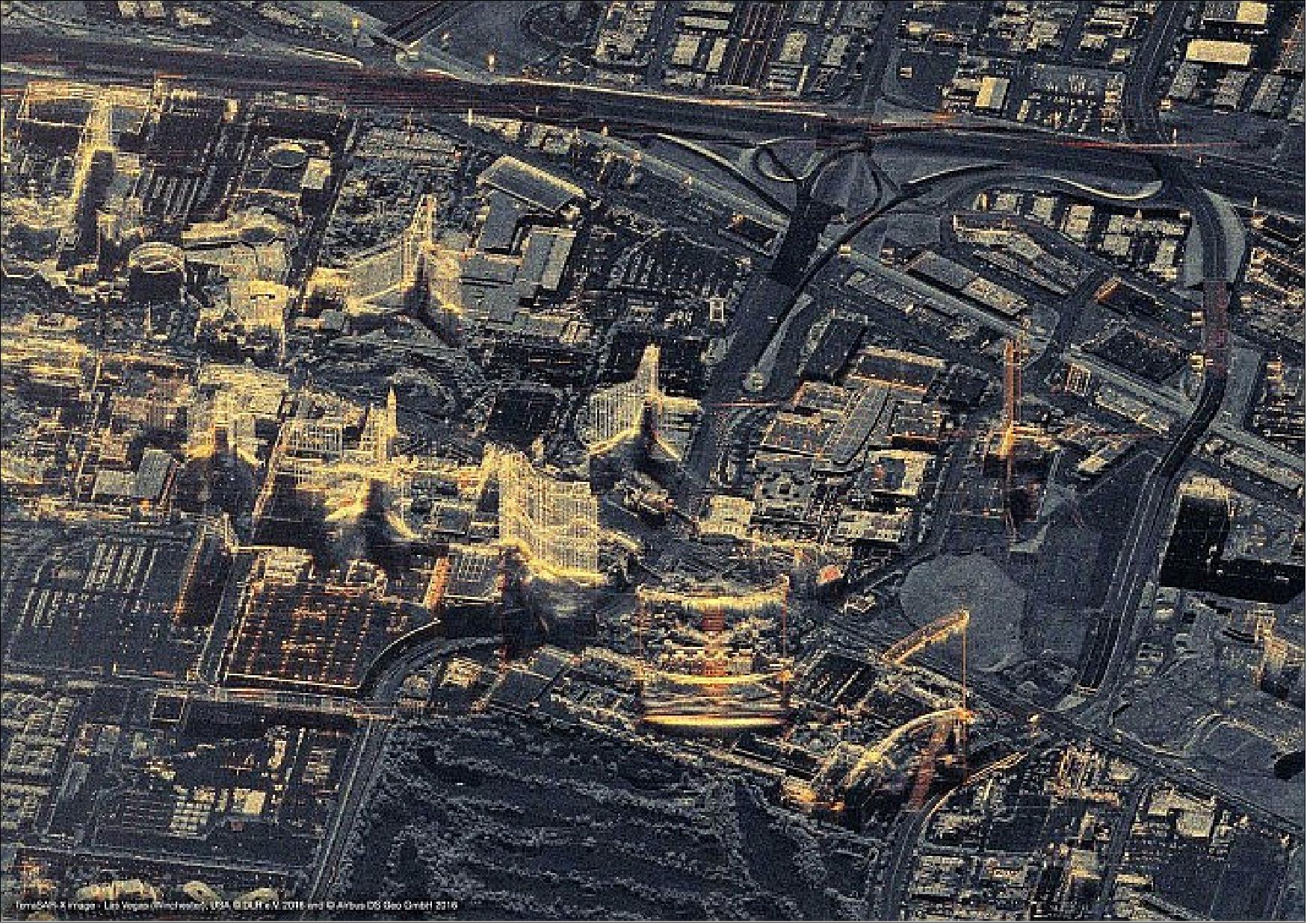
• January 13, 2017: The satellite duo, TerraSAR-X and TanDEM-X, continue to orbit in close formation to make bistatic observations for scientific applications. Both satellites have used their consumables frugally; each spacecraft spent so far about half a tank volume of hydrazine; the batteries are in good operational conditions, and the quality of the radar imagery has remained excellent since the start of the missions. The project expects a continuation of operational services of the two missions until about 2020 — these predictions depend of course on the assumption that no unanticipated events occur. After all, in June 2017, TerraSAR-X will be 10 years on orbit. 30) The measurement of the polar regions by TSX/TDX is so precise that glacier movements can be seen in the centimetre range, and changes in elevation caused by ice melting are measured in the meter range. Initial studies have shown that in some regions, glaciers are losing up to 30 m thickness per year in the area of the glacier tongues.
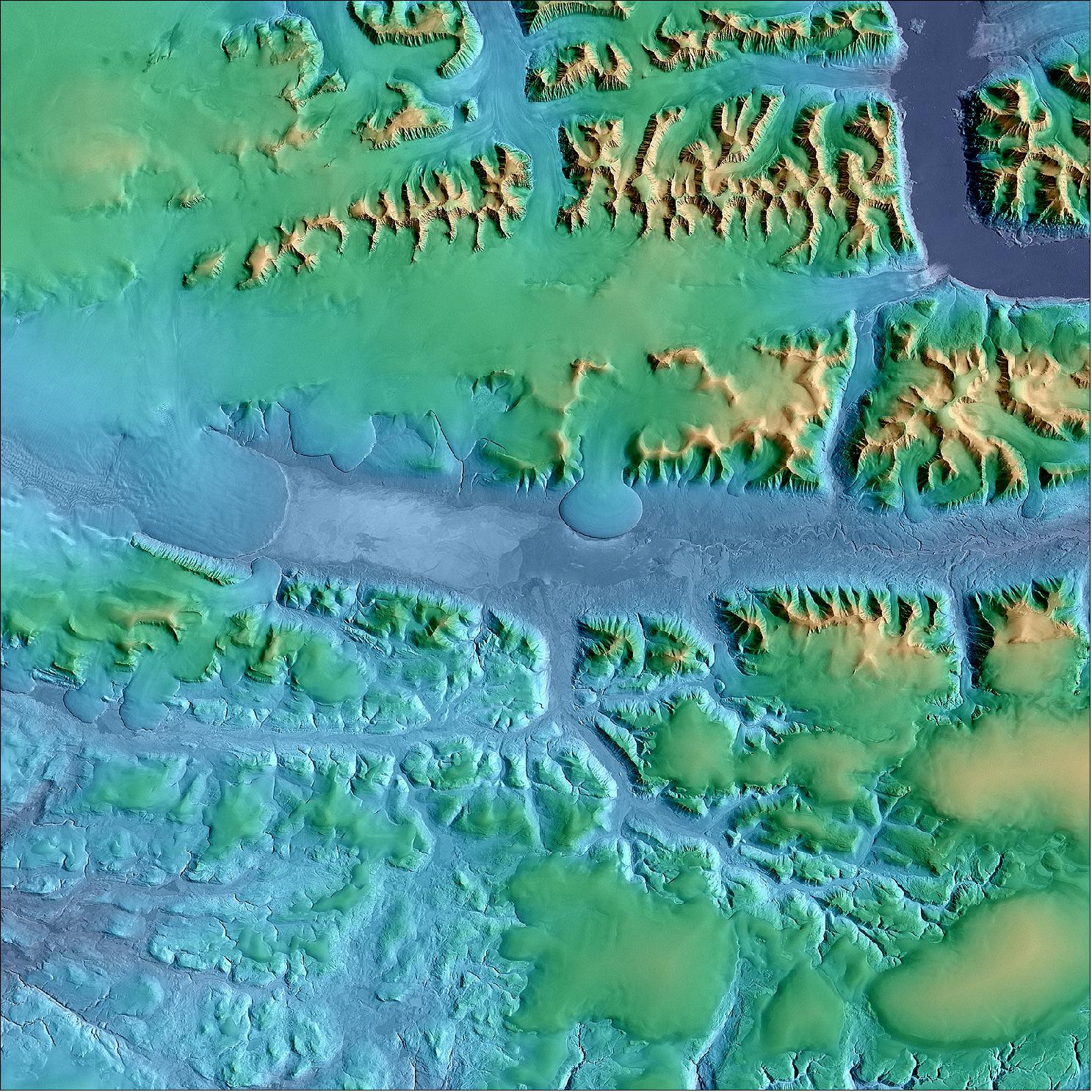
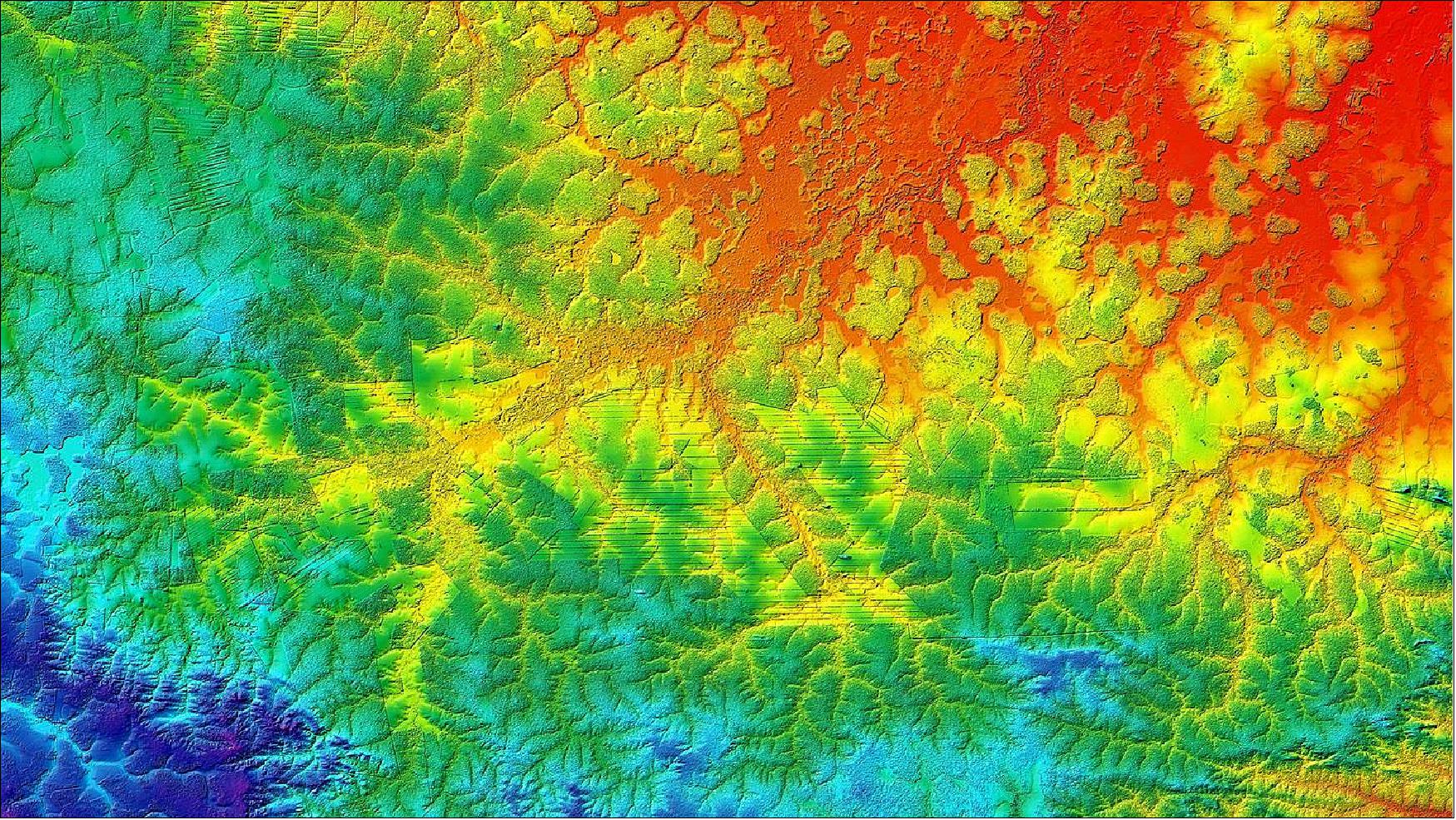
• October 17, 2016: The German satellite duo TerraSAR-X and TanDEM-X have consistently delivered one-of-a-kind Earth observation data since 2007 and 2010, hence shaping the international research landscape. Now, scientific users from across the globe have gathered for the TerraSAR-X and TanDEM-X Science Meeting at DLR (German Aerospace Center) in Oberpfaffenhofen, where they will discuss the results obtained from the data and define requirements for future remote sensing technology. 31)
• July 2016: Since the launch of the TDX (TanDEM-X) mission in 2010, the two missions TSX (TerraSAR-X) and TDX share a joint space segment consisting of both TSX and TDX and a common ground segment which was first developed for TSX and had been later extended for TDX. While TanDEM-X uses both satellites (nominally one in receive-only mode) for an acquisition, TerraSAR-X data are acquired by either one of the two satellites. It is this sharing of one ground segment which necessitated ongoing updates of the TerraSAR-X ground segment in accordance with the TanDEM-X mission constraints. 32) Table 2 summarizes the acquisition mode portfolio. In response to a growing demand for larger coverages at moderate resolution and for higher resolution with more radiometric looks, the original portfolio was extended by the new Wide ScanSAR and Staring Spotlight mode in 2013. Users can directly order future TanDEM-X data takes and request products generated from archived Level 0 data through catalogue ordering. The mission offers different acquisition modes, including StripMap data in fully polarized and Along-Track Interferometric configurations, although these are used for specific campaigns. The Dual-Receive Antenna (DRA) configuration, while offering redundancy, affects the acquisition and downlink timeline, preventing data replay during acquisition. To address limitations in the availability of ATI products, an experimental mode called ATIS, using a single antenna, is also offered. Users can access data from these experimental modes through catalogue orders.
Mode | Coverage Azimuth x Range (km2) | Resolution Class (m) |
ScanSAR Wide (SCW) | 200 x (194–266) | 40 |
ScanSAR (SC) | 150 x 100 | 18 |
StripMap (SM) | 50 x 30 | 3 |
Spotlight (SL) | 10 x 10 | 1.7 - 3.5 |
High-Resolution Spotlight (HS) | 5 x 10 | 1.4 - 3.5 |
300 MHz High-Resolution Spotlight (HS 300) | 5 x (5-10) | 1.1 - 1.8 |
Staring Spotlight (ST) | (2.5 – 2.8) x ~ 6 | 0.24 azimuth, 1.0 range |
- The science phase of the mission began in September 2014 when TanDEM-X moved away from TerraSAR-X, maintaining a distance of 76 km behind TerraSAR-X. Users can directly order data from all modes, and once data is archived, catalog ordering is possible. The mission has adopted various flight configurations, including pursuit monostatic, bistatic, and dual-receive antenna modes, each with its own implications on data acquisition and quality. To ensure data quality consistency, measures are taken to align the performance of TanDEM-X (TDX) with TerraSAR-X (TSX), including roll angles and time delays. TSX may also acquire data when TDX exceeds certain baseline thresholds. Simultaneous operation of TSX and TDX instruments in active mode increases imaging opportunities and reduces conflicts among acquisition hot spots. Data from PSM TanDEM-X acquisitions can be processed into SAR products, similar to TerraSAR-X products, with some differences in performance and characterization parameters. Near Real-Time (NRT) data processing has been improved over the years, allowing for quick data dissemination, including support for maritime applications like ship and oil detection. The mission features flexible operational functions between TSX and TDX, and the satellites are designed for an extended lifetime.
- The mission has made significant progress in generating a global Digital Elevation Model (DEM), with more than 80% of land surfaces covered by March 2016. The system undergoes continuous monitoring and verification for accuracy. Compared to SRTM the TanDEM-X DEM features a much lower percentage of void areas, especially in desert areas, a result of the re-acquisition at lower incidence angles and hence better SNR. Further details on the global DEM quality can be found in reference 34) Beyond the generation of a global TanDEM-X DEM as the primary mission goal, a dedicated science phase was aimed at demonstrating the generation of even more accurate DEMs on local scales and applications based on along-track interferometry and new SAR techniques, with a focus on multistatic SAR, polarimetric SAR interferometry, digital beamforming and super-resolution. As both satellites are still working very well and have plenty of resources left, an agreement to continue the mission beyond 2015 was concluded between DLR and Airbus Defence & Space. Acquisition of interferometric data for and generation of local DEMs of even higher accuracy levels (posting of 6 m and relative vertical accuracy below 1 m) is the key objective for this new mission phase. If the baseline geometries are suitable for further scientific experiments will be included in the timeline as well.

• July 30, 2015: Airbus Defence and Space, owner of the commercial distribution rights for TerraSAR-X data, and ESA have signed a contract securing the continued supply of TerraSAR-X data for the Copernicus Data Warehouse. The agreement is valid until the end of 2020, thus continuing the successful cooperation between Airbus Defence and Space and ESA for the provision of TerraSAR-X data to public institutions across Europe in place since 2008. TerraSAR-X has been a key data source, particularly for activities addressing emergency and security-related issues, reliable monitoring needs, and land cover change, both in Europe and beyond. It's unique reliability and high accuracy make it an ideal data source within ESA's multi-mission approach. The agreement includes the provision of archived and newly acquired TerraSAR-X data for the Copernicus core datasets in the area of maritime monitoring, as well as additional datasets for further maritime applications, particularly sea ice monitoring.
• February 2015, the TanDEM (TanDEM-X and TerraSAR-X) missions are operating nominally, supporting the science mission. The new science phase started in September/October 2014, applies to both missions of the formation, TerraSAR-X and TanDEM-X, and is planned to last until the end of 2015. 36)The first part of the science mission, from Sept. 20, 2014, to the end of March 2015, is the so-called “Pursuit Monostatic Phase“, in which the TanDEM-X spacecraft is flying at a distance of 76 km behind its twin, TerraSAR-X. Each spacecraft acquires monostatic data of the same area which are then interferometrically ground-processed. At the end of March, the formation changed again; the “Bistatic Phase” started in which bistatic acquisitions with a large horizontal baseline (up to 3600 m) were taken up to September. After a drift phase of about a month, the bistatic phase continued in October with a small baseline of ~250 m, until the end of the year. Independently from the science mission, the TerraSAR-X mission (generation of non-interferometric SAR products), is being served in parallel from both spacecraft.
• October 10, 2014: After four years of successful data acquisition for the new global topographical map of Earth, the Science Phase is beginning. The radar satellite TerraSAR-X has been orbiting the Earth since June 2007; in June 2010 its twin, TanDEM-X, followed it into space. For almost four years, the two satellites have been operated in a close flight formation by DLR (German Aerospace Center). During this time, the satellites have been acquiring data to generate a new global topographical map of the Earth. 37) 38) The goal of the TanDEM-X mission is to produce a highly precise, three-dimensional image of the Earth with uniform quality and unprecedented accuracy. For large parts of the Earth, there currently exist only approximate, inconsistent or incomplete elevation models derived from different data sources and collection methods. TanDEM-X is filling in these gaps and providing a homogeneous elevation model to be used as an indispensable basis for numerous commercial applications and scientific investigations.
• September 17, 2014: The initial preparations for the science phase began with the transition to the new formation on 17 September 2014. TanDEM-X moved away from TerraSAR-X and has been flying at a distance of 76 km behind its twin since 20 September 2014. This has resulted in a time delay of 10 seconds. Since the Earth rotates at approximately 500 m/s at the equator, the orbit of TanDEM-X has also had to be displaced laterally by 5 km so that both satellites are imaging the same area (footprint) on the surface. TanDEM-X continues to follow a helical orbit. Unlike the imaging for the DEM (Digital Elevation Model) of the Earth, the helix will not be adhered to for weeks at a time; instead, significantly greater variations will be permitted. The distance between TanDEM-X and the nominal orbit of TerraSAR-X will vary between 0 and 1000 m over the next five months. The aim is to continue to operate both satellites using interferometry, to enable three-dimensional imaging of the surface of the Earth to continue. After changing the orbit of TanDEM-X in recent weeks, the two satellites are being operated independently of one another, in what is known as 'Pursuit Monostatic Mode'.
- The advantage of this new orbital configuration is that the distance between the satellites – the baseline – can be made substantially more flexible. In the new orbital configuration, data for elevation models can be generated with an elevation accuracy of a few tens of centimetres, for example. This opens up new applications in the areas of the geosphere, cryosphere and hydrosphere. This data is unique and will be used in the investigation of volcanic eruptions, the melting of ice as well as, for example, tomographic imaging of cities. The orbital configuration will be changed again in the spring of 2015 to enable other applications and demonstrations. The imaging for the DEM was completed, with the exception of a few images. Final DEM tiles for more than a quarter of the land area – for example, for the flat areas of Australia, North America, Siberia, South and West Africa and South America – have already been processed. The success of TanDEM-X forms the basis for the development of innovative radar technologies. The aim is to achieve a significantly higher imaging capability, which will exceed that of TanDEM-X by a factor of 100. While TanDEM-X only enables one global image of the Earth to be acquired per year, Tandem-L will image the entire landmass of the Earth at a higher resolution twice a week. Hence, Tandem-L will be able to capture dynamic changes on the surface of the Earth with the required imaging repetition frequency and provide urgently needed information for solving topical scientific questions involving the areas of the biosphere, geosphere, cryosphere and hydrosphere.
• September 16, 2014: The lava outflow on the Holuhraun field northeast of Iceland's Bardarbunga volcano continues unabated. The lava field has grown to cover an area greater than 25 km2. In this satellite image, the extent of the lava field is revealed using different colours. 39)Researchers from DLR/IMF (Institut für Methodik der Fernerkundung), Oberpfaffenhofen are continuing to monitor the area. Radar images can be used to analyze changes to Earth's surface throughout the entire process. To create this image, three sets of data were acquired at different times, but from the same viewpoint, and then superimposed. They date from 13 August, 4 September and 15 September 2014 and were acquired by the German radar satellite TerraSAR-X. Yellow shows the growth of the lava field between 13 August and 4 September; red shows the expansion between 4 and 15 September. It is obvious that the area has doubled in the shorter second period. A second eruption area can also be seen as a small red spot in the lower right corner of the image.
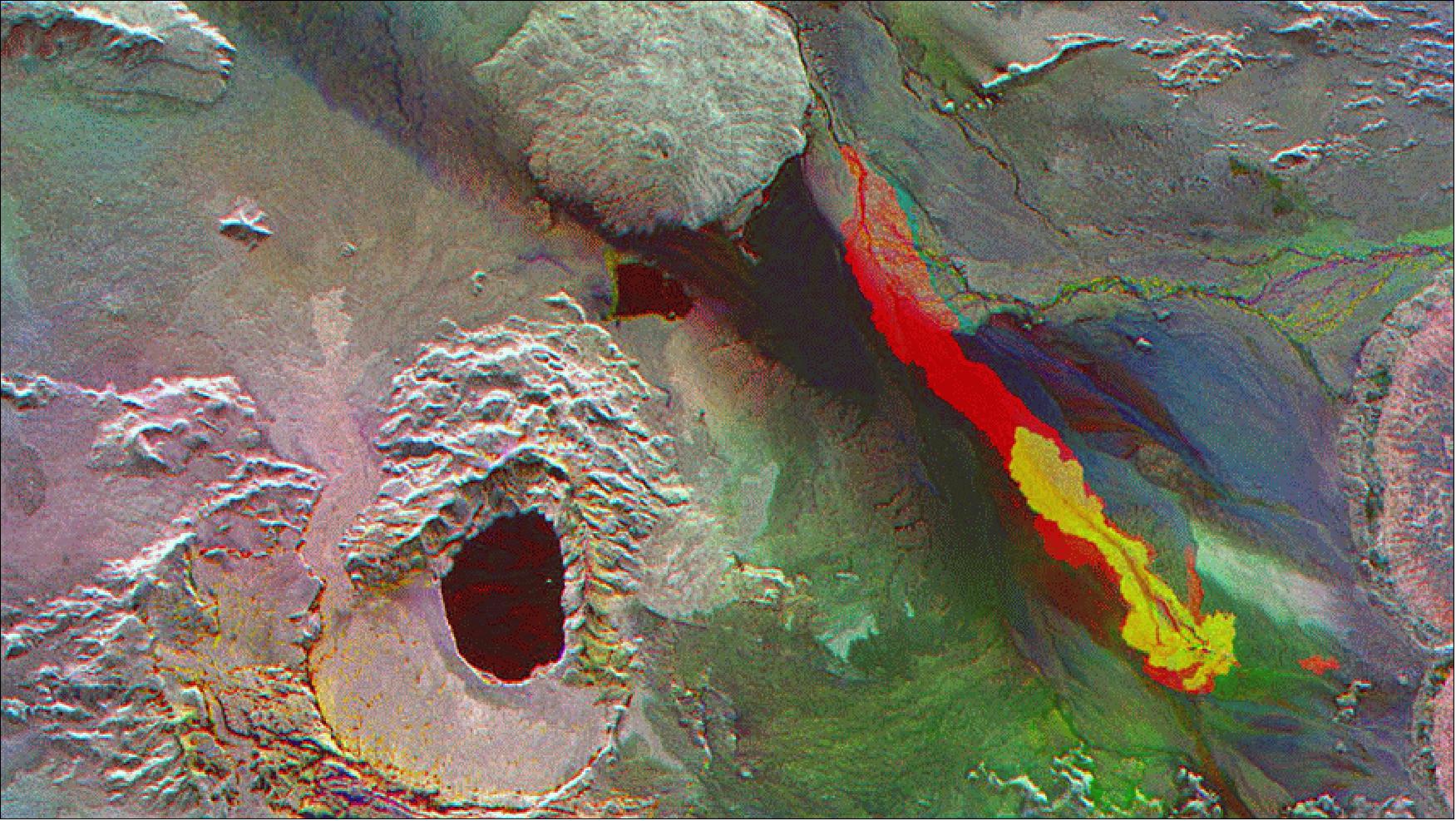
• September 10, 2014: Bardarbunga, (Bárðarbunga) in Iceland, one of the largest volcanoes in Europe and located beneath the biggest glacier in Europe, became active again in mid-August. For several years now, DLR researchers have been keeping a close eye on Bardarbunga and the system of volcanoes associated with it - an enormous network of subterranean magma channels, vents and craters. TerraSAR-X has now provided important data on the volcano's latest activity. 40)Activity in the Bardarbunga volcano system began with earthquakes having magnitudes of up to of 5.7 on the Richter scale, indicating that magma beneath the surface was moving and rising. On 29 August, a lava flow escaped from a breach in the Holuhraun lava field to the north of the glacier - an ice-free area. The Holuhraun lava field has now grown to cover an area of over 19 km2.
- The image shown here covers an area of approximately 30 km x 50 km, and the recently ejected lava covers an area of roughly 10 km2. The brighter areas, which are also highlighted in red for better visibility, indicate changes in amplitude (the intensity of the radar signals returned to the satellite). Since the rough surface of freshly-cooled lava reflects the radar signals back very strongly, it appears bright and is easily visible on the lava flow at the lower right in the image or on the two arcs at the right edge of the image (the northern edge of Vatnajökull). Smooth surfaces, such as water, reflect the incoming radar signals away from the satellite and so appear dark on the images.

Parameter | TerraSAR-X | TanDEM-X |
Internal calibration: |
≤ 0.15 dB / ≤ 0.5º |
|
Geometric calibration: Pixel location accuracy (azimuth/range) | 12.1 cm / 10.7 cm | 9.2 cm / 11.0 cm |
Antenna pointing: (azimuth / elevation) | 0.01º / 0.04º | < 0.02º / < 0.02º |
Antenna Model: shape and gain-offset | ≤ ±0.2 dB | ≤ ±0.2 dB |
Radiometric calibration: |
|
|
- New imaging modes (introduced in July 2013):
- To meet the increasing demand for higher resolution and more detail, as well as increased coverage, the capabilities of the TerraSAR-X mission were extended by implementing two new modes. The flexible instrument design allows such upgrades even with the satellites in orbit. A Wide ScanSAR product with an extended swath width of up to 260 km and a Staring Spotlight product with an intrinsic azimuth resolution of 24 cm, which is being traded for a considerably improved radiometric resolution, have been added to the product portfolio. The staring Spotlight mode even further increases the high geometric resolution of the standard High-Resolution Spotlight product. By widening the azimuth beam steering angle range, thereby extending the synthetic aperture, an azimuth resolution of 0.24 m can be achieved. It is available as single polarization 300 MHz range bandwidth variant. The scene extent varies between 2.5 km and 2.8 km in azimuth and 4.6 km to 7.5 km in range. The standard ScanSAR covers a 100 km wide swath. In order to meet the increasing demand for wider swath coverage, the new Wide ScanSAR mode was introduced. This new ScanSAR variant offers a 195 km to 266 km wide swath by employing 6 specific beams with a reduced azimuth resolution of 40 m and a variable range bandwidth of 50 MHz to 100 MHz.
Parameter | Sliding Spotlight (HS, 300 MHz) | Staring Spotlight (ST, 300 MHz) |
Scene size | Azimuth: 5 km | Azimuth: 2.5 km - 2.8 km |
Full performance including angle range | 20º - 55º | 20º - 45º |
Data access incidence angle range | 15º - 60º | 15º - 60º |
Azimuth steering angle | ±0.7º | ±2.2º |
Azimuth resolution | 1.1 m (single polarization) | 0.24 m (single polarization) |
Ground range resolution | 1.1 m – 1.8 m | 0.9 m – 1.8 m |
Polarizations | HH or VV (single) | HH or VV (single) |

Parameter | Four Beam ScanSAR | Six Beam ScanSAR |
Number of subswaths | 4 | 6 |
Scene size (Nominal L1b product length) | Azimuth: 150 km | Azimuth: 200 km |
Full performance incidence angle range | 20° - 45° | 15.6° - 49° |
Data access incidence angle range | 15° - 60° | 15.6° - 49° |
Elevation beams | 27 | 10 |
Azimuth resolution | 18.5 m | 40 m |
Range bandwidth | 100 and 150 MHz | 81.25 to 31.25 MHz |
Ground range resolution | 1.70 m - 3.49 m | 6 m - 10 m |
Polarizations | HH or VV (single polarization) | HH, VV (single polarization), VH (cross polarization) |
-The new operational TerraSAR-X 6 beam Wide ScanSAR mode with cross-track coverage of up to 260 km shows excellent results in NESZ, ambiguity ratios and resolution. Taking into account, that during the design of the satellite, only swath widths of 100 km were required, the challenging task was achieved with an iterative optimization approach. The outcome is a very impressive achievement for the mission. 42)Both GEOINT (Geospatial Intelligence) and IMINT (Image Intelligence) increasingly make use of high-resolution SAR data. The new TerraSAR-X Staring Spotlight mode provides the highest spatial resolution presently available on a commercial spaceborne SAR system. The TerraSAR-X Staring Spotlight mode provides a means to assess man-made objects more precisely. Image measurements of size, shape and position are more accurate, target interpretation is more reliable. 43

• June 2014: LTSM (Long-Term System Monitoring) of the TerraSAR-X and TanDEM-X missions. In order to guarantee a stable quality of SAR products and to monitor the correct operation of the entire SAR systems, both systems are regularly monitored. LTSM covers the SAR system-related parts of the combined TerraSAR-X and TanDEM-X system (space & ground segment). The detection of long-term SAR system performance changes is the primary subject of the LTSM. The objective of LTSM is the collection and supply of information that can be used to initiate (if needed and feasible) dedicated actions to maintain the specified SAR product quality. Furthermore, the LTSM can help to reveal the causes for events that seem to be by chance (e.g. non-reproducible failure in command execution) by analyzing similar cases (detection of coincidences with other events, operational or environmental conditions). 44)
Instrument operations: Continuous monitoring of both SAR instruments in orbit is required to detect degradations of the satellite hardware and to compensate them by adapting the respective parameters. Therefore, the instrument status of TSX-1 and TDX-1 is checked regularly. The main source is the telemetry data of the satellites, downlinked via the S-band. In addition, complementary ground segment data is evaluated in order to derive regular statistics on instrument load (commanded and executed acquisitions) etc.
Monitoring of TRMs (Transmit/Receive Modules): For characterizing individual TRMs simultaneously a method based on orthogonal codes is applied. Regular antenna health checks, based on the automated acquisition of special system datatakes at regular intervals, monitor the TRM transmit and receive gain, as well as transmit and receive phase for both instruments in-flight. Possible degradation or drifts can be found by depicting gain and phase trends over time. All active TRMs work within the established limits and no trend can be observed, indicating the stability of the TSX-1 instrument and the TRM settings, respectively. The amplitude deviation (1σ) stays below 0.1 dB and the phase deviation is under 1° for all TRMs.
Doppler centroid: SAR image quality is affected by the Doppler centroid. The main contribution, an effective squint angle due to Earth rotation, is compensated by Total Zero Doppler Steering. The residual Doppler centroid is estimated for each datatake by the operational SAR processors. As the Doppler centroid frequency of the SAR signal is related to the location of the azimuth beam centre, the evaluation of Doppler estimations over a number of datatakes can reveal antenna mispointing in flight direction. This sensitivity of the SAR system has been exploited in the monostatic commissioning phase of the satellites. The mean Doppler values are concentrated around 0 Hz mainly (95 % of the total acquisitions) in a tube of ±120 Hz, providing a stable image quality over the mission time. The data collected over the operational mission time does not show any trends. Outliers could be identified as non-nominal satellite conditions (e.g. GPS anomalies).
- The measurements and the extended analyses performed for long-term system monitoring of both SAR systems TerraSAR-X and TanDEM-X show very high stability of the instrument performance. Since the launch of the respective satellite, no degradation in the performance of both instruments has been observed. All parameters show a constant behaviour. Hence, by all these measurements performed for LTSM, it can be concluded that TerraSAR-X and TanDEM-X could be characterized and adjusted precisely, achieving the end a highly accurate and stable SAR System (Ref. 44).
• January 09, 2014: For ten days, 74 scientists and tourists were trapped in the Antarctic on board the Russian Akademik Shokalskiy research vessel. Strong winds had driven ice floes into a bay, blocking the ship's advancement. High-resolution satellite data of TerraSAR-X provided by DLR (German Aerospace Center) helped to assess the ice conditions at the location. 45)In pack ice, the situation can change quickly when the wind shifts. This is why researchers from the DLR Earth Observation Center (EOC) use up-to-date, high-resolution images from the Earth observation satellite TerraSAR-X to provide the crew of the research vessel with up-to-date information regarding the ice conditions. The German radar satellite operates in a variety of modes to permit imaging with varying swath widths, resolutions and polarizations.
- Seeing through clouds and darkness, the satellite is able to observe the ocean and frozen waters from an altitude of around 500 km, providing a swath width of 30 km. To do this, it emits microwaves that are reflected back to the satellite in a way that depends on the characteristics of the reflecting surface. The technology provides an extremely high-resolution image of down to 3 m. This is crucial, as the ice structure may change greatly over just a few hundred meters. Faced with the situation of the Akademik Shokalskiy, the DLR ground station processed the satellite images in near real-time and transmitted them to the rescue centre in Australia just one hour after the acquisition of the Antarctic scenes. Scientists from the DLR Microwaves and Radar Institute (IMF) used TerraSAR–X to acquire images of the trapped research ship on 1 January 2014. Software at the DLR Research Center for Maritime Safety in Bremen was used to track the ships, by utilising the contrast and differing textures of the vessel and sea ice to detecting the vessels amongst the frozen masses.
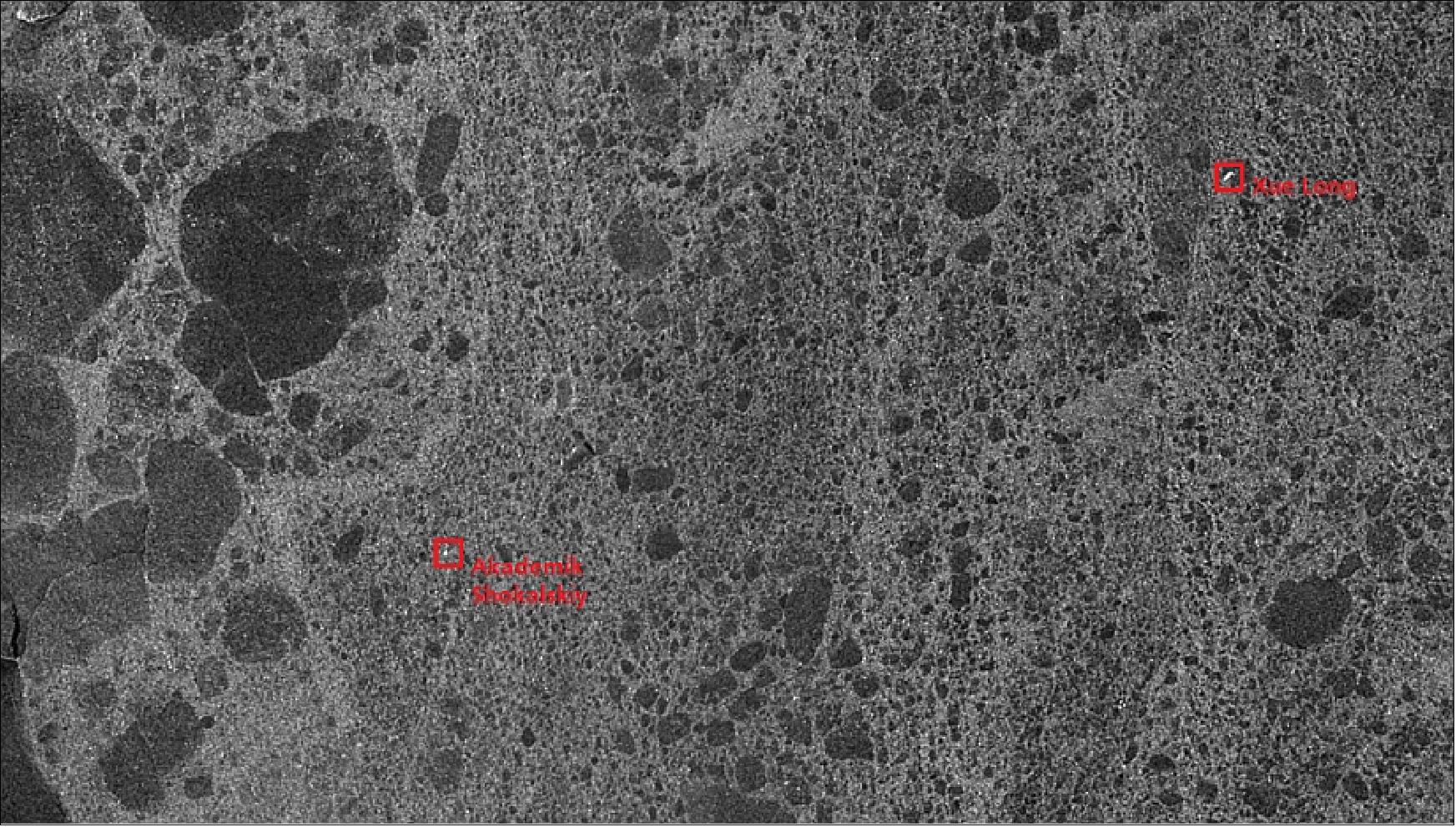
• October 2013: To comply with increased requirements on data freshness, especially from the MERS (Maritime and Emergency Response Services) segments, Astrium Geo-Information Services / Infoterra GmbH has constantly been upgrading TerraSAR’s ground station network access. As a benefit, especially through improved polar station access and processing capabilities, NRT (Near-Real-Time) delivery requirements can be served since early 2012. 46)In 2013, the product portfolio for TerraSAR-X was enhanced with two new operational modes for the user community: 47) 48) 49) ST (Staring Spotlight) mode, available since October 15, 2013, with resolution: 0.25 m (azimuth) x 0.8 m to 1.77 m (range); scene size: 2.1 to 2.7 km (azimuth), 7.5 to 4.6 km (range); single polarization: (HH, VV); SCW (ScanSAR Wide) mode, available since July 14, 2013, with resolution: 40 m (azimuth) x 6 to 10 m (range); scene size: 200 km (azimuth x 194 to 266 km (range); single polarization (HH, VV, HV/VH). The new TerraSAR-X Wide ScanSAR mode (SCW) provides an overview of an area of up to 400,000 km2 within a single acquisition - anywhere and independent of weather conditions. Wide ScanSAR data is thus ideally suited for monitoring ship traffic, detection of oil spills, and monitoring of maritime assets and sea ice, and contributing to the security, safety and efficiency of maritime activities around the globe.
- TerraSAR-X standard HR product uses the High-Resolution Spotlight mode with 300 MHz chirp bandwidth and offers an azimuth resolution of 1.1 m at 5 km azimuth scene extension with variable ground range resolution as a function of incidence angle at 10 km ground range scene extension. A so-called sliding Spotlight mode is used to generate this product. This results in a fairly good azimuth scene extension and equally distributed SNR across the image while grating lobes are reduced to a minimum to achieve the best possible ambiguity performance. The system is, however, capable of an improved azimuth resolution by applying the so-called ST (Staring Spotlight) mode operationally available as of fall 2013. This provides the best possible azimuth resolution (0.2 m, 1 look) using a much greater azimuth steering angle range from ±2.2º compared to the sliding Spotlight mode. It has been shown that the azimuth ambiguities that occur because of wide azimuth beam steering can be controlled by proper timing commanding. the azimuth scene extension increases with incidence angle, in contrast to the sliding Spotlight mode with constant azimuth scene extension. Different from Spotlight operations the Stripmap modes apply a SAR antenna beam that is always orthogonal to the flight direction.
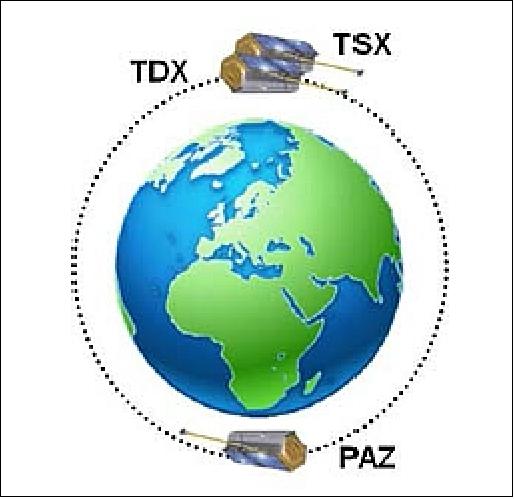
• August 30, 2013: With a spacecraft design life of 5 years, TerraSAR-X should have been out of service for over a year and a half (launch on June 15, 2007). However, engineers at DLR switched the satellite to yet another mode: TerraSAR-X could now record image strips over 200 km wide (in ScanSAR Wide mode, also referred to as SCW). The satellite does so by sweeping this large area in multiple stages, very quickly pivoting the radar beam numerous times across the direction of flight.
- TerraSAR-X had already delivered more than 120,000 images since being launched. However, the image strips from the TerraSAR-X satellite have been limited to a width of 100 km so far. For the first time, DLR is able to acquire an image of the entire German Bight from east to west, at a single point in time and in high resolution. The wide-swath radar imagery is providing the oceanographer with a great deal of information on the tidal flat and associated inlets between individual islands and the coast, as well as on the high water level in the Elbe estuary and near the island of Sylt. The operational condition of the TerraSAR-X spacecraft and its payload is still very good and the fuel reserves should enable the mission to continue operating until at least 2015
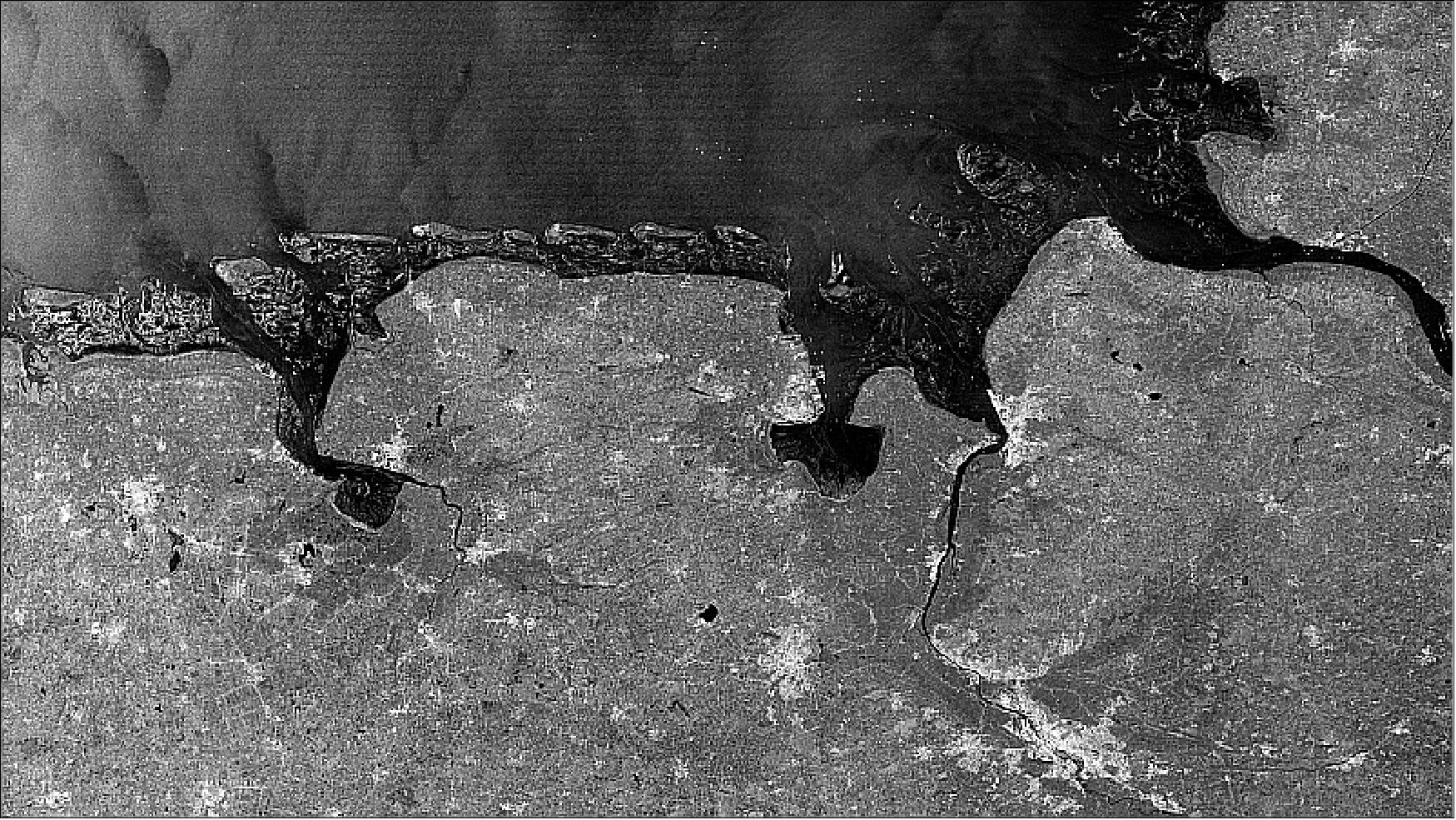
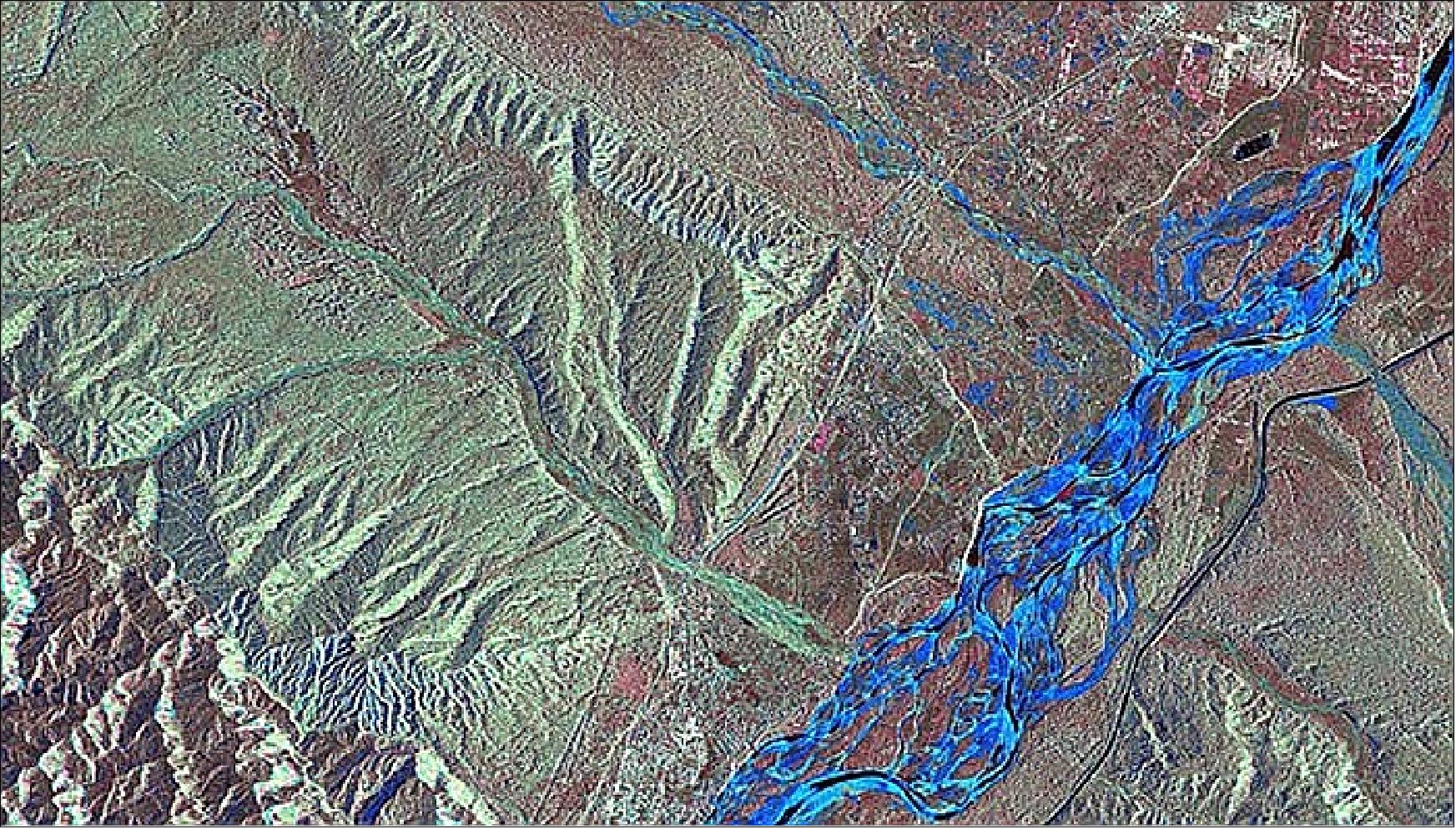
• The TerraSAR-X spacecraft and its payload were operating nominally in 2013.
• November 2012: Since January 2011, the Earth under the Santorini volcano has been stirring. Most of the time, it is barely noticeable, but every now and then the inhabitants notice small tremors jolting the volcanic archipelago. Nearly circular, and seemingly carved from stone, the submerged caldera is located in the Aegean Sea (Mediterranean, Greece). 53)Funded by the UK National Environment Research Council, radar specialist Juliet Biggs, Parks and volcanologist David Pyle (University of Oxford) began to study the Santorini volcano closely. Using GPS receivers, they determined precise locations with millimetric accuracy on a daily basis. The TerraSAR-X radar satellite also observed the archipelago from orbit, at an altitude of 514 km, recording its uplift and expansion from one orbit to the next. The results showed that the Kameni islands had risen 8 to 14 cm in many places. The breadth of the caldera as a whole has increased by about 14 cm since early 2011.
- In the analysis of the radar data, the red and yellow shading shows the areas where the ground has risen the most. The main island of Thira is unaffected by the deformation, thus appearing blue. The researchers believe that a magma chamber has formed at a depth of 4 km. They must now combine the information on the volcano's present behaviour with the knowledge of previous eruptions. The TerraSAR-X radar satellite has provided important information in this regard.

• October 2012: Figure 29 is the TerraSAR-X image of the month. 54)The image was acquired on June 23, 2009. The scene measures 50 km x 30 km. TerraSAR-X also cast a penetrating eye on the around 2000 m high mountains and the salt lake, which lies at an altitude of some 1270 m. The image shows rough surfaces in orange and smooth ones in grey/black. - The large, black surface bordering the industrial area in the middle of the image is the Wendover Facility. The large-scale industrial extraction of brine takes place here, which is needed for manufacturing potash.
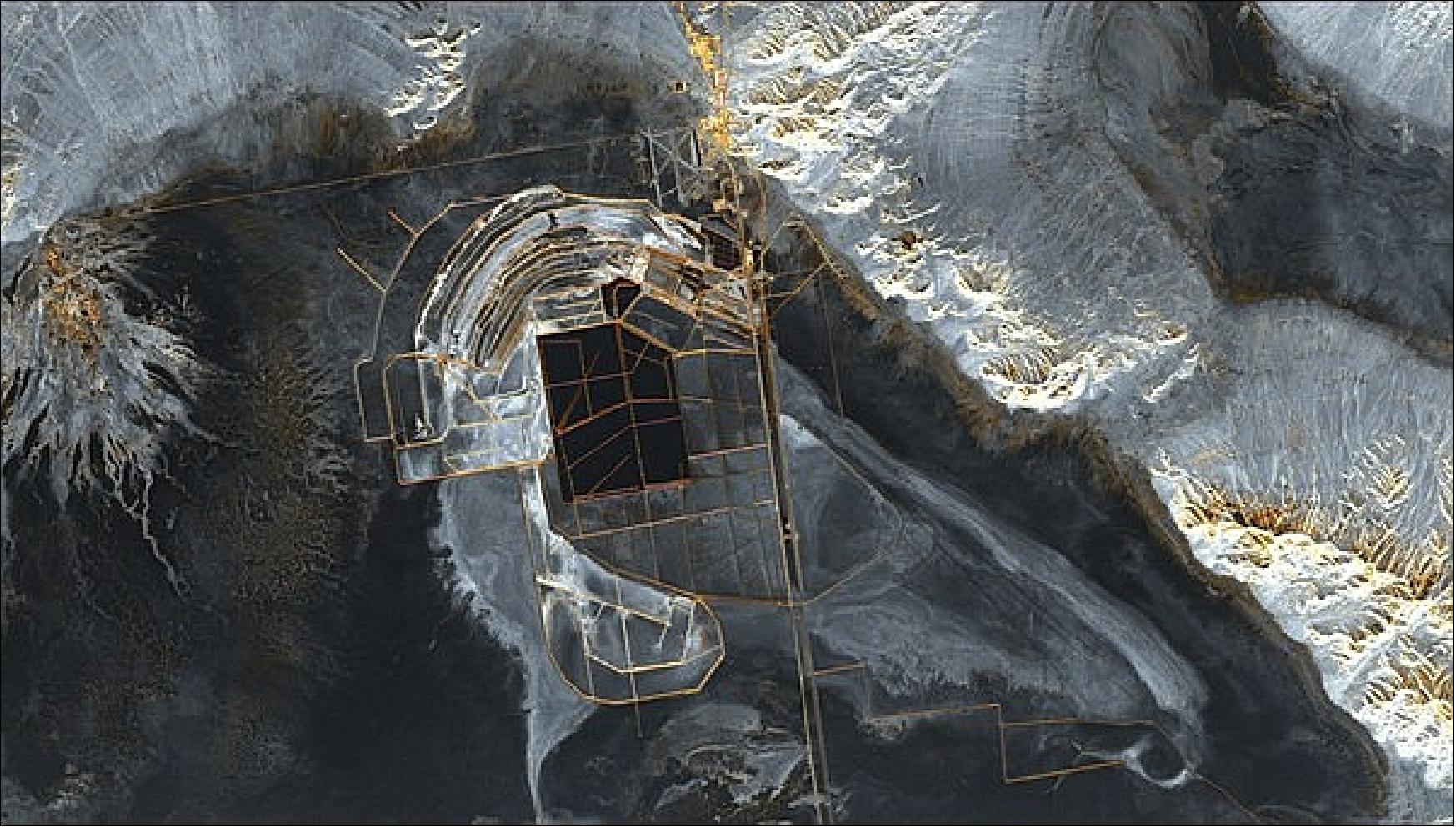
• June 15, 2012, the TerraSAR-X mission completed its 5th year on orbit. Designed to operate for five years, the satellite had now completed its nominal service life but it remains in excellent condition; it is expected to continue functioning for several more years. 55) The TerraSAR-X satellite mission has successfully supported or enabled a wide range of relief efforts and projects. Since June 2010, the satellite has been in good company; TerraSAR-X has been orbiting the Earth in close formation with its almost identical twin, TanDEM-X. Together, they are creating a highly accurate digital elevation model of Earth. With its own unchanged mission targets still in focus, TerraSAR-X has been meeting all expectations here as well (Ref. 55).
• January 2012: The TerraSAR-X satellite was fully operational and continuous its close formation flight with the TanDEM-X spacecraft. After a year of formation flight of TerraSAR-X, with TanDEM-X, the twin satellites had completely mapped the entire land surface of Earth for the first time. The data is being used to create the world's first single-source, high-precision, 3D digital elevation model of Earth. DLR controls both radar satellites, generates the elevation model, and is responsible for the scientific use of TanDEM-X data. 56)
- Ice and snow can be colourful - when observed by TerraSAR-X. The radar signals are able to penetrate the snow cover to a depth of one ~ 1 meter – and the subsurface reflects the pulse in different ways. This makes the frozen delta of the Mackenzie River in Canada appear multi-coloured in an image revealing the various structures in the landscape underneath the snow. 57)The colourful winter landscape serves a particular purpose for researchers, as they can use various images of the same region to track movement – when the river landscape freezes, when the ice sheet begins to break up again and when the thaw begins. The duration and intensity of this icy period are important indicators for climate research.
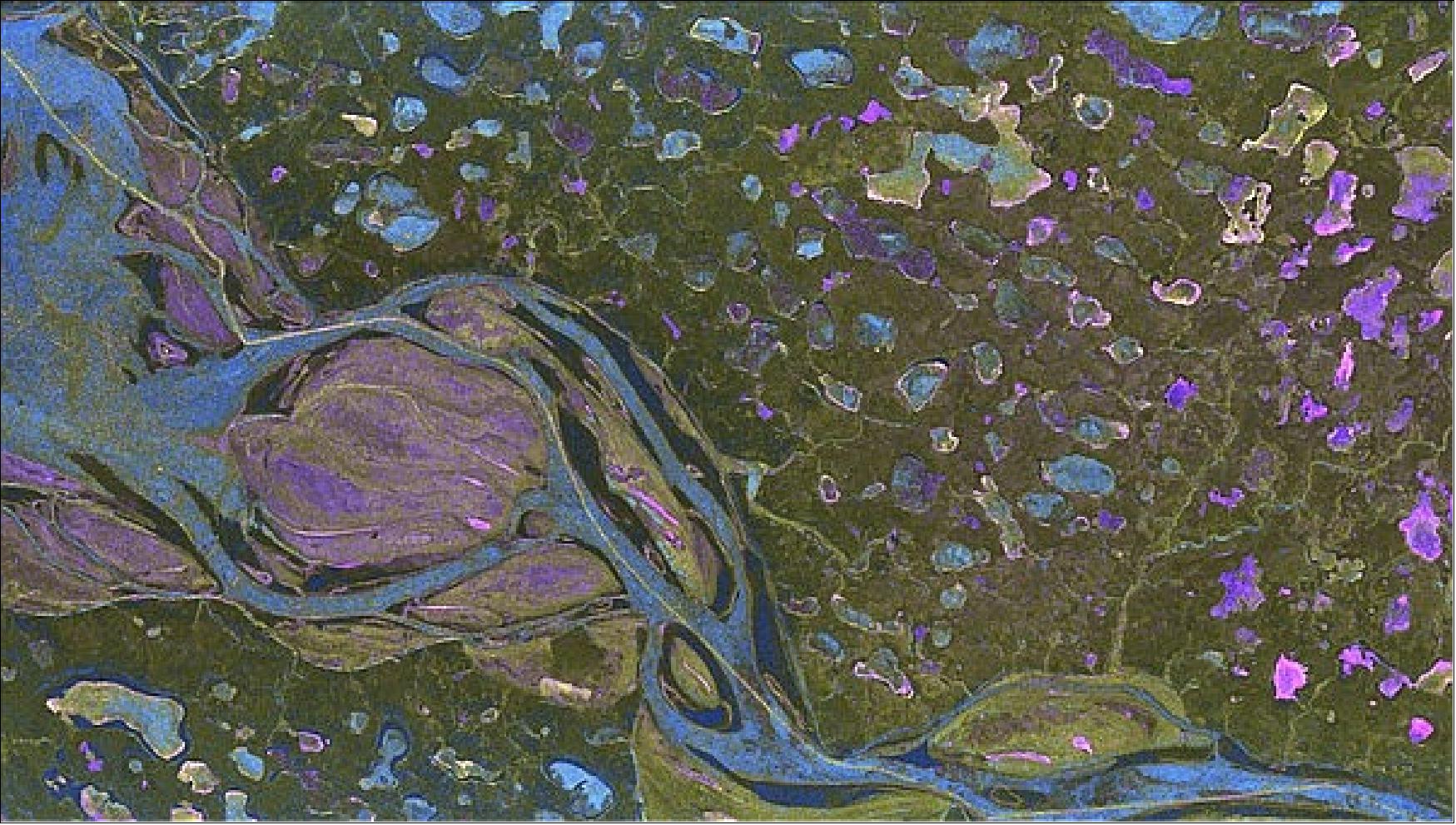
• 2011: DInSAR (Differential SAR Interferometry) study. TOPS (Terrain Observation with Progressive Scan) data was used in the processing chain to measure ground displacement movements by means of DInSAR. The investigation analyzed a stack of 8 TOPS and 8 stripmap images in terms of time-series performance for subsidence estimation. The estimated deformation used the SBAS (Small BAseline Subset) technique, which takes into account possible DEM errors and the APS (Atmospheric Phase Screen), have shown a good agreement between the TOPS and stripmap results. The investigation of the deformation using TOPS-stripmap cross-interferograms has also been performed and successfully exploited by means of CS (Coherent Scatterers). The results yield that, as expected, the phase is preserved for CS even if there is no spectral overlap. 58)
• January 2011, TerraSAR-X is fully operational and in close formation flight with the TanDEM-X spacecraft. The two spacecraft provide a single-pass interferometric configuration, which was declared operational in December 2010. The collection of data for a global homogeneous DEM started - as planned - in early 2011.Figure 31 is the image of the month of July 2011 of TerraSAR-X. The Puyehue volcano erupted on June 4, 2011, in the southern Andes mountains. A field of lava, appearing as a uniform, light blue surface, is currently forming there. Radar images acquired by TerraSAR-X have been providing valuable information to the staff of the Chilean Volcano Risk Program since the eruption began, helping them to assess the situation and predict its future development. 59)
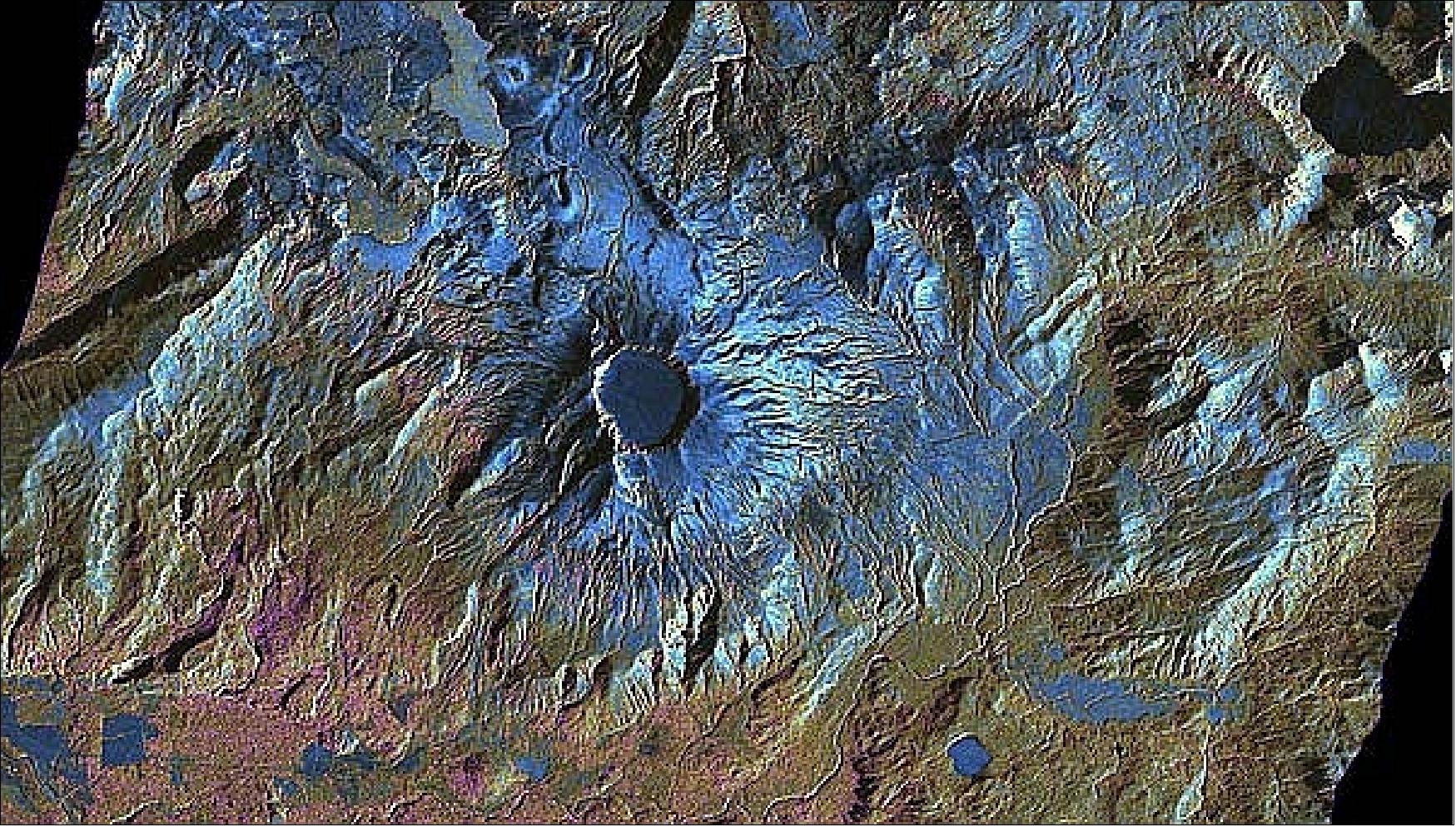

• The TerraSAR-X spacecraft and its payload were operating nominally as of 2010.
• January 2010: Imagery of the TerraSAR-X spacecraft as well as optical imagery from other spacecraft was being used by a DLR/DFD analysis team to support the disaster relief activities of the devastating earthquake that hit Haiti on Jan. 12, 2010. Satellite-based maps were generated of the stricken region and provided to the relief organisations via the internet. In the absence of any or very little information, the current state of the infrastructure in the capital city of Port-au-Prince was of great service to the relief workers. The quickly generated reference maps were giving an overview of the road network as well as of important buildings and facilities such as the airport as they were before and after the earthquake. 61)
• October/November 2009: high-resolution TerraSAR-X data were acquired in Antarctica in the left-looking observation mode. The areas of scientific interest were located within glacier basins and ice streams that flow through the Transantarctic Mountains and into the Ross Ice Shelf. Detailed ice velocity patterns on the Nimrod glacier basin and Starshot glacier were studied. 62)
• Summer 2009: two years after launch, two dedicated calibration campaigns were performed, one for the re-calibration of TerraSAR-X, and one for the experimental DRA (Dual Receive Antenna) mode. The effective and exact calibration techniques already successfully applied for the commissioning of TerraSAR-X in 2007 have shown once again how accurately the complex TerraSAR-X system can be adjusted. Moreover, by deriving the stability of the whole SAR system by real measurements two years after launch, the accuracy could be improved further on. - In total, 40 campaigns against reference targets and about 150 acquisitions across the Amazon rainforest were successfully executed and evaluated. The stability and the accuracy of the whole TerraSAR-X system and especially the radar instrument itself is still of unprecedented quality. 63)
• 2009: the measurement of ground object motions with the SAR ATI (Along-Track Interferometry) data acquisition capability was demonstrated from a spaceborne SAR instrument in different contexts, two typical applications are traffic flows and water surface currents. 64) 65)
- With regard to traffic flow, the focus lies on detecting a number of small (compared to the image resolution) separate moving objects to derive traffic information for a whole area or larger sections of a road network from it. With its large-area data acquisition and weather and daylight independence, SAR offers great potential to augment existing networks of traffic sensors or sometimes to be the only source of traffic data. This TTP (TerraSAR-X Traffic Processor) includes SAR focusing, vehicle detection and measurement for public roads as well as the generation of an easily distributable traffic data product. Road data are extracted from a database for the processed scene and enable to restrict processing to only relevant image areas, to enhance moving object signatures by adaptive filtering of the SAR data and to provide velocity measurements for detected objects based on azimuth displacement.
•Spring 2009: The TerraSAR-X spacecraft and its payload are operating nominally in 2009. 66) 67) 68) 69)Spacecraft and ground segment are fully operational. Image products (Spotlight, Stripmap, ScanSAR) are calibrated and released. The product quality is within initial specification or better. Operation of the SAR instrument proved to be very stable. Demonstrations accomplished: Repeat pass interferometry, along-track interferometry, persistent scatterer evaluation, TOPSAR (while TOPSAR was demonstrated, implementation is pending), total zero Doppler steering, and demonstration of quadpol mode. Demonstration of quadpol mode. The use of TerraSAR-X data was demonstrated for geoscientific applications, oceanography and disaster monitoring during the commissioning phase.
• February 16, 2009: TOR (Tracking, Occultation and Ranging) payload radio occultation measurements were activated permanently onboard TerraSAR-X. The IGOR receiver of TOR was powered up shortly shortly after deployment of the TerraSAR-X mission delivering continuous tracking data for POD (Precise Orbit Determination) support. 75) 76) The radio occultation measurements (TOR-RO) were enabled for a test period of 4 weeks from January 15 through February 15, 2008. This campaign yielded a daily total of about 250 neutral atmospheric profiles for temperature and humidity as well as additional ionospheric data of the vertical electron density distribution. SLR measurements tracking the TerraSAR-X spacecraft are being regularly performed by the global SLR community. The ranging campaign yielded a total of 735 laser passes for a period between June 16, 2007, and Oct. 1, 2007. Single shot accuracies of 3-4 cm are being reported from the specially equipped ground station at Graz, Austria.
• January/February 2008: LCTSX (LCT on TerraSAR-X) FSO (Free Space Optics) communication demonstrations: In a series of ISL (Intersatellite Link) tests, the LCT (Laser Communication Terminal) on TerraSAR-X as well as the one flown on the DoD NFIRE (Near Field Infrared Experiment) spacecraft, have exchanged data simultaneously at rates of 5.625 Gbit/s ((equivalent to ~200,000 A4-pages per second). According to Tesat-Spacecom GmbH, it has taken < 25 seconds, on average, for the terminals to lock onto each other and begin transmissions. A key feature of the system is its ability to establish and maintain a link, even when the sun is directly behind the target spacecraft. 70) 71) 72) 73) 74)
- The first LEO-LEO inter-satellite link was performed above the Pacific Ocean near Central America. Continuous free-space optical transmissions were maintained for as long as the two spacecraft were within the line-of-sight position of each other (both spacecraft in LEO) amounting to about 20 minutes on an average pass. On these free-space transmissions, the measured BER (Bit Error Rate) was < 10-9. Since the NFIRE spacecraft is not producing its own imagery, a closed-loop link was configured where the data from TerraSAR-X was directly re-transmitted from NFIRE to establish “duplex operations” at 5.625 Gbit/s. - Further tests are planned with transmissions to ground stations in Germany and in Spain.
- The orbits of the two LEO satellites (TerraSAR-X and NFIRE) propagated in opposite directions to each other. This required the LCT to track its counter terminal across an azimuth range of about 80º. The elevation range was about 10º. The link distance varied between 3,700 km and 4,700 km, with a maximum range rate of 8,500 m/s. As was verified in later experiments the pointing accuracy of the LCT allows closing spatial acquisition between NFIRE and TerraSAR-X significantly faster than 10 s. Frequency acquisition has been optimized to lock the phases within 20 s. The bit error rate was always better than 10-9
.• December 13, 2007: A successful ORR (Operational Readiness Review) of TerraSAR-X took place with all systems tested and validated - and with nominal operations of the spacecraft. The mission was officially declared operational on January 7, 2008. Scientists and engineers from DLR and EADS Astrium had spent the past few months calibrating and commissioning the satellite. 79) 80) 81) The results of calibrating TerraSAR-X approve the accuracy calculated before launch and put the described strategy to calibrate efficiently a multiple mode SAR system like TerraSAR-X on a solid base. All requirements and/or goals had been achieved for the project. 82) The SAR products were operationally released 5.5 months after launch. This was possible due to an intensive combined test program of the ground segment and the space segment. The CP (Commissioning Phase) planning tool allowed flexible planning and re-planning throughout the CP. Over 12000 DTs (Data Takes), i.e. scenes, were acquired and processed.
- The main objectives of the CP were the calibration and verification of the entire SAR system chain in order to achieve the specified SAR image and product quality as well as the operationalization and validation of the ground segment functions. This involved in particular the tuning and adjustment of the TMSP (TerraSAR-X Multi-Mode SAR Processor) to meet the in-orbit data characteristics and to optimize the SAR focusing results. The TMSP consistently generates phase-preserving SSC (Single-look Slant-range Complex) data sets from the imaging modes Stripmap, ScanSAR and Spotlight for all specified polarization modes. Derivation of multi-look detected products (MGD, GEC and EEC) is based on SSCs as an interim production stage 83)
• November 2007: A bistatic X-band experiment was successfully performed. TerraSAR-X (TSX) was used as the transmitter and DLR's new airborne radar system F-SAR, was programmed to acquire data in a quasi-continuous mode to avoid echo window synchronization issues. The F-SAR system was used as a bistatic receiver in this configuration. Precise phase and time referencing between both system was derived during the bistatic processing. The experiment was considered a success after data analysis. 77) 78)For the first time, a spaceborne-airborne X-band acquisition had been successfully conducted, including high-resolution SAR processing. The bistatic image shows an improved resolution, SNR and no range ambiguities, as well as a different perspective of the imaged scene.
• October/November 2007: Demonstration of the novel TOPSAR (Terrain Observation with Progressive Scan) SAR operations support mode concept on TerraSAR-X (on behalf of ESA). The TOPSAR technique employs a very simple counter-rotation of the radar beam in the opposite direction to a “spot observation”; hence, the name TOPS (Terrain Observation with Progressive Scan). The first TOPSAR images and interferometric results were demonstrated on the TerraSAR-X spacecraft during the commissioning phase in the fall of 2007. TerraSAR-X was able to provide this demonstration because its TSX-SAR instrument because it was able to electronically steer the antenna azimuth pattern. This capability, together with the high flexibility of the satellite commanding, provided the opportunity to implement on the satellite the TOPSAR acquisition mode. 84) 85)
- TOPSAR is an ESA-proposed acquisition mode for wide swath imaging which aims at reducing the drawbacks of the ScanSAR mode. The basic principle of TOPSAR is the shrinking of the azimuth antenna pattern (along-track direction) as seen by a target on ground. This is obtained by steering the antenna in the opposite direction as for Spotlight. The TOPSAR mode is intended to replace the conventional ScanSAR mode. The technique aims at achieving the same coverage and resolution as ScanSAR, but with a nearly uniform SNR (Signal-to-Noise Ratio) and DTAR (Distributed Target Ambiguity Ratio). 86) 87) 88)
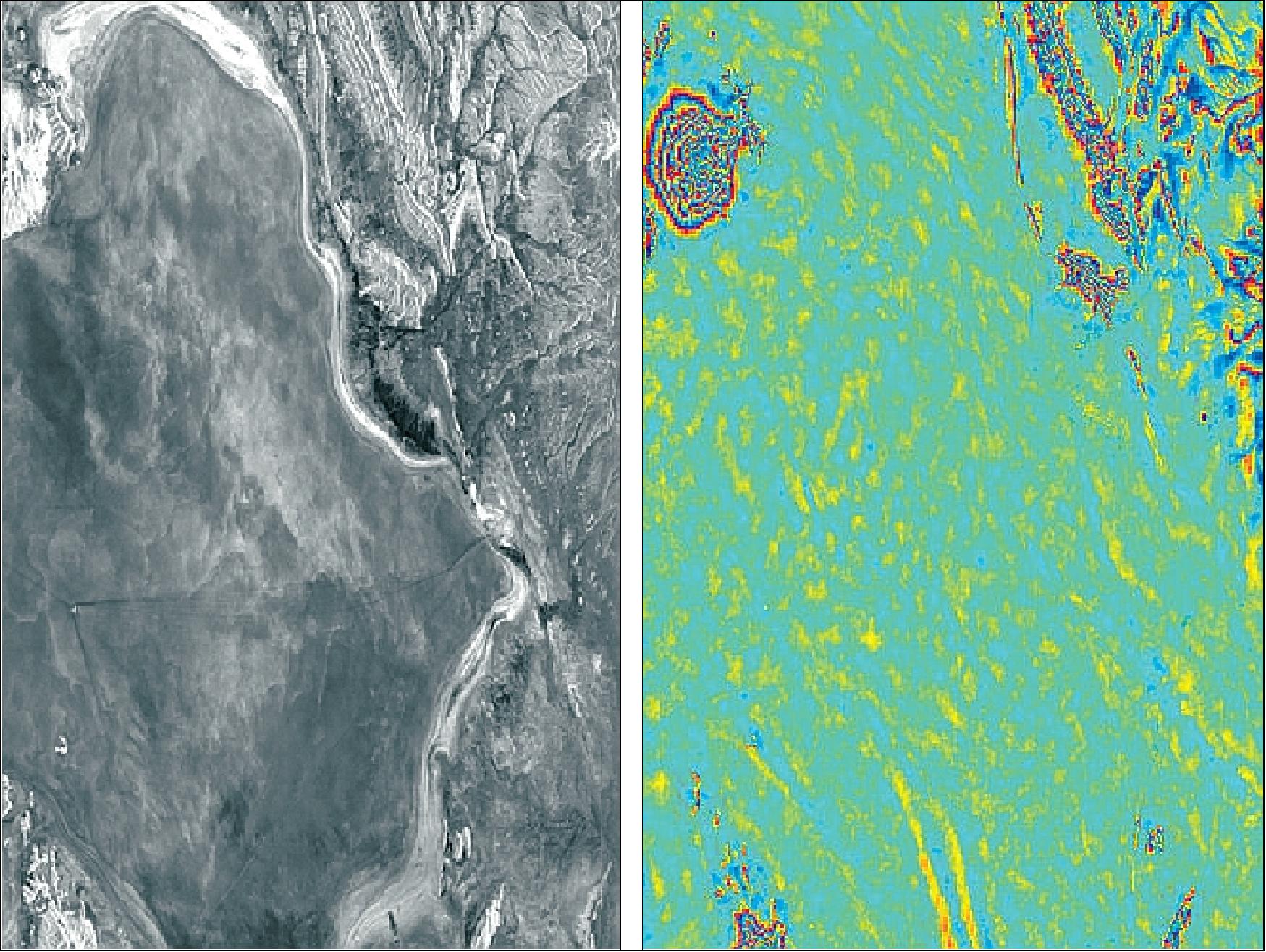
• June 19, 2007: Contact with the spacecraft was established shortly after launch. All systems were functioning nominally. All standard imaging modes (strip, spot & scan) were exercised in this early phase. Hence, LEOP (Launch and Early Orbit Phase) was successfully completed on June 21, 2007. Thereafter, TerraSAR-X was put into its commissioning phase; it was expected to remain in this status until the end of 2007. During this survey, a thick cloud cover prevailed. Nevertheless, radar satellites such as TerraSAR-X offer imaging capability even in the case of cloudy skies and at night. However, exceptional strong precipitation events like heavy thunderstorms may influence even radar imaging. Such an event can be seen at the upper left part of the radar image as a bright ”veil”.

Sensor Complement
TSX-SAR (TerraSAR-X SAR instrument). TSX-SAR is an active phased array X-band antenna system providing high-resolution and multi-polarization SAR imagery (H and V), permitting the operational modes of “stripmap,” “spotlight,” and “scanSAR.” The beam-forming capability and quality of the active phased array technology introduce a range of flexibility, permitting the acquisition of high-resolution imagery as well as wide-swath imagery. The active phased array front-end is structured in azimuth direction (along-track) into three antenna leafs, each comprised of four antenna panels. One antenna panel is made up of 32 active sub-arrays in elevation, each comprising an HP (Horizontal Polarization) and a VP (Vertical Polarization) slotted waveguide radiator. Each of the 384 sub-arrays (32 x 12) is equipped with a T/R (Transmit/Receive) module - also referred to as TRM. The dual-polarized waveguide radiator allows polarization selection via a polarization switch in the T/R module (TRM). In toggle mode, it can switch the polarization from pulse to pulse. This allows for the simultaneous acquisition of two image polarizations. 90) 91) 92) 93) 94) 95) 96) 97)
The front end is controlled by ACE (Antenna Control Electronics), providing programmable real-time control of the antenna beam shape, pointing and polarization in transmission, and reception. For each commanded antenna beam, one of 256 stored elevation beam configurations is combined with one of 256 azimuth beam configurations; the resulting excitation coefficients are transferred to the T/R modules. Beam steering in azimuth (± 0,75º) and elevation (± 20º) is performed by ACE, which provides programmable real-time control of antenna beam shape, pointing and polarization in transmission and reception. The switching of an antenna beam can take place at a maximum rate of 275 Hz. ACE is controlled by CE (Control Electronics), consisting of DCE (Data & Control Electronics), ICU (Instrument Control Unit) and RFE (Radio Frequency Electronics). RFE contains the USO (Ultra Stable Oscillator), the up- and down-conversion and preamplifying stages and provides programmable signals for internal calibration.
CE provides the following functions:
• Generation and transmission of the Tx signal
• Reception and A/D conversion of the Rx signal
• SAR data buffering, compression and formatting
• Instrument timing and control.
The transmit signal is produced in a digital chirp generator. An AWG (Arrayed Waveguide Grating) writes up to 8 different waveforms of commanded length and a bandwidth of up to 300 MHz in a waveform memory. One of these 8 waveforms is selected for each pulse and can be switched from pulse to pulse. In the receive path, one of three anti-aliasing filters which are matching to the ADC sampling rates of 110, 165 or 330 MHz, can be selected. The data are compressed online with a BAQ algorithm after the time extension buffering. The BAQ processing works on blocks of 128 consecutive samples with a selectable compression rate of 8 to 4, 3, or 2 bits per sample. A transparent mode also allows bypassing of data compression.


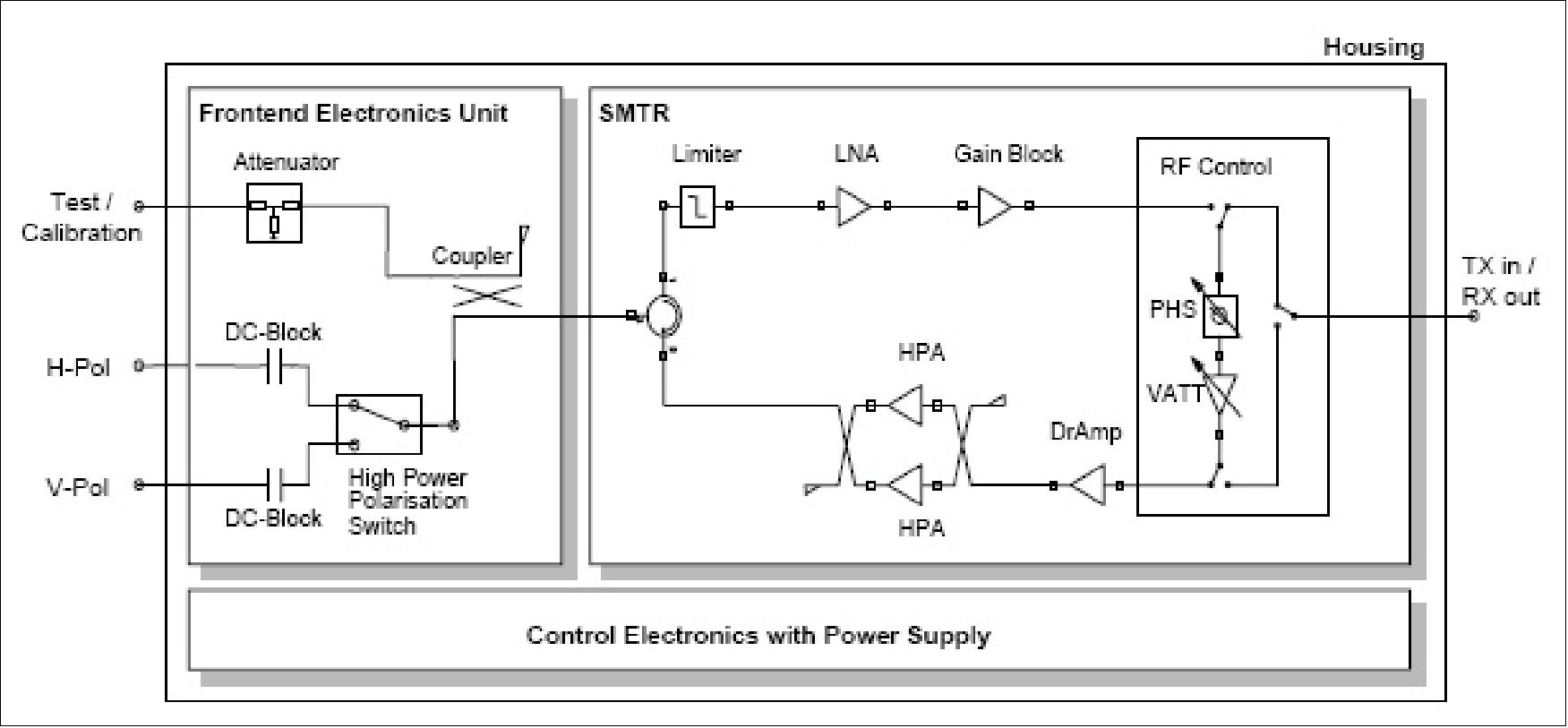
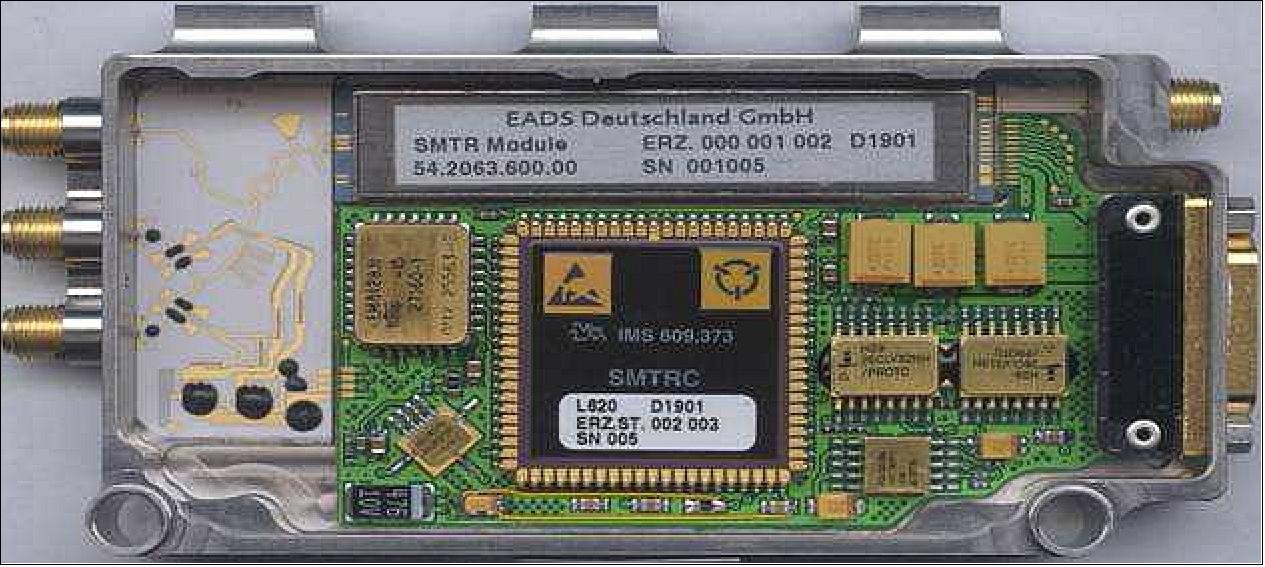
The TSX-SAR instrument elements are fully redundant, i.e. a main and a redundant functional chain exists. This feature makes it possible to activate both functional chains at the same time, one being the master for timing purposes, thus permitting operations in an experimental DRA (Dual Receive Antenna) mode where the echoes from the azimuth antenna halves can be received and then separated during ground processing, e.g. to serve the application of ATI (Along Track Interferometry). For ATI support the SAR antenna can be grouped into two segments, each of 2.4 m. 99) 100) 101)
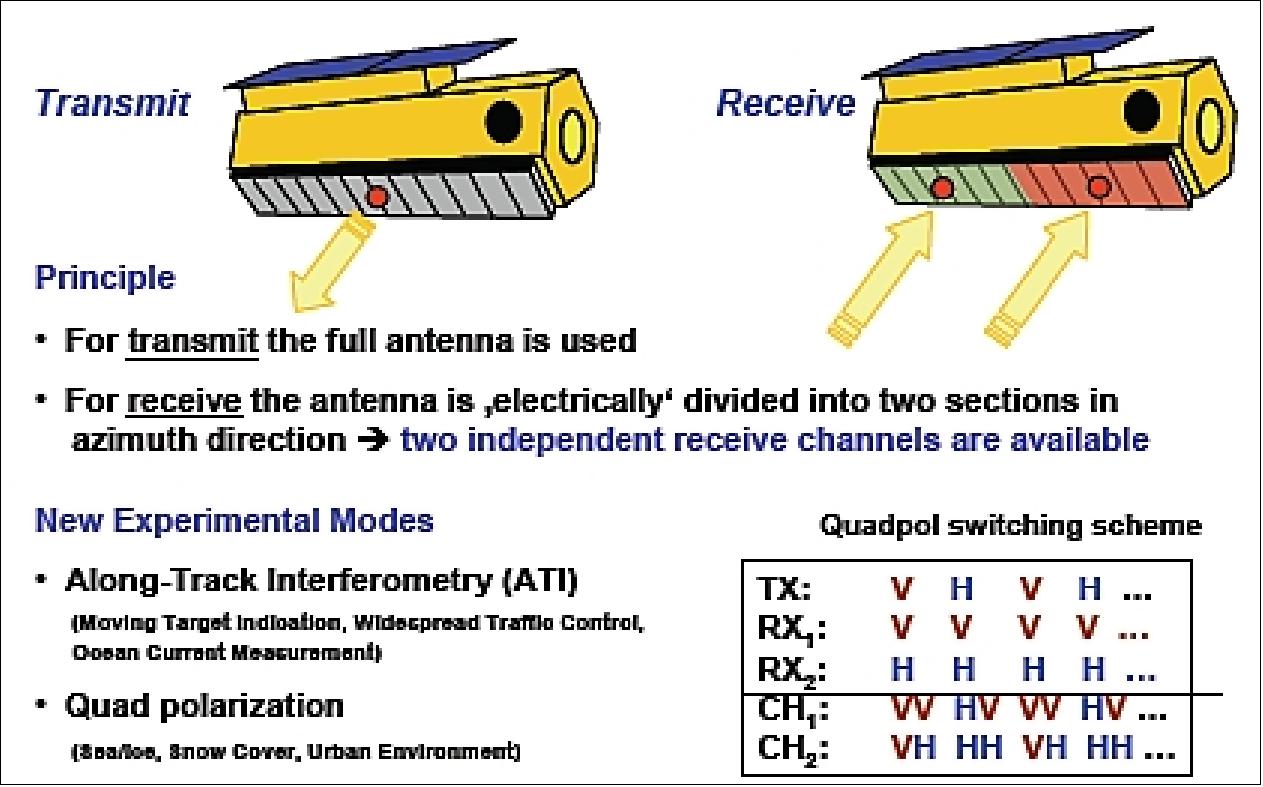
Due to the bistatic configuration (one transmitting antenna, two receiving antenna elements), the effective ATI baseline is half antenna separation, i.e. 1.2 m, which corresponds to a time lag of 0.17 ms. Ideal ATI time lags for oceanic current measurements at X-band should be on the order of a few milliseconds, i.e. about 20 times longer than this; thus, the sensitivity of TSX-SAR to small current variations will be quite low. The ATI feature permits the measurement of surface currents with a spatial resolution of about 1 to 2 km. 102)
Parameter | Value | Parameter | Value |
Antenna type | Active phased array | Beam scan angle range | ±0.75º (az), ±19.2º (el.) |
X-band center frequency | 9.65 GHz (3.1 cm wavelength) | Incidence angle access range | 15º - 60º |
Antenna aperture size | 4.8 m x 0.8 m x 0.15 m | Radiated peak power | 2260 W |
Phase centers | 12 (az.) x 32 (el.) | Stripmap duty cycle | 18% (on transmit) |
Polarization modes | HH/VV/HV/VH | Spotlight duty cycle | 20% (on transmit) |
System noise figure | 5.0 dB | Operational PRF range | 3000 - 6500 Hz |
Selectable BAQ compression rates | 8 to 4, 3, 2, by-pass | ADC sampling rates (8 bit, I&Q) | 110, 165, 330 MHz |
Max receive duty cycles | 100%, 67%, 33% | Chirp bandwidth range | 5 - 300 MHz |
SSMM (Solid State Mass Memory) capacity | 320 Gbit (BOL), | TSX-SAR instrument mass | 394 kg |
Quantization of signal | 8 bit I, 8 bit Q | SAR data compression | online BAQ |
Nominal look direction | Right side of groundtrack | Yaw steering | Yes |
TSX-SAR provides a variety of Spotlight, Stripmap and ScanSAR image products. The full operator access to the active phased array antenna together with the 300 MHz modes allows for a large number of custom-designed high-performance image products. 103)
The spotlight mode is realized on a “sliding spotlight operation” concept. 104) Compared to the starring spotlight operation, the sliding spotlight has the advantage of more uniform NESZ (Noise Equivalent Sigma Zero) performance achieved in the along-track direction due to the averaging of the gain variation of the main beam in azimuth. The signal bandwidth is maintained constant for the whole incidence angle range in order to provide a constant slant range resolution.
Parameter/Operational mode | Spotlight | Spotlight | Experimental Spotlight | Stripmap mode (SM) | ScanSAR mode (SC) |
Resolution, cross-track | 2 m | 2 m | 1 m | 3 m | 16 m |
Product coverage, (km) |
|
|
|
|
|
Access range of incidence angles (full performance) | 20-55º | 20-55º | 20-55º | 20-45º | 20-45º |
Access range of incidence angles (data collection) | 15-60º | 15-60º | 15-60º | 15-60º | 20-60º |
Sensitivity NESZ - typical |
-23 dB |
-23 dB |
-20 dB |
-22 dB |
-21 dB |
DTAR ambiguity ratio | < -17 dB | < -17 dB | < -17 dB | < -17 dB | < -17 dB |
Source data rate, (8/4 BAQ) | 340 Mbit/s | 340 Mbit/s | 680 Mbit/s | 580 Mbit/s | 580 Mbit/s |
The primary image performance parameters, provided in Table 7, are NESZ (Noise Equivalent Sigma Zero) and DTAR (Distributed Target Signal to Ambiguity Ratio). They show performance variations within the image as well as over the incidence angle range. NESZ is that value of sigma nought (σo) of a uniform scene that produces a processor output signal to noise of unity. The “access range” is the cross-track accessible (viewable) range on the ground provided by electronic beam steering. The instantaneous range of TSX-SAR is e.g. 30 km (max) in stripmap mode and 100 km for ScanSAR mode. In Stripmap operation, the synthetic aperture is typically given by the -3dB beamwidth of the antenna azimuth pattern defining the processed Doppler bandwidth. In order to provide sufficient performance swath width near the nadir, a broadening of the elevation beamwidth is needed. This is achieved by amplitude/phase tapering of the active antenna. 105)
TSX-SAR Operations and Support Modes
• TSX-SAR is capable to image on either side of the subsatellite track, this is accomplished by a roll maneuver of the spacecraft. The S/C roll feature extends the FOR (Field of Regard) of the instrument for possible event coverage. However, observations to the right side of the subsatellite track are considered to be the preferred (and default) operations mode, due to power constraints in the left-side configuration (the solar array isn't pointing into the sun anymore; also, the communications link to the ground is obstructed).
• There are also experimental dual-receive modes for wide bandwidth (300 MHz), providing even higher resolution, as well as for full polarization and along-track interferometry (ATI), the latter two being achieved by splitting the receive antenna into two azimuth halves (split-antenna stripmap mode). The dual-receive mode provides the potential for ATI velocity measurements of ocean currents, and a full polarimetric mode, by receiving simultaneously the H and V components with the two apertures (the dual-receive mode is also being used to demonstrate traffic velocity measurements on highways). The redundancy concept in the TerraSAR-X receiving chain and the front-end design offers the possibility to use the second spare receiving channel in parallel to the main receiving channel.
• Capability of repeat-pass as well as Along Track Interferometry (ATI)
• Support of four imaging modes: Stripmap, ScanSAR, High-resolution Spotlight, and Spotlight 106) 107) 108)
- SM (Stripmap Mode). The ground swath is illuminated with a continuous sequence of pulses while the antenna beam is fixed in elevation and azimuth. This results in an image strip with continuous image quality in azimuth.
- SC (ScanSAR Mode). SC mode provides a large area coverage. The wider swath is achieved by scanning several adjacent ground sub-swaths with simultaneous beams, each with a different incidence angle. Due to the reduced azimuth bandwidth, the azimuth resolution of a ScanSAR product is lower than in StripMap mode.

- HS (High-resolution Spotlight Mode). HS provides the highest geometrical resolution. Therefore the size of the observed area on the ground is smaller than the one in all other modes. During the observation of a particular ground scene, the radar beam is steered like a spotlight so that the area of interest is illuminated longer and hence the synthetic aperture becomes larger. The Maximum azimuth steering angle range is ± 0.75º.
- SL (Spotlight Mode). The HS and SL modes are very similar. In SL mode the geometric azimuth resolution is reduced in order to increase the azimuth scene coverage.
• The instrument features selectable or dual polarization (support of single, dual and full polarization modes). Further flexibility is provided by a large number of possible antenna beam configurations. The number of beams in elevation for SM or SC support is about 12. The number of beams in elevation for HS or SL support is about 95.
- Single polarization:
The radar transmits either H or V-polarized pulses and receives in H or V polarization. The resulting product will consist of one polarimetric channel in one of the combinations of HH, HV, VH or VV. It can be operated in all different modes HS, SL, SM and SC.
- Dual polarization:
In this mode, the radar toggles the transmit and/or receive polarization on a pulse-to-pulse basis. The effective PRF in each polarimetric channel is half of the total PRF, which means that the azimuth resolution is slightly reduced. The polarimetric phase between both channels can be exploited, e.g. for interferometry or classification purposes. The product consists of two layers that can be selected out of the possible combinations. Dual polarization is possible for all image modes as well.
- Quad polarization:
Quad polarization is possible in the experimental dual receive antenna mode as the signal can be received simultaneously in H and V polarization. By sending alternating H and V pulses, the full polarimetric matrix can be obtained. Currently, quad-polarization is not operationally foreseen (only research support).
• Left or right side observation capability. The slew capability of the TerraSAR-X spacecraft allows observations to be conducted on either side of the sub-satellite track. This feature is of great value for event monitoring - doubling in effect the FOR (Field of Regard).
TSX-SAR Instrument Calibration
The calibration process transforms the image magnitude or power into the required physical units, which are assumed to be those of radar cross section (RCS) or back-scattering coefficient σo (sigma-zero, radar cross section per unit illuminated area). Three major tasks are being performed by the calibration: 109) 110) 111) 112) 113) 114)
- Internal calibration:
The compensation of instrument fluctuations. This is performed by in-orbit verification of the instrument against pre-flight results. This internal calibration yields a stabilized radar instrument and defines the radiometric stability.
- Antenna pattern calibration:
The compensation of the antenna pattern within the SAR scene in order to obtain a constant gain across the whole SAR image. This is performed by a determination/estimation of the actual antenna pattern. We thus obtain relatively calibrated SAR data products and the relative radiometric accuracy.
- External calibration:
The correction of the radiometric bias. This is performed by measuring the radar system against standard ground targets with known radar cross-section (RCS). This external calibration yields an absolutely calibrated radar system and defines the absolute radiometric accuracy.
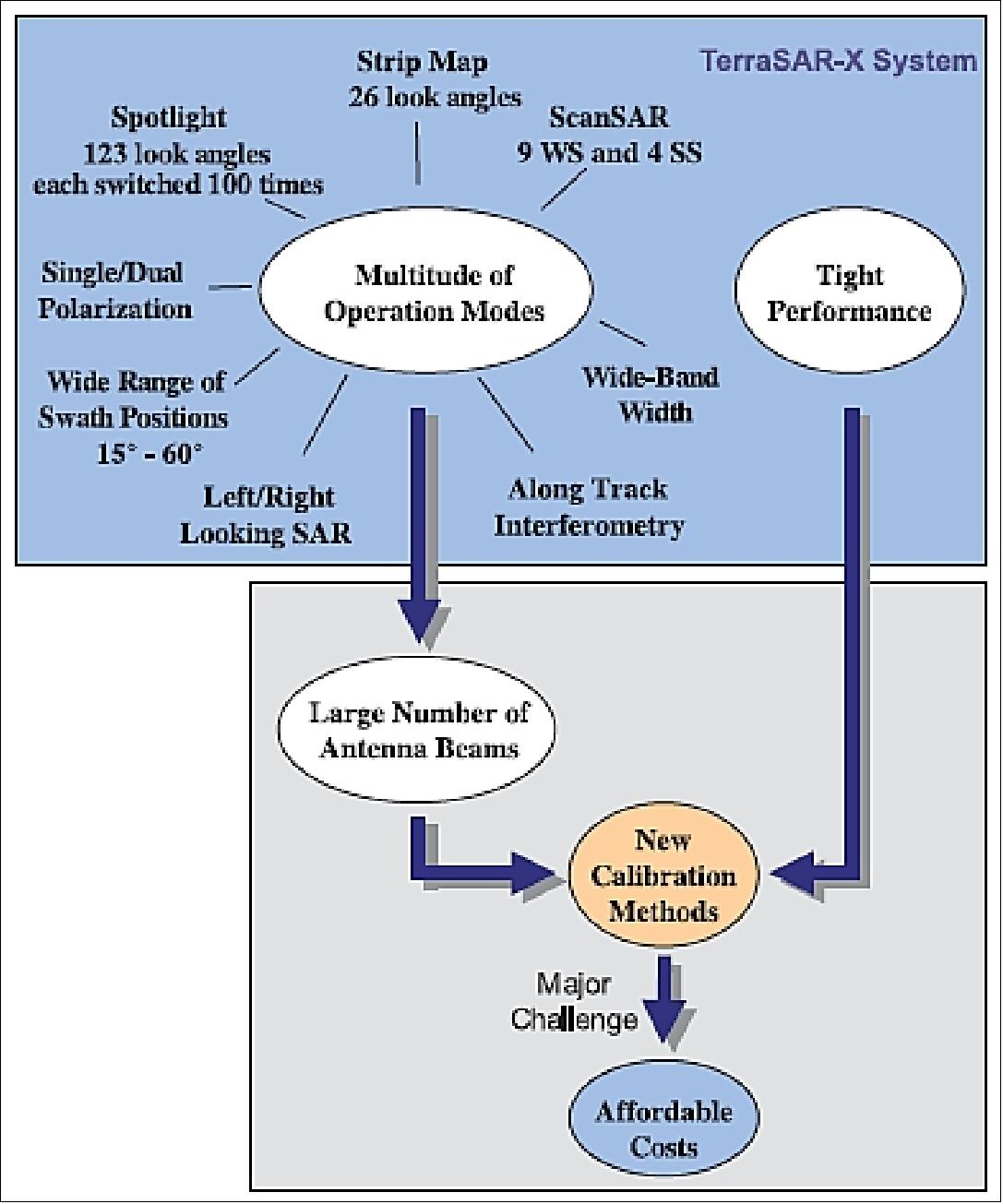
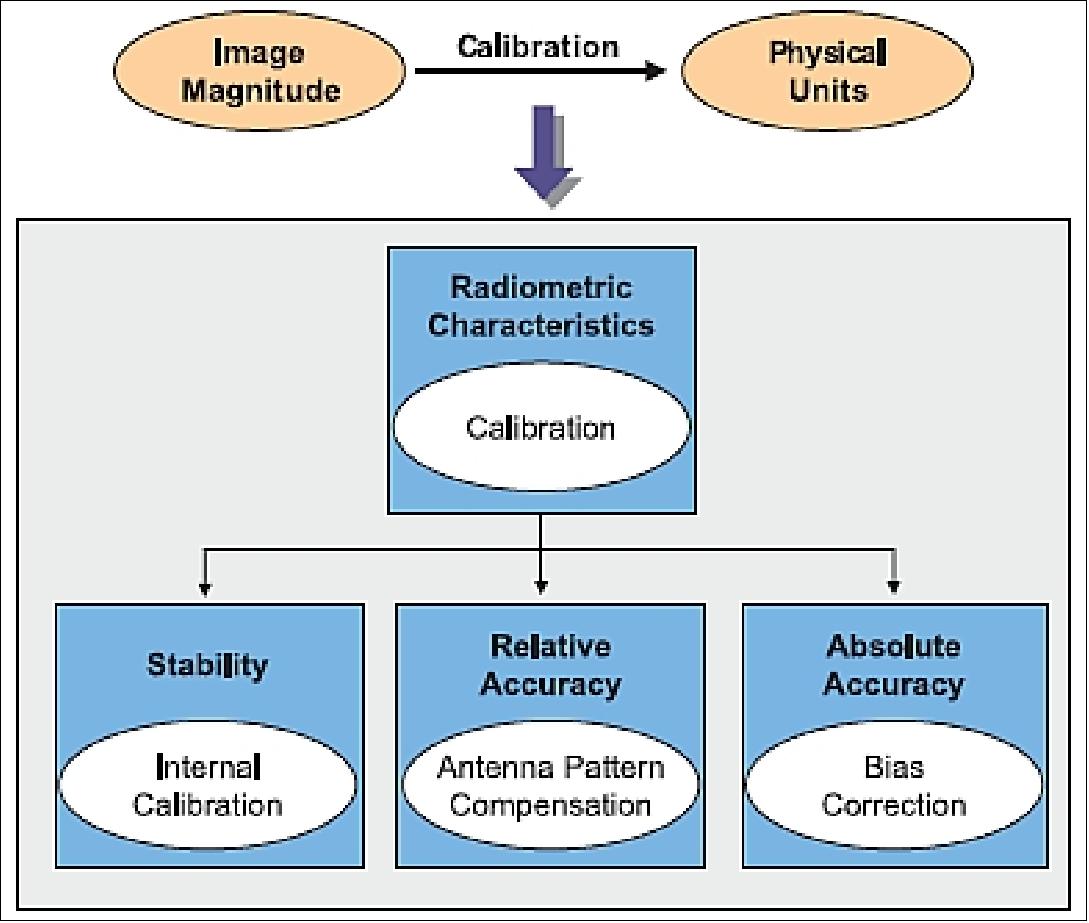
The internal calibration facility of TSX-SAR monitors the critical elements of the XFE (X-band Front-end), consisting of the 384 T/R modules (TRMs) of the active phased array. Each module is feeding a radiating sub-array for horizontal and vertical polarization - controlling the beam steering in azimuth and elevation direction. Three different types of calibration pulses are applied, whereby sets of these pulses are needed at the start and end of each data take. All calibration pulses have the same length and bandwidth (chirp) as is commanded for the mode.
Precise modelling of the antenna is only possible if the actual characteristics of each individual transmit/receive module (TRM) are known. A calibration network (CAL N/W) records the internal instrument behaviour characterizing the instrument stability over time. The antenna performance can be monitored with an innovative characterization mode based on the so-called PN-gating method. 115)

The essential TSX-SAR calibration facilities/references are:
• Standard ground target monitoring for the bias correction
• Ground receivers for in-flight measurements
• Different analysis and evaluation tools
- The antenna pattern model provides an estimation of the actual antenna pattern
- SARCON (SAR Product Control Software), for the point target and distributed target analysis of different calibration targets.
Introduction of new SAR calibration technology: 116)
Due to the multitude of operational modes based on the active phased antenna array with hundreds of T/R modules, a large number of different antenna beams are obtained (some 10,000 for TSX-SAR). For this situation, a conventional calibration approach is not feasible. Hence, DLR/HR developed innovative and efficient calibration methods for the TSX-SAR instrument.
The two most important innovations are:
- PN-gating (Pseudo Noise-gating), a new method for internal calibration. PN-gating is a technique of monitoring the gain and phase variations in the transmit and receive paths of individual T/R modules while all 384 modules are operating - representing a characterization under the most realistic conditions with the advantage that all modules can be characterized simultaneously. For this purpose, the instrument is operated in a special module characterization mode. The simulated results of the PN-gating method are very promising (confirmed by ground tests).
- A precise antenna model, based on a new antenna pattern optimization. The modular nature of an active phased array antenna provides the capability of mathematical modelling. Such an antenna model is in fact a software tool that accurately determines the antenna beam patterns based on detailed characterization of the antenna hardware and the knowledge of the antenna control parameters. To achieve the required radiometric quality, highly accurate pre-launch characterization data was needed.
After pre-launch validation against the near-field pattern measurements and in-flight verification, the antenna model is used to generate the in-orbit calibrated beam patterns required for radiometric corrections in the SAR processing throughout the satellite lifetime. The antenna model is capable of accurately determining not only the individual beam patterns but also the relative gain variations from beam to beam. Determination of the absolute gain from measurements over external calibration targets can then be reduced to a few beams.
In order to maintain the SAR antenna performance, the following steps are implemented into the TSX-SAR calibration system:
• Compensation of radar instrument drift with the help of internal calibration
• Individual TRM (T/R Module) characterization by using the novel PN-gating method.
The internal calibration facility features a coupling into an additional port of each TRM. Calibration pulses are routed through the XFE to characterize critical elements of the transmit (Tx) and receive (Rx) path. The acquired signals can only be measured at the composite ports of the distribution networks. Three different types of calibration pulses are applied, whereby sets of these pulses are needed at the start and end of each data acquisition. All calibration pulses have the same length and bandwidth as the transmit pulse commanded for the mode.
In orbit, absolute power level degradation is calibrated via external targets like transponders or corner reflectors. Thus, only relative characterization results are of interest.
The calibration pulses are applied to the XFE to characterize the instrument's influence on the radar signal. The three types of calibration pulses account for the transmit path, the receive path, and for differences in the routing of the first two pulse types. The acquired signals can be measured at the receiving ports of the distribution networks. Evaluating the amplitude and phase of the calibration signals provides information on how to model the instrument drift during data acquisition. This drift is corrected during SAR image processing to obtain high-quality SAR products.
The spaceborne results from repeated measurements of the same instrument and antenna conditions prove the high measurement accuracy of the PN-gating technique. The repeated measurements were in a time frame of weeks. Still, the accordance of the estimation results to each other is almost perfect.

Calibration results:
During the commissioning phase, the baseline calibration procedure approach of TSX was subdivided into six major tasks. The successive baseline calibration procedures were: 117) 118) 119)
• Geometric calibration, to assign the SAR data to the geographic location on the Earth's surface.
• Antenna pointing determination, to obtain a correct beam pointing of the antenna.
• Antenna model verification, to ensure the provision of the antenna patterns of all operation modes and the gain offset between different beams.
• Relative radiometric calibration, for radiometric correction of SAR data within an illuminated scene.
• Absolute radiometric calibration, for measuring the SAR system against standard targets with well-known radar cross section (RCS).
The results of calibrating TerraSAR-X approve the accuracy calculated before launch and put the new strategy to calibrate efficiently a multiple mode SAR system like TerraSAR-X on a solid base. The key element of this strategy was an antenna model approach. TerraSAR-X is the first SAR satellite calibrated with this innovative method.
All requirements and/or goals have been achieved even better than predicted. Through this successful demonstration of an effective and exact calibration technique, a new benchmark has been reached not only for calibrating complex SAR systems but in principle for future highly accurate spaceborne SAR sensors like TanDEM-X or Sentinel-1 (Ref. 117).
SAR Interferometry
TerraSAR-X offers a number of new perspectives to SAR interferometry when compared to ERS and also to Envisat: 120)
• High resolution of 3 m or better in stripmap and spotlight mode
• The option for a burst synchronized scanSAR mode
• The high range bandwidth will allow large baselines and the option for highly precise DEM generation
• X-band will show new scattering properties
• High observation frequency due to the short repeat cycle and variable incidence
• An ATI (Along Track Interferometric) mode.
The rather short orbit repeat cycle of 11 days and the electronically steerable antenna allows fast and frequent imaging of a certain site. At average latitudes, an interferometric pair can be acquired within only 12 days. And within 22 days 12 interferometric pairs with different observation geometries can theoretically be acquired. Note that the nominal antenna look direction is to the right. The left-looking mode is possible but has some operational deficiencies so it will only be used in high-priority situations.
Secondary Payloads
TOR (Tracking, Occultation and Ranging)
The TOR experiment is furnished by GFZ Potsdam, Germany. and CSR (Center of Space Research) at UTA (the University of Texas at Austin), USA. The TOR payload consists of the dual-frequency GPS receiver IGOR (Integrated GPS Occultation Receiver), developed and built by Broad Reach Engineering Company of Tempe, AZ, and LRR (Laser Retro Reflector) for evaluation of GPS-based orbit data as an independent tracking technique. The IGOR design is of BlackJack heritage of NASA/JPL, flown on such missions as CHAMP, SAC-C, Jason-1, and GRACE.
- The overall objectives are 121) 122)
1) To collect atmospheric and ionospheric radio occultation (RO) data using IGOR and to augment the global RO data sets obtained from other LEO satellites (namely CHAMP and GRACE) to be used for improvements of numerical weather forecasts, climate change studies and space weather monitoring. Derived fundamental atmospheric and ionospheric quantities (e.g. temperature, water vapour) are being used as complementary information for SAR data error correction.
2) Use of the resulting high-quality orbit and atmospheric correction products in conjunction with DLR-provided TSX-SAR data for improved analysis.
Specific objectives for the TSX-SAR science studies are:
- Landslide, rock fall and urban land subsidence
- Ice sheet applications
- TSX-SAR digital terrain model for surface water availability modelling
- Seismic loading cycle studies
3) The IGOR GPS tracking capabilities are being used for ground-based POD (Precise Orbit Determination) algorithm processing with accuracies of < 5 cm in position (IGOR is a 48-channel space-qualified GPS receiver). The orbits can be made available within less than 3 hours after data reception and are being used for SAR science analysis and eventually for NWP analysis. 123)
The IGOR payload suite consists of a dual-redundant dual-frequency (L1/L2) GPS receiver unit, a built-in SSR(Solid-State Recorder), a built-in PC, and an antenna set comprised of:
• Two POD (Precision Orbit Determination) L1/L2 patch antennas
• Two RO (Radio Occultation) antennas 1x4 L1/L2 patch arrays
The IGOR instrument [GPS, SSR, antennas, PC] consists of a box of size 200 mm x 240 mm x 100 mm with a mass of about 4.2 kg.

The main components of the infrastructure for TerraSAR-X GPS occultations are the GPS receiver onboard the satellite and the ground segment. It consists of the Polar receiving station at Ny-Ålesund (Spitzbergen), the fiducial GPS ground network, the ultra-rapid precise orbit determination facility, the operational occultation processing system and, for archiving and distribution: the TerraSAR-X data Center.
The generation of the TerraSAR-X GPS occultation data products will be performed by an automatic data processing system, which will be based on the CHAMP/GRACE processing system. This system is designed to be extendable for the processing of additional GPS occultation missions, as of TerraSAR-X.
Support mode | Data product | Description |
TOR-SAR | PSO (Precise Science Orbit) | Highly precise orbit generated with a time delay of a few days after request by TOR-SAR for InSAR processing |
SAR | High-precision regional and local SAR products for the identified SAR analyses of the project team | |
TOR-RO | USO (Ultra-rapid Science Orbit) | Precise orbit delivered with a latency of fewer than 3 hours after data taken for occultation processing |
Atmospheric excess phases | Calibrated atmospheric excess phases of the occultation radio link including precise satellite orbit data (position and velocity) for each occultation | |
Occultation tables | Lists of daily occultation events including additional information as e.g. location and time, duration, satellite and ground station numbers | |
Atmospheric profiles | Vertical profiles of atmospheric parameters: bending angles, refractivity, pressure, density, temperature from Earth's surface to 40 km height, water vapour up to 20 km | |
Ionospheric profiles | Vertical profiles of electron density from Earth’s surface to the orbit height of TerraSAR-X | |
TOR-POD | PSO (Precise Science Orbit) | Highly precise orbit generated with a time delay of a few days after request by TOR-SAR for InSAR processing |
USO (Ultra-rapid Science Orbit) | Precise orbit delivered with a latency of fewer than 3 hours after data take for occultation processing |


Two independent GPS receiver units are used on TerraSAR-X for increased redundancy. For each receiver, an independent passive GPS antenna (Seavey SPA-16C/S) and low noise amplifier (Delta Microwave L5690) are employed, which enable representative signal-to-noise ratios (C/N0) of 45 dB-Hz near the boresight direction. To provide adequate sky coverage during right- and left-looking SAR operations, the MosaicGNSS GPS antennas are oriented opposite to the SAR antenna. In the nominal flight configuration (right-looking SAR), the boresight points to the left of the flight direction with a 33.8º offset from the vertical. The IGOR choke ring antennas, in contrast, are exactly zenith pointing (Ref. 17).
LRR (Laser Retro Reflector)
LRR is of CHAMP and GRACE heritage developed at GFZ. A passive optical device for accurate satellite tracking from ground laser ranging stations of the SLR network. SLR tracking to spaceborne laser retro-reflectors is performed by a global network of about 40 ground stations of the ILRS (International Laser Ranging Service). The SLR data provide an independent observation type at a comparable accuracy level to the onboard GPS tracking data for the quality assurance of the TerraSAR-X POD (Precise Orbit Determination).
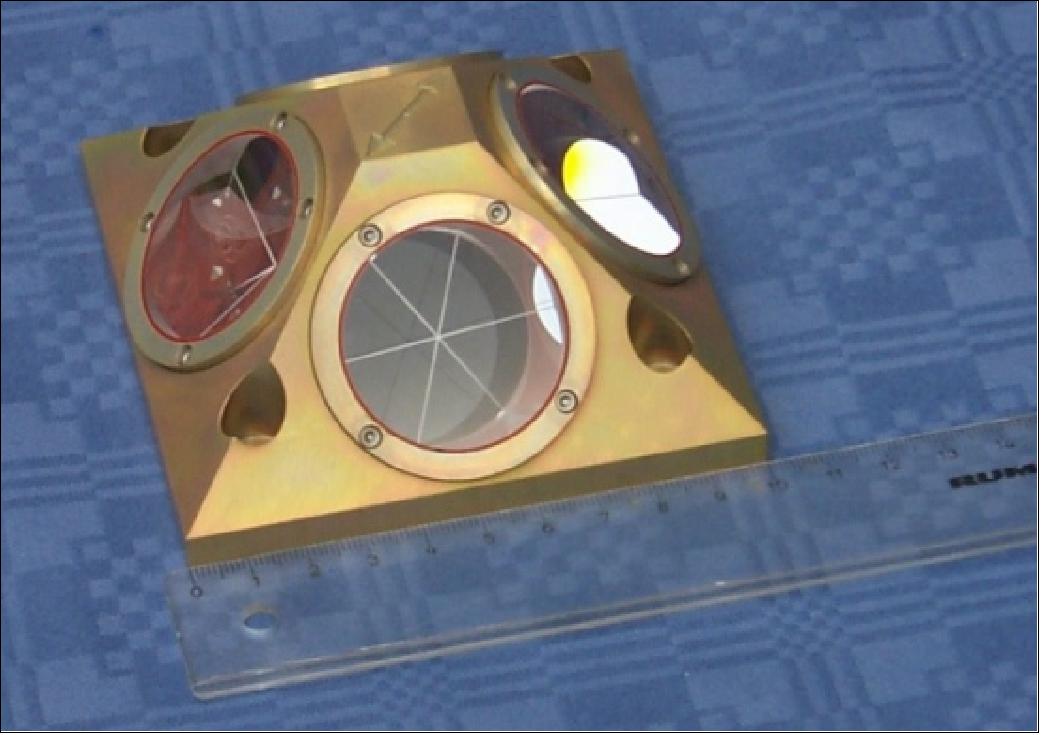
LCT (Laser Communication Terminal)
TSX-LCT is an experimental secondary payload, developed and built by Tesat-Spacecom GmbH of Backnang as the prime contractor (formerly Bosch SatCom GmbH; Tesat Spacecom is owned by EADS Astrium), Germany. LCT development contributions come also from EADS Astrium and Zeiss Optronik. LCT funding comes from DLR and from BMBF. The LCT (optical communication) technology and implementation were chosen due to its potential of providing higher data rates, lower mass and lower power than required by conventional RF communications. 124) 125) 126) 127) 128) 129)
The LCT objective is to provide either a bidirectional communications link for binary digital data transfer between two satellites, (like LEO-MEO) or between one satellite and an optical ground station.
LCT on TerraSAR-X, also referred to as LCTSX, is designed as a COTS (Commercial-Off-The-Shelf) product and is already being made available to other missions. Tesat-Spacecom provides the full ground test equipment especially the so-called STB (System Test Bed) to verify the complete sequence of LCT operational states from start-up over acquisition and tracking to communication under space environment, i.e. link distance, satellite's in-orbit vibrations, sun light.
- The intent is to use LCT on future high data rate missions, in Earth observation as well as in commercial communications. 130) 131) 132)

Background: The on-orbit verification of the first coherent optical communication system on a satellite builds on more than 15 years of European efforts under programs and studies like SOLACOS (Solid State Laser Communications in Space), DLR-LCT, MEDIS (Multimedia Experiment and Demonstration System), and SILEX (Semiconductor Intersatellite Link Experiment). SILEX, an ESA design, was the only project that was realized, with an LEO terminal on SPOT-4 (launch March 24, 1998) and a GEO terminal on ARTEMIS (launch July 12, 2001).
In 1998, Tesat-Spacecom was selected for the Celestri and Teledesic programs by Motorola to build all laser crosslinks for a LEO constellation (in the meantime Teledesic program was cancelled). The laser oscillators, qualified for Teledesic, are now foreseen for many scientific missions like DWL, GIFTS and ALADIN.
Note: LCT is considered the first laser communication system in space that operates on a coherent basis (homodyne digital receiver and BPSK modulation). In a coherent system, the receiver operates by optically adding a locally generated field to the receiver field prior to photodetection. The prime objective is to use the added local field to improve the detection of the weaker received field in the presence of the receiver thermal noise. - In contrast, the SILEX concept employs a direct-detection system in which the desired information is intensity modulated onto an optical source and transmitted to the receiver terminal. In this concept, the photodetector at the receiver side provides basically the function of a power detection device. - While coherent detection is the most advanced technology, which enables the maximum data rate at minimum power, it requires relatively complicated hardware and it cannot be used through atmospheric turbulence.
Homodyne BPSK optical modulation scheme (Ref. 72)
Homodyne BPSK (Binary Phase Shift Keying) is superior to all other optical modulation schemes since it is the most sensitive for both, tracking and communication. More important, however, is its immunity against sunlight. Homodyne BPSK allows to the maintenance of the communication link, and as a precondition also tracking, even if the sun is in the receiver's field of view.
Homodyne BPSK is based on phase modulation and coherent detection. The signal to be detected is superposed to the beam of a local oscillator laser running on the same frequency as the signal's carrier. With the optical phases of both, the signal carrier and local oscillator, being locked to each other one have a sensitive detection and demodulation scheme for the phase signal.
The same LCT system of Tesat-Spacecom is also flown on the NFIRE (Near Field Infrared Experiment) spacecraft of the US MDA (Missile Defense Agency) of DoD with a launch on April 24, 2007, from the Mid-Atlantic Regional Spaceport on Wallops Island, VA (Minotaur vehicle of OSC). The NFIRE spacecraft has been built by General Dynamics C4 Systems of Gilbert, Arizona (formerly SpectrumAstro). LCT is installed on NFIRE as a secondary payload to evaluate laser communication technology. The primary mission of the NFIRE satellite is to collect images of a boosting rocket to improve understanding of exhaust plume phenomenology and plume-to-rocket body discrimination. In addition, forest fires, volcanoes and ground-based rocket engine tests are on NFIRE's observation list for the two-year mission as well.
In a further verification step, an ISL (InterSatellite Link) between LCT on TerraSAR-X and LCT on the NFIRE satellite is planned to be established.
Parameter | Value | Parameter | Value |
User serial data rate | 24 x 225 Mbit/s | Sun passage capability | Communication links are maintained when satellites pass in front of the sun |
Optical serial data rate | 5.5 Gbit/s | ||
Operating wavelength | 1064 nm | Radiation tolerance | ≥ 80 krad |
Modulation scheme | BPSK | Detection scheme | Coherent, homodyne |
Pointing range | Complete hemisphere | Instrument mass | 25 kg including radiation shielding |
Optical output power | 0.5 W nominal, switchable to 1 W | Power consumption | 35 W (average), < 130 W (max) |
Optical interfaces | One common path for transmitting & receive beam | Doppler compensation range | ±7 km/s line-of-sight velocity |
Acquisition scheme | Beaconless, both terminals spatially scanning | Scalability | ≥ 86,000 km |
BER (Bit Error Rate) | < 10-8 for up to 8,000 km range inter-satellite link | BER | < 10-4 for link through the atmosphere |
Instrument: LCT is accommodated on the anti-sun side of TerraSAR-X to guarantee a free hemispherical FOV which includes the Earth's surface. The terminal consists of one physical unit with a mass of about 33 kg and an average power consumption of 136 W. For thermal control reasons, LCT is equipped with a heat pipe radiator having a radiator area of 0.57 m2 and a mass of about 10 kg. Due to the generous energy margin of the spacecraft, LCT can be operated in parallel with the TSX-SAR instrument for most of the year.
The instrument is functionally divided into Optics Unit (OU) which includes the coarse pointing mechanism, telescope, fine pointer and receiver and a Frame Unit. The OU consists of the beam-handling elements of the terminal. The CPU (Coarse Pointing Unit) is designed as a stiff lightweight construction to guide incoming and outgoing laser beams from/to its free aperture to the telescope (124 mm aperture diameter). The aperture of the CPU is steered by two independent motors with full pointing, tracking and communications performance over the entire hemisphere. Within the CPU, the beam is guided by two high-precision mirrors keeping track of the optical path onto the line-of-sight to the counter terminal. The leaving and received beams have a common path through the telescope (athermal, lightweight) for magnification/demagnification and through the Fine Pointing Unit (FPU).
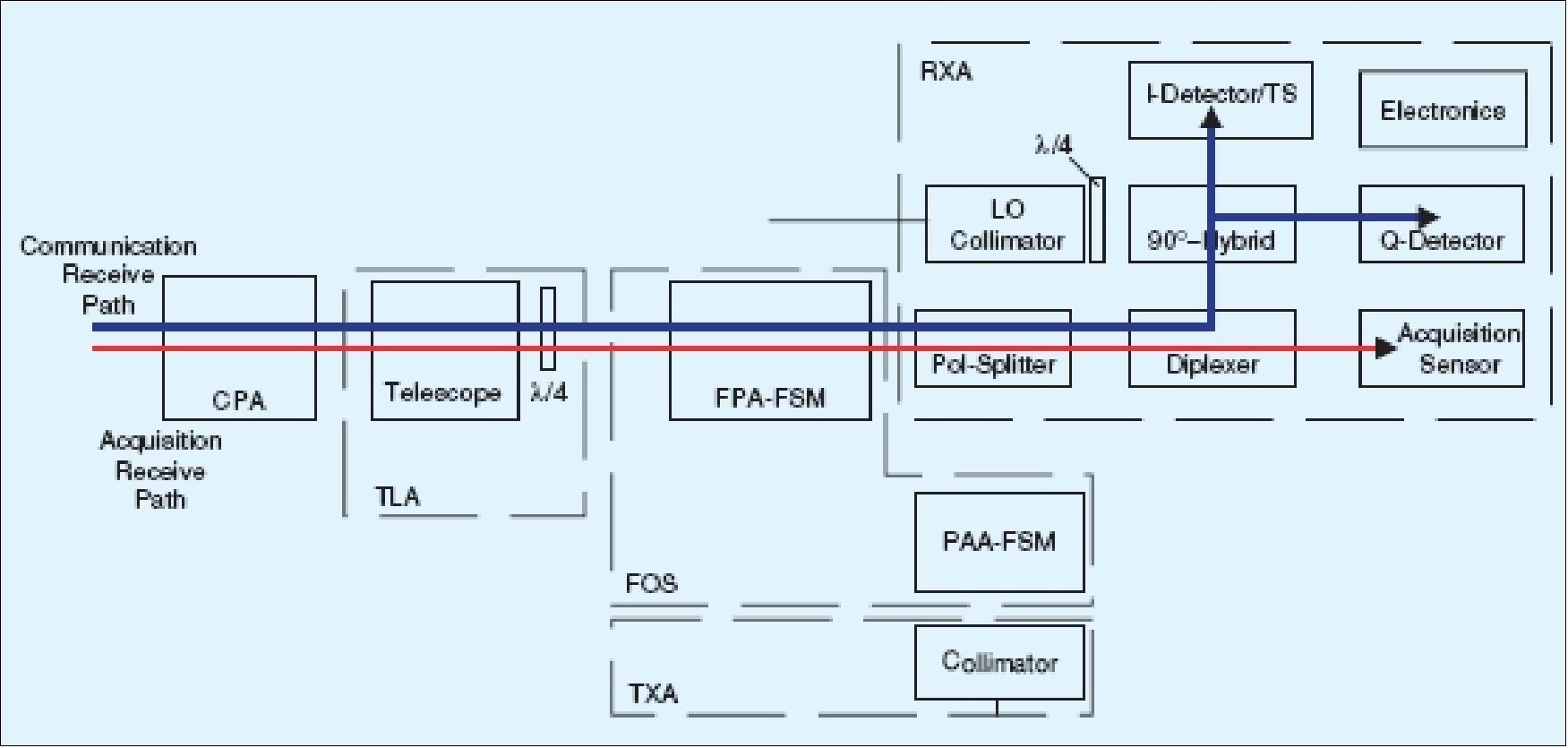
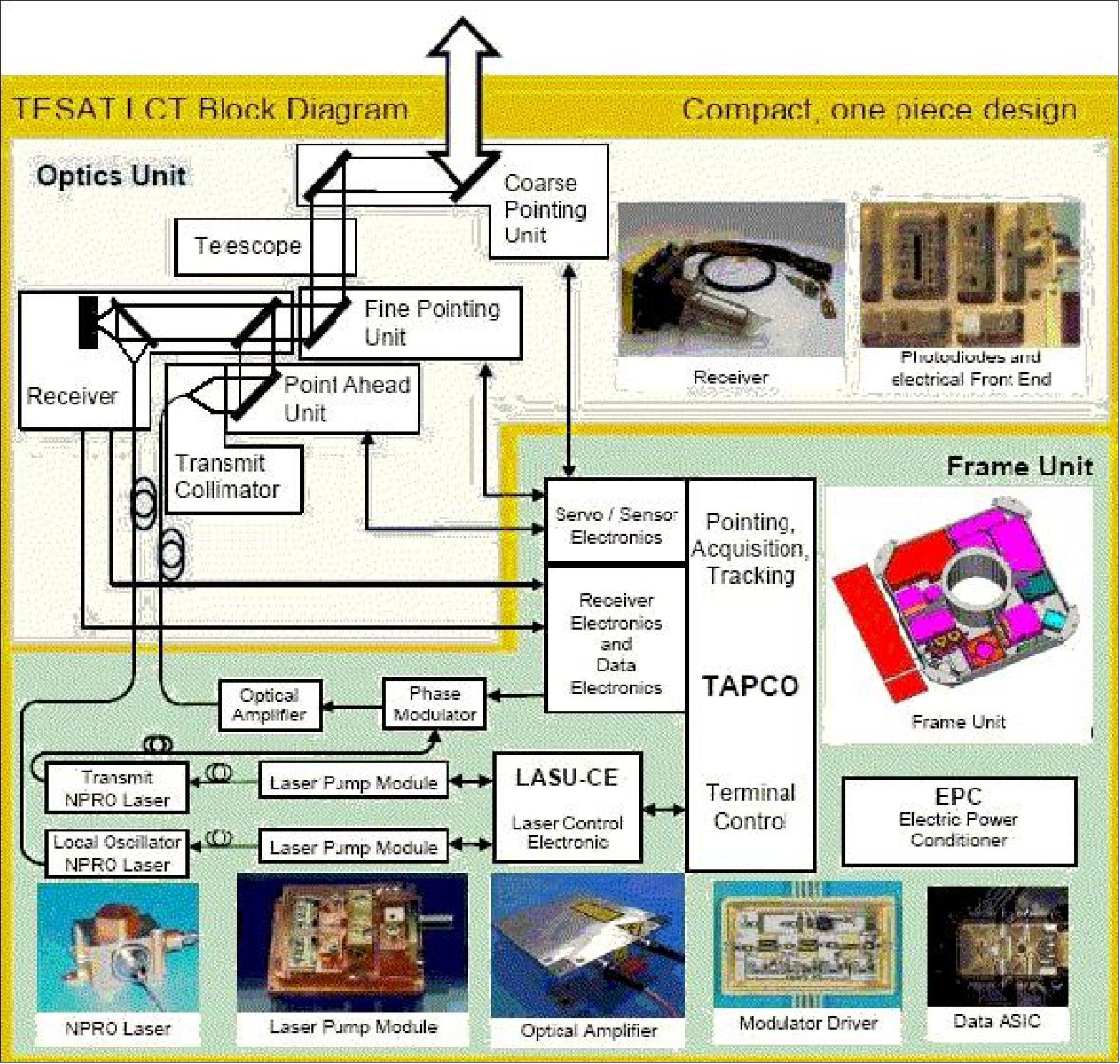
The FPU employs a high-bandwidth pointing mechanism which provides the necessary tracking gain at frequencies up to 1 kHz in order to cancel satellite vibrations. The receiving beam is then separated from the leaving beam path by a polarization beam splitter and sent to the receiver. The receiver contains the 90º hybrid replacing the optical bench. There the received beam is superposed with a local oscillator laser in order to extract maximum sensitivity for a given received power level. The 90º hybrid has the added functionality of generating four separate beams with 0º, 90º, 180º and 270º relative phase shifts. The acquisition, tracking and communications information contained within the four outputs of the 90º hybrid is detected in the photodiode arrays of the electrical front end, where they are immediately preprocessed. Further processing of this information (phase locking, data extraction, tracking servo) is performed by the electronics located in the Frame Unit.
The Frame Unit contains the EPC (Electrical Power Conditioner) for the terminal, the Terminal and PAT (Pointing, Acquisition and Tracking) Controller (TAPCO), data electronics and the Laser Subsystem. The Laser Subsystem contains two highly reliable diode pump modules for pumping two solid-state lasers in NPRO [(Non-Planar Ring Oscillator) after Kane, Byer] configuration. One laser serves as transmit laser. The second laser serves as a local oscillator and is fed directly to the receiver optical bench. The laser pump modules were developed to a large extent by researchers at the Fraunhofer Institute for Laser Technology (ILT) in Aachen, Germany, on behalf of Tesat.
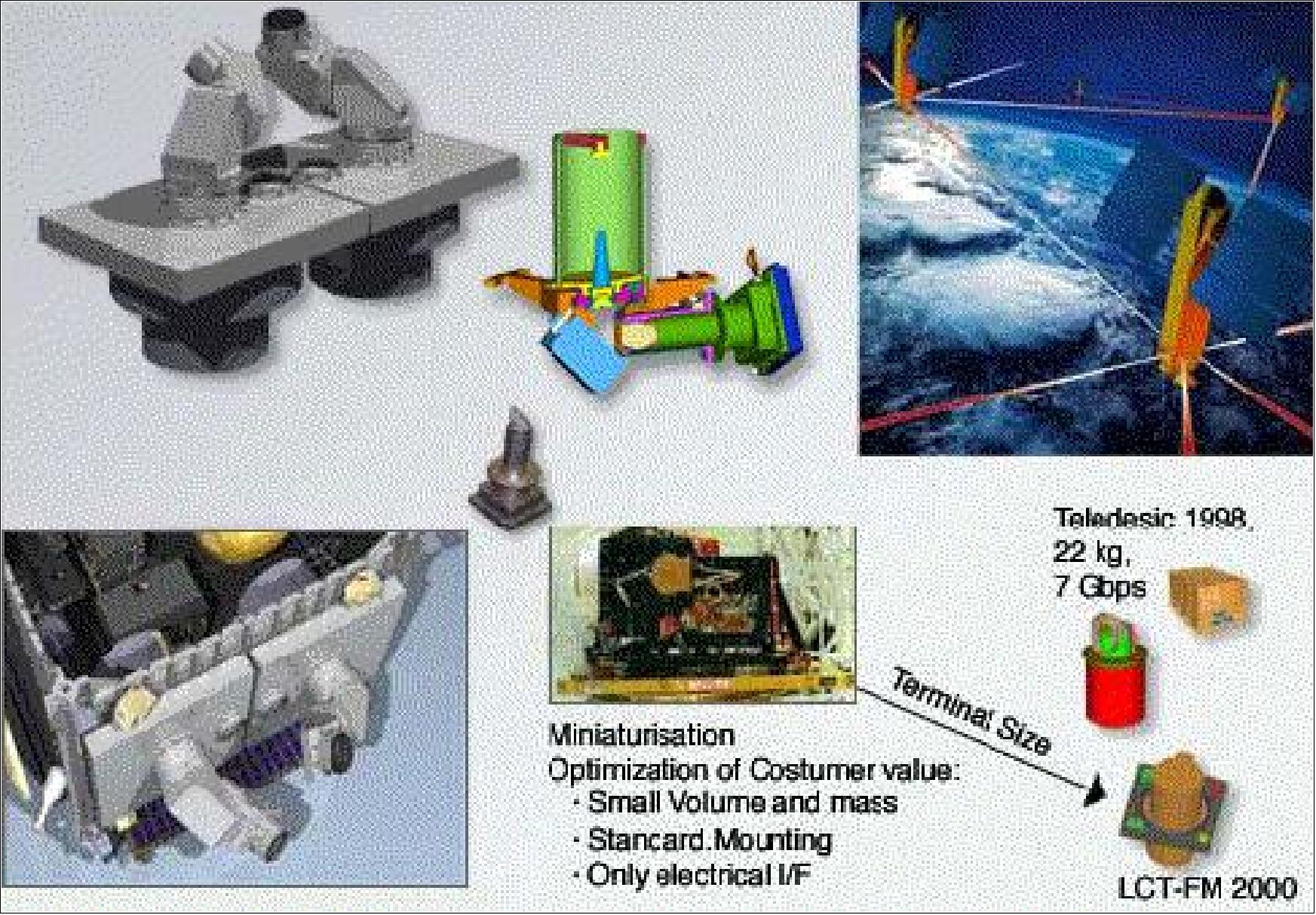
The LCT equipment employs a high-performance PowerPC processor (505 MIPS) which allows running both tasks, real-time control loops and TM/TC data processing, at the same time on a single computer. It exhibits small dimensions (180 mm x 160 mm x 60 mm) and low mass (1 kg). The power consumption is in the range of 15 W, depending on the processor load. The onboard computer provides a variety of hardware interfaces, especially high-resolution A/D and D/A interfaces as well as a MIL-STD-1553B interface. Due to its modular software architecture, the functionality can be easily adapted to application-specific requirements. 133)
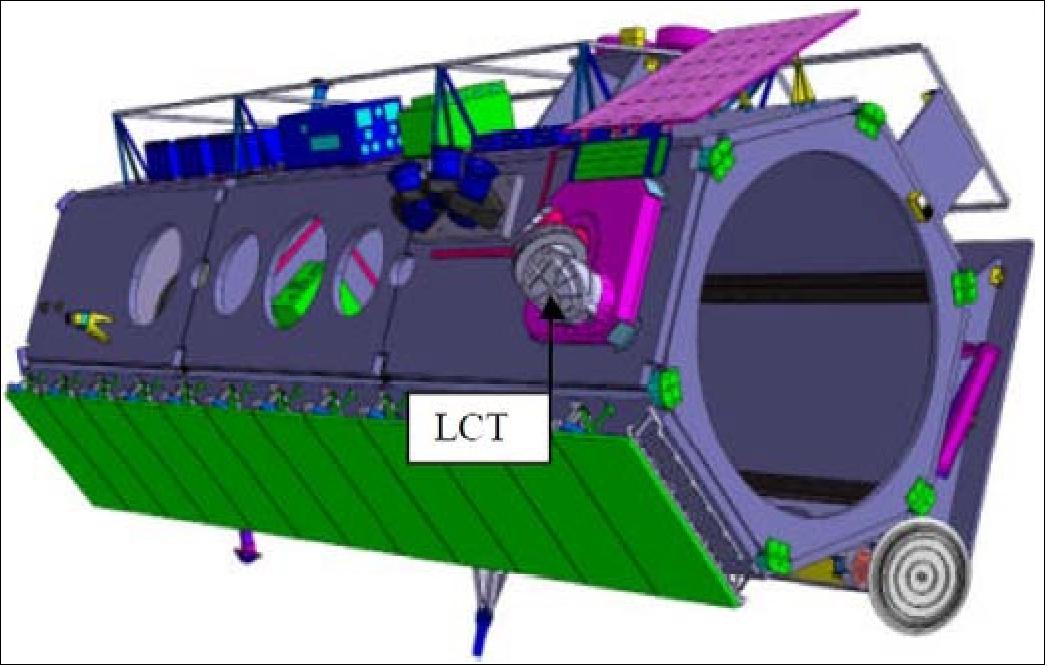

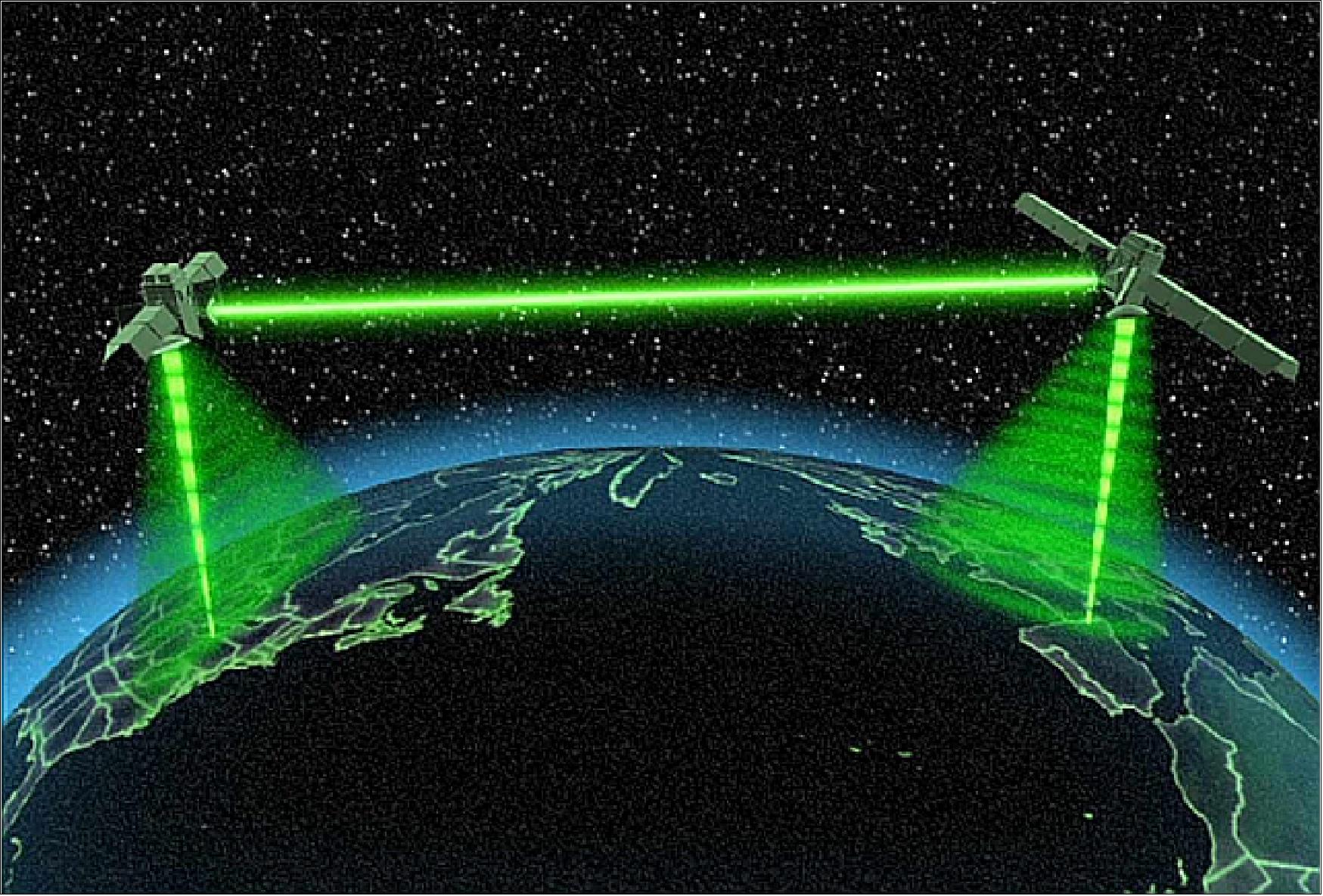
Ground Segment
The TerraSAR-X ground segment is composed of the following elements: 134) 135) 136)
• DLR/GSOC is providing the functions of spacecraft operations (commanding, monitoring, planning & scheduling, orbit and attitude determination, etc.) and data acquisition with ground stations in Weilheim and Neustrelitz. Weilheim is used as TT&C station, while Neustrelitz serves as the central receiving station of payload data at 300 Mbit/s.
• DLR/HR (DLR/Institut für Hochfrequenztechnik und Radar) is providing the IOCS (Instrument Operation and Calibration Segment) function
• PGS (Payload Ground Segment) function. The DLR research functions of data processing, analysis and simulation are provided by DFD (Deutsches Fernerkundungsdatenzentrum) and IMF (Institut für Methodik der Fernerkundung). The DLR Ground Segment provides the main functions Space and Ground Segment planning, orbit control and analysis, spacecraft telemetry reception and command, data reception and archiving, calibration and performance analysis, product generation, delivery and provision of user services.
• To comply with increased requirements on data latency, especially from the Maritime and Emergency Response Services, the TerraSAR’s ground station network access has constantly been upgraded especially through additional polar station access.
Airbus Defence and Space (formerly EADS Astrium/Infoterra) is providing the service infrastructure for commercial data exploitation and customer interfaces. Additional direct payload access stations - commercial partners of Infoterra - are foreseen to extend the baseline receiving station concept.
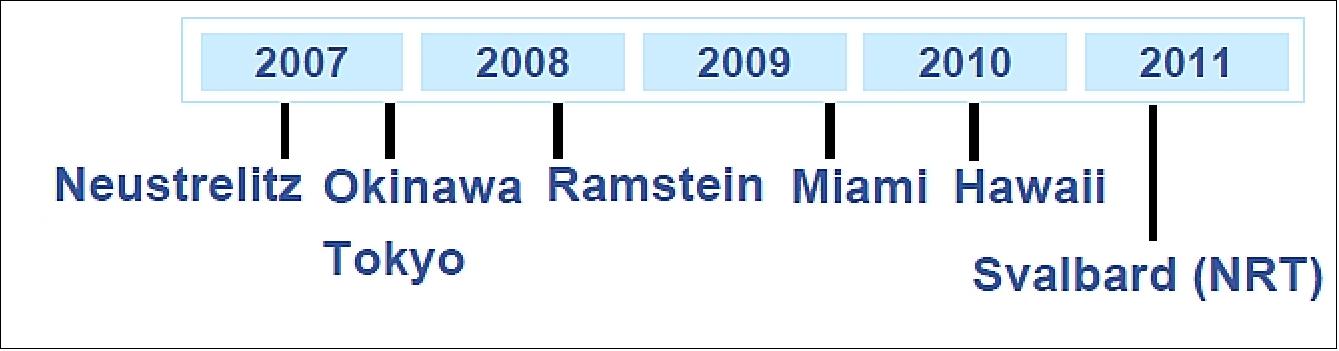
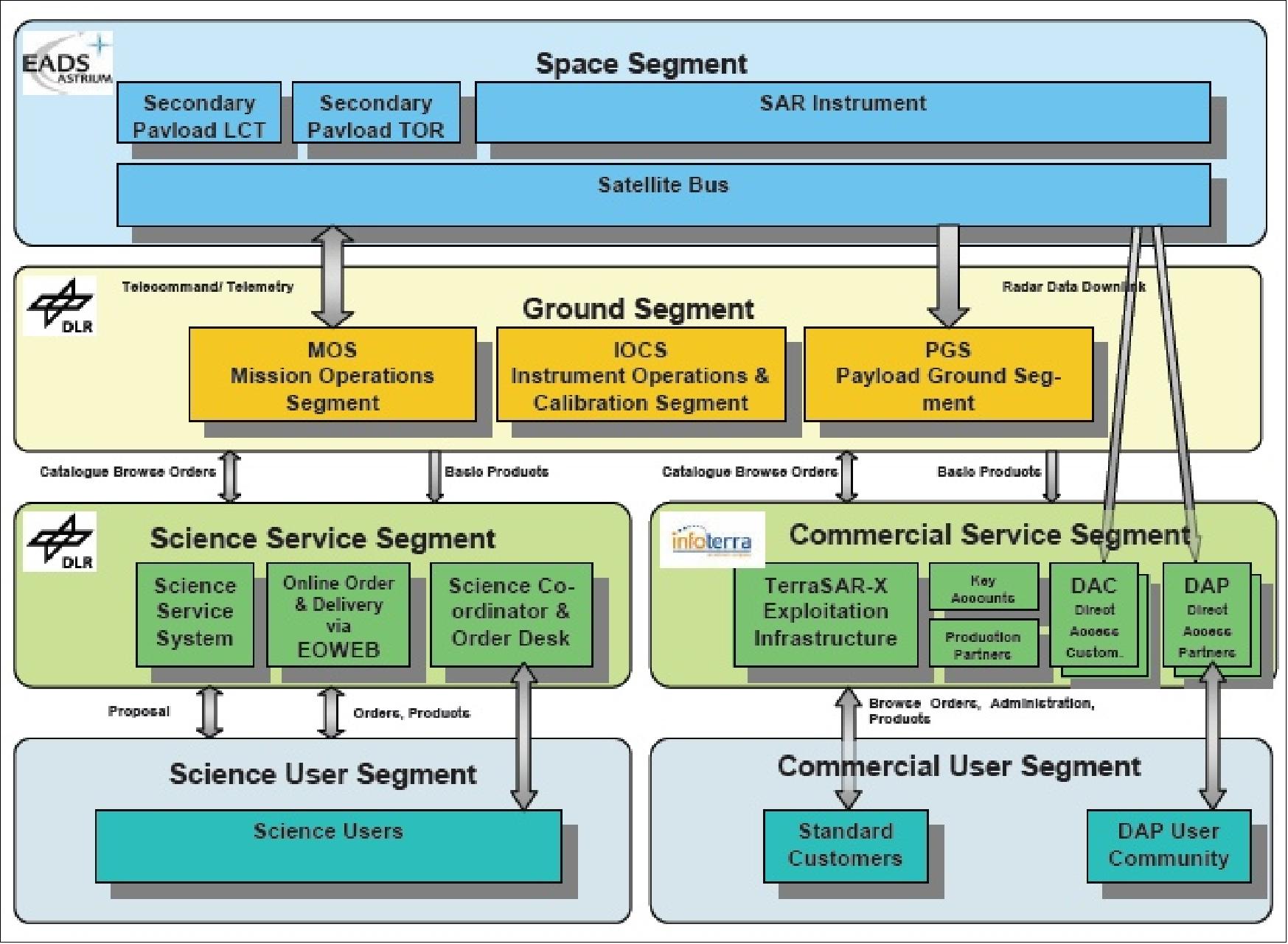


References
1) A. Roth, R. Werninghaus, “Status of the TerraSAR-X Mission,” Proceedings of IGARSS 2005, Seoul, Korea, July 25-29, 2005
2) R. Werninghaus, W. Balzer, S. Buckreuss, J. Mittermayer, P. Mühlbauer, W. Pitz, “The TerraSAR-X Mission,” Proceedings of EUSAR 2004, Ulm, Germany, May 25-27, 2004
3) S. Buckreuss, W. Balzer, P. Mühlbauer, R. Werninghaus, W. Pitz, “TerraSAR-X, A German Radar Satellite,” International Radar Symposium (IRS 2003), Dresden, Germany, Sept. 30 - Oct. 2, 2003
4) S. Buckreuss, W. Balzer, P. Mühlbauer, R. Werninghaus, W. Pitz, “The TerraSAR-X Satellite Project,” Proceedings of IEEE/IGARSS 2003, Toulouse, France, July 21-25, 2003
5) M. Suess, S. Riegger, W. Pitz, R. Werninghaus, “TerraSAR-X - Design and Performance,” Proceedings of EUSAR 2002, Cologne, Germany, June 4-6, 2002
6) A. Roth, M. Eineder, B. Schättler, “TerraSAR-X: A New Perspective for Applications Requiring High Resolution Spaceborne SAR Data,” Proceedings of ISPRS Workshop, Hannover, Germany, Oct. 6-8, 2003
7) R. Werninghaus, I. Zerfoswski, “The TerraSAR Mission,” Proceedings of Advanced SAR Workshop, Saint-Hubert, Quebec, Canada, June 25-27, 2003
8) M. Stangl, R. Werninghaus, R. Zahn, “The TerraSAR Active Phased Array Antenna,” 2003 IEEE International Symposium on Phased Array Systems and Technology, Boston, MA, Oct. 14-17, 2003
9) Stefan Ochs, Wolfgang Pitz, “The TerraSAR-X and TanDEM-X Satellites,” Proceedings of the 3rd International Conference on Recent Advances in Space Technologies (RAST 2007), Istanbul, Turkey, June 14-16, 2007
10) IEEE Geoscience and Remote Sensing, Feb. 2010, Vol. 48, No 2, Special Issue on TerraSAER-X: Mission, Calibration, and First Results
11) Astrium, “Radarservices with TerraSAR-X,” Briefing at the German Embassy, Ottawa, Canada, June 10, 2011
12) Gunter Schreier, “TerraSAR and German Contributionsto theGMES Programme,” Nov. 2008
13) “TerraSAR-X mission brochure: The German Radar Eye in Space,” July 2009, URL: http://www.dlr.de/Portaldata/28/Resources/dokumente/RE/Broschuere_TSX_lowres.pdf
14) Burkhard Fladt, “TerraSAR System Evolution - Toward New Applications,” EADS Astrium, URL: http://www.dlr.de/Portaldata/1/Resources/veranstaltungen/eo_konferenz/01_eads_astrium_2.pdf
15) Gunter Schreier, Marek Tinz, “German EO missions: TerraSAR-X, TanDEM-X, EnMAP, Firebird,” 3rd Ground Segment Coordination Body Workshop, Frascati, Italy, June 6-7, 2012
16) Michael Eineder,Irena Hajnsek, Gerhard Krieger, Alberto Moreira, Kostas Papathanassiou, “Tandem-L: Satellite Mission Proposal for Monitoring Dynamic Processes on the Earth’s Surface,” DLR Brochure, April 2014, URL: http://www.dlr.de/hr/en/Portaldata/32/Resources/dokumente/broschueren/Tandem-L_web_Broschuere2014_en.pdf
17) O. Montenbruck, Y. Yoon, J. S. Ardaens, D. Ulrich, “In-flight Performance Assessment of the Single Frequency MosaicGNSS Receiver for Satellite Navigation,” Proceedings of the 7th International ESA Conference on Guidance, Navigation & Control Systems (GNC 2008), June 2-5, 2008, Tralee, County Kerry, Ireland
18) ”German radar satellite TerraSAR-X – 15 years in space and still in perfect shape,” DLR News, 14 June 2022, URL: https://www.dlr.de/content/en/articles/news/2022/02/20220614_15-years-terrasar-x.html
19) Information provided by Stefan Buckreuss of DLR, Oberpfaffenhofen.
20) ”TerraSAR-X ESA archive,” ESA, 22 April 2021, URL: https://earth.esa.int/eogateway/catalog/terrasar-x-esa-archive
21) ”Glacier retreat in Antarctica – innovative radar technologies enable improved predictions,” DLR, 1 February 2019, URL: https://www.dlr.de/dlr/en/desktopdefault.aspx/tabid-10081/151_read-31986/year-all/#/gallery/33389
22) P. Milillo, E. Rignot, P. Rizzoli, B. Scheuchl, J. Mouginot, J. Bueso-Bello, P. Prats-Iraola, ”Heterogeneous retreat and ice melt of Thwaites Glacier, West Antarctica,” Science Advances, Volume 5, No 1, 30 January 2019, eaau3433, https://doi.org/10.1126/sciadv.aau3433, URL: http://advances.sciencemag.org/content/advances/5/1/eaau3433.full.pdf
23) Stefan Buckreuss, Thomas Fritz, Markus Bachmann, Manfred Zink, ”TerraSAR-X and TanDEM-X Mission Status,” EUSAR 2018 (12th European Conference on Synthetic Aperture Radar), Aachen, Germany, June 4-7, 2018
24) Information provided by Stefan Buckreuss of DLR/HR (Microwave and Radar Technology Institute).
25) Michele Martone, Paola Rizzoli, Christopher Wecklich, Carolina González, José-Luis Bueso-Bello, Paolo Valdo, Daniel Schulze, Manfred Zink, Gerhard Krieger, Alberto Moreira, ”The global forest/non-forest map from TanDEM-X interferometric SAR data,” Remote Sensing of Environment, Vol. 205, pp: 352–373, Feb. 2018, URL of abstract: https://www.sciencedirect.com/science/article/pii/S0034425717305795
26) ”Project Forest/Non-Forest Map,” DLR/MRI, URL: http://www.dlr.de/hr/en/desktopdefault.aspx/tabid-12538/21873_read-50027
27) ”Excellence in space – 10 years of TerraSAR-X,” DLR, June 14, 2017, URL: http://www.dlr.de/dlr/en/desktopdefault.aspx/tabid-10081/151_read-22816/year-all/#/gallery/27214
28) ”Airbus celebrates 10 years of precision and reliability of TerraSAR-X satellite,” Airbus DS, June 2017, URL: http://www.intelligence-airbusds.com/en/7853-airbus-celebrates-10-years-of-precision-and-reliability-of-terrasar-x-satellite
29) ”Landmarks Calendar 2017 Celebrating 10 Years TerraSAR-X, Airbus DS; URL: http://www.intelligence-airbusds.com/en/5751-image-detail?img=42196#.WUqCF25Lc_c
30) Information provided by Stefan Buckreuss of DLR/HR (Microwave and Radar Technology Institute).
31) ”The future of radar – scientific benefits and potential of TerraSAR-X and TanDEM-X,” DLR, Oct. 17, 2016, URL: http://www.dlr.de/dlr/en/desktopdefault.aspx/tabid-10081/151_read-19779/year-all/151_page-3/#/gallery/24722
32) Birgit Schättler, Falk Mrowka, Egbert Schwarz, Marie Lachaise, ”The TerraSAR-X ground segment in service for nine years: current status and recent extensions,” Proceedings of the IEEE IGARSS (International Geoscience and Remote Sensing Symposium) Conference, Beijing, China, July 10-15, 2016
33) Stefan Buckreuss, Manfred Zink, ”TerraSAR-X and TanDEM-X Mission Status,” Proceedings of EUSAR 2016, 11th European Conference on Synthetic Aperture Radar, Hamburg, Germany, June 6-9, 2016
34) Christopher Wecklich, Carolina González, Benjamin Bräutigam, Paola Rizzoli, ”Height Accuracy and Data Coverage Status of the Global TanDEM-X DEM,” Proceedings of EUSAR 2016, 11th European Conference on Synthetic Aperture Radar, Hamburg, Germany, June 6-9, 2016
35) ”TerraSAR-X and RADARSAT-2 to Improve Monitoring Over North Canadian Region,” Airbus DS, Press Release, Nov. 30, 2015, URL: http://www.space-airbusds.com/en/press_centre/terrasar-x-and-radarsat-2-to-improve-monitoring-over-north-canadian-region.html
36) Information provided by Stefan Buckreuss of DLR/MRI (Microwave Radar Institute), Oberpfaffenhofen.
37) “TanDEM-X – Start of the Science Phase of the mission,” DLR Press Release, Oct. 10, 2014, URL: http://www.dlr.de/dlr/presse/en/desktopdefault.aspx/tabid-10312/475_read-11787/#/gallery/16691
38) I. Hajnsek, T. E. Busche, G. Krieger, M. Zink, A. Moreira, “TanDEM-X Ground Segment — Announcement of Opportunity: TanDEM-X Science Phase,” DLR/MRI, May 19, 2014, URL: https://tandemx-science.dlr.de/pdfs/TD-PD-PL_0032TanDEM-X_Science_Phase.pdf
39) Elke Heinemann, Christian Minet, “TerraSAR-X image shows spread of lava at Bardarbunga,” DLR, Sept. 16, 2014, URL: http://www.dlr.de/dlr/en/desktopdefault.aspx/tabid-10081/151_read-11583/year-all/#/gallery/16454
40) “A volcano comes to life – satellite picture of Bardarbunga on Iceland,” DLR, Sept. 10, 2014, URL: http://www.dlr.de/dlr/en/desktopdefault.aspx/tabid-10081/151_read-11494/year-all/#/gallery/16367
41) Stefan Buckreuss, “TerraSAR-X Mission Status,” Proceedings of EUSAR 2014 (10th European Conference on Synthetic Aperture Radar), Berlin, Germany, June 3-5, 2014
42) Ulrich Steinbrecher, Thomas Kraus, Gabriel Castellanos Alfonzo, Christo Grigorov, Daniel Schulze, Benjamin Bräutigam, “TerraSAR-X: Design of the new operational Wide ScanSAR mode,” Proceedings of EUSAR 2014 (10th European Conference on Synthetic Aperture Radar), Berlin, Germany, June 3-5, 2014
43) Corinna Prietzsch, Lars Petersen, Oliver Lang, Jan Anderssohn, Diana Weiling, “Site Monitoring applications with high-resolution TerraSAR-X data,” Proceedings of EUSAR 2014 (10th European Conference on Synthetic Aperture Radar), Berlin, Germany, June 3-5, 2014
44) Johannes Böer, Ulrich Steinbrecher, Markus Bachmann, Daniel Schulze, Benjamin Bräutigam, “Overview and Status of TerraSAR-X / TanDEM-X Long Term System Monitoring,” Proceedings of EUSAR 2014 (10th European Conference on Synthetic Aperture Radar), Berlin, Germany, June 3-5, 2014
45) Miriam Kamin, Susanne Lehner, “DLR satellite data helps in the rescue of the Akademik Shokalskiy,” DLR, January 09, 2014, URL: http://www.dlr.de/dlr/en/desktopdefault.aspx/tabid-10081/151_read-9173/year-all/#gallery/13383
46) Thomas Schrage, Juergen Janoth, Alexander Kaptein, Noemie Bernede, Steffen Gantert, Ralf Duering, “TerraSAR-X Next Generation – Mission Overview,” Proceedings of the 64th International Astronautical Congress (IAC 2013), Beijing, China, Sept. 23-27, 2013, paper: IAC-13-B1.2.8
47) Josef Mittermayer, Steffen Wollstadt, Pau Prats, Rolf Scheiber, Wolfgang Koppe, “Staring Spotlight Imaging with TerraSAR-X,” Proceedings of IGARSS (International Geoscience and Remote Sensing Symposium), Munich, Germany, July 22-27, 2012
48) “Discover TerraSAR-X New Imaging Modes,” Astrium, Oct. 2013, URL: http://www.astrium-geo.com/en/5054-discover-terrasar-x-new-imaging-modes
49) “Astrium Enhances TerraSAR-X Resolution and Coverage Capabilities,” Space Daily, Oct. 16, 2013, URL: http://www.spacedaily.com/reports/Astrium_Enhances_TerraSAR_X_Resolution_and_Coverage_Capabilities_999.html
50) Adrien Muller, Katja Bach, Noémie Bernede, Ralf Düring, Berthold Jäkle, Wolfgang Koppe, Oliver Lang, Sahil Suri, Thomas Schrage, Fernando Cerezo, Juan Ignacio Cicuendez Perez, Miguel Angel Garcia Primo, Basilio Garrido, Miguel Angel Serrano, “PAZ and TerraSAR-X Constellation, Innovation through International Cooperation,” Proceedings of the 64th International Astronautical Congress (IAC 2013), Beijing, China, Sept. 23-27, 2013, paper: IAC-13-B1.1.4
51) Stefan Buckreuss, Manuela, Braun,“Wide-angle view from space,” DLR News, Aug. 30, 2013, URL: http://www.dlr.de/dlr/en/desktopdefault.aspx/tabid-10081/151_read-7994/year-2013/%23gallery/12103#gallery/12103
52) “DLR delivers satellite images for Indian flood assistance,” DLR, June 24, 2013, URL: http://www.dlr.de/dlr/en/desktopdefault.aspx/tabid-10081/151_read-7429/year-all/#gallery/11369
53) “TerraSAR-X image of the month – the Santorini volcano expands,” DLR, Nov. 23, 2012, URL: http://www.dlr.de/dlr/en/desktopdefault.aspx/tabid-10081/151_read-5675/year-all/
54) “TerraSAR-X image of the month – stay clear of the salt flats,” DLR, Oct. 19, 2012, URL: http://www.dlr.de/dlr/en/desktopdefault.aspx/tabid-10081/151_read-5496/year-all/
55) “Anniversary in space – five years of TerraSAR-X,” DLR, June 15, 2012, URL: http://www.dlr.de/dlr/en/desktopdefault.aspx/tabid-10081/151_read-3950/year-all/
56) Elisabeth Mittelbach, Manfred Zink, “A step closer to mapping the Earth in 3D,” DLR, Jan. 13, 2012, URL: http://www.dlr.de/dlr/en/desktopdefault.aspx/tabid-10081/151_read-2451/year-2012/
57) “TerraSAR-X image of the month – Lively winter view,” DLR, March 2, 2012, URL: http://www.dlr.de/dlr/en/desktopdefault.aspx/tabid-10081/151_read-2809/year-all/
58) Luca Marotti, Pau Prats, Rolf Scheiber, Steffen Wollstadt, Andreas Reigber, “TOPS Differential SAR Interferometry with TerraSAR-X,” Proceedings of Fringe 2011, 8th International Workshop on 'Advances in the Science and Applications of SAR Interferometry,' Frascati, Italy, Sept. 19-23, 2011, (ESA SP-697 January 2012), URL of paper: http://elib.dlr.de/73447/1/marotti.pdf
59) “TerraSAR-X image of the month - Volcanic eruption in Chile,” DLR, July 22, 2011, URL: http://www.dlr.de/dlr/en/desktopdefault.aspx/tabid-10081/151_read-957/year-2011
60) “TerraSAR-X image of the month: Urban sprawl around Istanbul,” DLR, May 17, 2011, URL: http://www.dlr.de/dlr/en/desktopdefault.aspx/tabid-10212/332_read-784/
61) “DLR scientists support relief workers in Haiti earthquake disaster,” ESA, Jan. 16, 2010, URL: http://www.dlr.de/en/desktopdefault.aspx/tabid-6215/10210_read-22076/
62) Dana Floricioiu, Kenneth Jezek,Michael Eineder, Katy Farness, Wael Abdel Jaber, Nestor Yague-Martinez, “TerraSAR-X Observations over the Antarctic Ice Sheet,” Proceedings of IGARSS (IEEE International Geoscience and Remote Sensing Symposium) 2010, Honolulu, HI, USA, July 25-30, 2010
63) Marco Schwerdt, Dirk Schrank, Markus Bachmann, Clemens Schulz, Björn Döring, Jaime Hueso Gonzales, “TerraSAR-X Re-Calibration and Dual Receive Antenna Campaigns performed in 2009,” Proceedings of EUSAR 2010, 8th European Conference on Synthetic Aperture Radar, June 7-10, 2010, Aachen, Germany
64) Steffen Suchandt, Hartmut Runge, Alexander Kotenkov, Helko Breit, Ulrich Steinbrecher, “Extraction of traffic flows and surface current information using TerraSAR-X along-track interferometry data,” Proceedings of IGARSS (IEEE International Geoscience and Remote Sensing Symposium) 2009, Cape Town, South Africa, July 12-17, 2009
65) Roland Romeiser, Steffen Suchandt, Hartmut Runge, Ulrich Steinbrecher, “Analysis of first TerraSAR-X along-track InSAR derived surface current fields,” Proceedings of IGARSS (IEEE International Geoscience and Remote Sensing Symposium) 2009, Cape Town, South Africa, July 12-17, 2009
66) “TerraSAR-X Passes Two Year Mark,” Space Mart, June 19, 2009, URL: http://www.spacemart.com/reports/TerraSAR_X_Passes_Two_Year_Mark_999.html
67) Irena Hajnsek, Josef Mittermayer, Stefan Buckreuss, Kostas Papathanassiou, “Polarization Capabilities and Status of TerraSAR-X,” Proceedings of the 4th International POLinSAR 2009 Workshop, Jan. 26-30, 2009, ESA/ESRIN, Frascati, Italy, URL: http://earth.esa.int/workshops/polinsar2009/participants/441/pres_9_hajnsek_441.pdf
68) Stefan Buckreuss, Achim Roth, “The TerraSAR-X Mission,” 2nd STG/IPY SAR Coordination meeting, Oberpfaffenhofen, Sept 30, 2008, URL: http://bprc.osu.edu/rsl/GIIPSY/documents/1_TerraSAR-X-IPY-2008-09-30_Buckreuss%20%5BCompatibility%20Mode%5D.pdf
69) S. Buckreuss, A. Roth, “Status Report on the TerraSAR-X Mission,” Proceedings of IGARSS 2008 (IEEE International Geoscience & Remote Sensing Symposium), Boston, MA, USA, July 6-11, 2008
70) P. B. de Selding, “US-German Laser Intersatellite Link Performs Well on 2 Spacecraft,” Space News, March 17, 2008, pp. 1+4
71) “TerraSAR-X And NFIRE Fire Up The Pipe With Laser Data Transfer,” May 14, 2008, Space Daily, URL: http://www.spacemart.com/reports/TerraSAR_X_And_NFIRE_Fire_Up_The_Pipe_With_Laser_Data_Transfer_999.html
72) Robert Lange, Frank Heine, Hartmut Kämpfer, Rolf Meyer, "High Data Rate Optical Inter-Satellite Links," 35th ECOC (European Conference on Optical Communication) Sept. 20-24, 2009, Vienna, Austria
73) Carl Lunde, Renny Fields, Robert Wong, Josef Wicker, David Kozlowski, Jonah Skoog, Gerd Muehlnikel, John Hartmann, Uwe Sterr, Michael Lutzer, “Joint United States-Germany Satellite Laser Communications Project: NFIRE-to-TerraSAR-X ISLs and NFIRE-to-Ground SGLs,” International Workshop on Ground-to-OICETS Laser Communications Experiments 2010, (GOLCE 2010), May 13-15, 2010, Tenerife, Spain, paper: GOLCE2010-08
74) M. Gregory, F. Heine, H. Kämpfner , R. Meyer , R. Fields , C. Lunde, “Tesat Laser Communication Terminal Performance results on 5.6 Gbit Coherent Inter Satellite and Satellite to Ground Links,” ICSO 2010 (International Conference on Space Optics), Rhodes Island, Greece, Oct. 4-8, 2010
75) Ludwig Grunwaldt, Rolf König, “ILRS Support for TerraSAR-X and TanDEM-X - Status and Future Prospects,” 16th International Workshop on Laser Ranging, Poznan, Poland, October 13-17, 2008, URL: http://ilrs.gsfc.nasa.gov/docs/ilr_7_TXR.pdf
76) L. Grunwaldt, “The TOR Payload on Tandem-X,” Proceedings of EUSAR 2008, 7th European Conference on Synthetic Aperture Radar, June 2-5, 2008, Friedrichshafen, Germany
77) S. V. Baumgartner, M. Rodriguez-Cassola, A. Nottensteiner, R. Horn, R. Scheiber, M. Schwerdt, U. Steinbrecher, R. Metzig, M. Limbach, J. Mittermayer, G. Krieger, A. Moreira. “Bistatic Experiment Using TerraSAR-X and DLR's new F-SAR System,” Proceedings of EUSAR 2008, 7th European Conference on Synthetic Aperture Radar, June 2-5, 2008, Friedrichshafen, Germany
78) Marc Rodriguez-Cassola, Stefan V. Baumgartner, Gerhard Krieger, Anton Nottensteiner, Ralf Horn, Ulrich Steinbrecher, Robert Metzig, Markus Limbach, Pau Prats, Jens Fischer, Marco Schwerdt, Alberto Moreira, “Bistatic spaceborne-airborne experiment TerraSAR-X/F-SAR: data processing and results,” Proceedings of IGARSS 2008 (IEEE International Geoscience & Remote Sensing Symposium), Boston, MA, USA, July 6-11, 2008
79) “TerraSAR-X goes into operation,” January 9, 2008, DLR, URL: http://www.dlr.de/en/desktopdefault.aspx/tabid-4237/6774_read-11191/
80) J. Mittermayer, B. Schättler , M. Younis, “TerraSAR-X Commissioning Phase Execution and Results,” Proceedings of IGARSS 2008 (IEEE International Geoscience & Remote Sensing Symposium), Boston, MA, USA, July 6-11, 2008
81) J. Mittermayer, R. Metzig, U. Steinbrecher, C. Gonzalez, D. Polimeni, J. Böer, M. Younis, J. Marquez, S. Wollstadt, D. Schulze, A. Meta, N. Tous-Ramon, C. Ortega-Miguez, “TerraSAR-X Instrument, SAR System Performance & Command Generation,” Proceedings of IGARSS 2008 (IEEE International Geoscience & Remote Sensing Symposium), Boston, MA, USA, July 6-11, 2008
82) M. Schwerdt, B. Bräutigam, M. Bachmann, B. Döring, D. Schrank, J. Hueso Gonzalez, “TerraSAR-X Calibration Results,” Proceedings of IGARSS 2008 (IEEE International Geoscience & Remote Sensing Symposium), Boston, MA, USA, July 6-11, 2008
83) H. Breit, B. Schättler, T. Fritz, U. Balss, H. Damerow, E. Schwarz, “TerraSAR-X Payload Data Processing : Results from Commissioning and early Operational Phase,” Proceedings of IGARSS 2008 (IEEE International Geoscience & Remote Sensing Symposium), Boston, MA, USA, July 6-11, 2008
84) Adriano Meta, Pau Prats, Ulrich Steinbrecher, Rolf Scheiber, Josef Mittermayer, “First TOPSAR image and interferometry results with TerraSAR-X,” Fringe 2007 Workshop, ESA/ESRIN. Frascati, Italy, Nov. 26-30, 2007, URL: http://earth.esa.int/workshops/fringe07/participants/650/pres_650_meta.pdf
85) A. Meta, P. Prats, U. Steinbrecher, J. Mittermayer, R. Scheiber, “TerraSAR-X TOPSAR and ScanSAR comparison,” Proceedings of EUSAR 2008, 7th European Conference on Synthetic Aperture Radar, June 2-5, 2008, Friedrichshafen, Germany
86) F. De Zan, A. M. Monti Guarnieri, “TOPSAR: Terrain Observation by Progressive Scans,” IEEE Transactions on Geoscience and Remote Sensing, Vol. 44, Issue 9, Sept. 2006, pp. 2352-2360
87) D. D'Aria, F. De Zan, D. Giudici, A. Monti Guarnieri, F. Rocca, “Burst-mode SARs for wide-swath surveys,” Canadian Journal of Remote Sensing, Vol. 33, No 1, 2007, pp. 27-38
88) Pau Prats, Luca Marotti, SteffenWollstadt, Rolf Scheiber, “TOPS Interferometry with TerraSAR-X,” Proceedings of EUSAR 2010, 8th European Conference on Synthetic Aperture Radar, June 7-10, 2010, Aachen, Germany
89) S. Buckreuss, R. Werninghaus, W. Pitz, “TerraSAR-X Mission Status,” Proceedings of the International Radar Symposium 2007 (IRS 2007), Cologne, Germany, Sept. 5-7, 2007
90) M. A. K. Biller, U. Hackenberg, R. Rieger, B. Schweizer, M. Wahl, B. Adelseck, H. Brugger, M. Lörcher, “Advanced RF Sensors for SAR Earth Observation using High Precision T/R-Modules,” Proceedings of the Advanced RF Sensors for Earth Observation 2006 (ASRI), Workshop on RF and Microwave Systems, Instruments & Sub-Systems, ESA7ESTEC, Noordwijk, The Netherlands, Dec. 5-6, 2006
91) A. Herschlein, C. Fischer, H. Braumann, M. Stangl, W. Pitz, R. Werninghaus, “Development and Measurement Results for TerraSAR-X Phased Array,” Proceedings of EUSAR 2004, Ulm, Germany, May 25-27, 2004
92) U. Hackenberg, M. Adolph, H. Dreher, H. Ott, R. Reber, R. Rieger, B. Schweizer, “T/R-Module for Synthetic Aperture Radar with Polarization Agility,” Proceedings of EUSAR 2004, Ulm, Germany, May 25-27, 2004
93) R. Rieger, B. Schweizer, H. Dreher, R. Reber, M. Adolph, H.-P. Feldle, “Highly Integrated Cost-effective Standard X-Band T/R Module Using LTTC Housing Concept For Automated Production,” Proceedings of. EUSAR 02, Cologne, Germany, June 2002, pp. 303-306
94) A. Herschlein, C. Fischer, H. Braumann, M. Stangl, W. Pitz, R. Werninghaus, “Development and Measurement Results for TerraSAR-X Phased Array,” Proceedings of EUSAR 2004, Ulm, Germany, May 25-27, 2004
95) R. Rieger, H.-P. Feldle, “Advanced T/R Module-Technology for SAR Applications,” Proceedings of EUSAR 2006, Dresden, Germany, May 16-18, 2006
96) W. Pitz, “The TerraSAR-X Satellite,” Proceedings of EUSAR 2006, Dresden, Germany, May 16-18, 2006
97) M. Brandfass, P. Flad, R. Zahn, “Latest Results of the TerraSAR-X Central Electronics,” Proceedings of EUSAR 2006, Dresden, Germany, May 16-18, 2006
98) D. Miller, M. Stangl, R. Metzig, ”On-Ground Testing of TerraSAR-X Instrument,” EUSAR 2006, Dresden, Germany, May 16-18, 2006
99) J. Mittermayer, H. Runge, “Conceptual Studies for Exploiting the TerraSAR-X Dual Receiving Antenna,” IEEE/IGARSS 2003, Toulouse, France, July 21-25, 2003
100) R. Romeiser, H. Breit, M. Eineder, H. Runge, P. Flament, K. de Jong, J. Vogelzang, “On the Suitability of TerraSAR-X Split Antenna Mode for Current Measurements by Along-Track Interferometry,” IEEE/IGARSS 2003, Toulouse, France, July 21-25, 2003
101) R. Romeiser, H. Breit, M. Eineder, H. Runge, ”Demonstration of current measurements from space by along-track SAR interferometry with SRTM data”, in Proceedings of IEEE/IGARSS 2002, Piscataway, N.J., USA, 2002.
102) U. Steinbrecher J. Mittermayer, M. Gottwald, R. Metzig, S. Buckreuß, “New Data Take Commanding Concept for TerraSAR-X Instrument,” Proceedings of EUSAR 2004, Ulm, Germany, May 25-27, 2004
103) S. Lehner, J. Horstmann, J. Schulz-Stellenfleth, “TerraSAR-X for Oceanography: Mission Overview,” Proceedings of IGARSS 2004, Anchorage, AK, USA, Sept. 20-24, 2004
104) J. Mittermayer R. Lord, E. Boerner, “Sliding Spotlight SAR Processing for TerraSAR-X Using a New Formulation of the Extended Chirp Scaling Algorithm,” IGARSS 2003, Toulouse, France, July 21-25, 2003
105) J. Mittermayer, V. Alberga, S. Buckreuss, S. Riegger, “TerraSAR-X: Predicted Performance,” Proc. SPIE 2002, Vol. 4881, Aghia Pelagia, Crete, Greece, September 22-27, 2002.
106) S. Buckreuss, W. Balzer, P. Mühlbauer, R. Werninghaus, W. Pitz, “TerraSAR-X, A German Radar Satellite,” International Radar Symposium (IRS 2003), Dresden, Germany, Sept. 30 - Oct. 2, 2003
107) A. Roth, M. Eineder, B. Schättler, “TerraSAR-X: A New Perspective for Applications Requiring High Resolution Spaceborne SAR Data,” Proceedings of the Joint ISPRS/EARSeL Workshop “High Resolution Mapping from Space 2003,” University of Hannover, Germany, Oct. 6-8, 2003
108) A. Roth, “Scientific Use of TerraSAR-X,” Proceedings of IGARSS 2004, Anchorage, AK, USA, Sept. 20-24, 2004
109) M. Schwerdt, D. Hounam, B. Bräutigam, J.-L. Alvarez-Pérez, “TerraSAR-X: Calibration Concept of a Multiple Mode High Resolution SAR,” Proceedings of IGARSS 2005, Seoul, Korea, July 25-29, 2005
110) M. Schwerdt, D. Hounam, M. Stangl, “Calibration Concept for the TerraSAR-X Instrument,” Proceedings of IGARSS 2003, Toulouse, France, July 21-25, 2003
111) M. Schwerdt, D. Hounam, T. Molkenthin, “Calibration Concepts for Multiple Mode High Resolution SARs like TerraSAR-X,” International Radar Symposium (IRS 2003), Dresden, Germany, Sept. 30 - Oct. 2, 2003
112) R. Lenz, W. Wiesbeck, “The TerraSAR-X active calibration instruments and performance analysis,” Proceedings of IGARSS 2006 and 27th Canadian Symposium on Remote Sensing, Denver CO, USA, July 31-Aug. 4, 2006
113) M. Schwerdt, D. Hounam, J.-L. Alvarez-Pères, T. Molkenthin, “The calibration concept of TerraSAR-X:a multiple-mode, high-resolution SAR,” Canadian Journal of Remote Sensing, Vol. 31, No. 1, 2005, pp. 30-36
114) R. Lenz, W. Wiesbeck, “Overview of the active TerraSAR-X calibrators and first results,” Proceedings of the International Radar Symposium 2007 (IRS 2007), Cologne, Germany, Sept. 5-7, 2007
115) B. Bräutigam, M. Schwerdt, M. Bachmann, “In-flight Monitoring of TerraSAR-X Radar Instrument Stability,” Proceedings of the International Radar Symposium 2007 (IRS 2007), Cologne, Germany, Sept. 5-7, 2007
116) M. Schwerdt, B. Bräutigam, M. Bachmann, T. Molkenthin, D. Hounam, M. Zink, “The Calibration of the TerraSAR-X System,” EUSAR 2006, Dresden, Germany, May 16-18, 2006
117) M. Schwerdt, B. Bräutigam, M. Bachmann, B. Döring, “TerraSAR-X Calibration Results,” Proceedings of EUSAR 2008, 7th European Conference on Synthetic Aperture Radar, June 2-5, 2008, Friedrichshafen, Germany
118) M. Schwerdt, B. Bräutigam, M. Bachmann, B. Döring, D. Schrank, Hueso J. Gonzalez, “Final TerraSAR-X Calibration Results Based on Novel Efficient Methods, IEEE Transactions on Geoscience and Remote Sensing, Vol. 48, Issue 2, 2010, pp. 677-689
119) M. Bachmann, M. Schwerdt, B. Bräutigam, “TerraSAR-X Antenna Calibration and Monitoring Based on a Precise Antenna Model,” IEEE Transactions on Geoscience and Remote Sensing, Vol. 48, Issue 2, 2010, pp. 690-701
120) M. Eineder, H. Runge, E. Boerner, R. Bamler, N. Adam, B. Schättler, H. Breit, S. Suchandt, “SAR Interferometry with TerraSAR-X,” Fringe Workshop 2003, ESA/ESRIN, Frascati, Italy, Dec. 1-5, 2003
121) Information provided by Christoph Reigber of GFZ, Potsdam
122) O. Montenbruck, J. Williams, T. Wang, G. Lightsey, “Preflight Validation of the IGOR GPS Receiver for TerraSAR-X,” May 2, 2005
123) O. Montenbruck, Y. Yoon, E. Gill, M. Garcia-Fernandez, “Precise Orbit Determination for the TerraSAR-X Mission, Proceedings of 25th ISTS (International Symposium on Space Technology and Science) and 19th ISSFD (International Symposium on Space Flight Dynamics), Kanazawa, Japan, June 4-11, 2006, paper: 2006-d-58
124) T. Schwander, R. Lange, H. Kämpfner, B. Smutny, “LCTSX: First On-Orbit Verification of a Coherent Optical Link,” Proceedings of the 5th International Conference on Space Optics, March 30-April 2, 2004, Toulouse, France, ESA SP-554
125) Information of LCT system provided by Rolf Meyer of DLR, Bonn-Oberkassel, Germany
126) B. Smutny, R. Lange, S. Seel, “Optical Terminals for the European ISS Module Columbus Based on Coherent Detection at 1064 nm,” 20th AIAA International Communication Satellite Systems Conference and Exhibit, May 12-15, 2002, Montreal, Quebec, Canada, AIAA 2002-2035
127) R. Barho, M. Schmid, “Coarse Pointing and Fine Pointing mechanism (CPA and FPA) for an optical communication link,” Proceedings of the 10th European Space Mechanisms and Tribology Symposium, Sept. 24-26, 2003, San Sebastián, Spain.
128) F. David, “Atmospheric turbulence monitoring at DLR,” Proceedings of the 11th SPIE International Symposium on Remote Sensing, Sept. 13-16, 2004, Maspalomas, Gran Canaria, Spain. Vol. 5572, 2004, pp. 10-23
129) T. Schwander, “Truly Hermetically Sealed Lasers for Reliable Long Term Space Operation,” 2nd ESA-NASA Working Meeting on Optoelectronics: Qualification of Technologies and Lessons Learned from Satellite LIDAR and Altimeter Missions,” June 21-22, 2006, ESA/ESTEC, Noordwijk, The Netherlands
130) R. Lange, B. Smutny, “Highly-Coherent Optical Terminal Design Status and Outlook,” IEEE LEOS (Laser & Electro-Optics Society) Newsletter, Vol. 19, No, 5, Oct. 2005
131) B. Smutny, “Coherent Laser Communication Terminals,” 14th CLRC (Coherent Laser Radar Conference, Snowmass, CO. USA, July 8-13, 2007
132) Berry Smutny, Hartmut Kaempfner, Gerd Muehlnikel, Uwe Sterr, Bernhard Wandernoth, Frank Heine, Ulrich Hildebrand, Daniel Dallmann, Martin Reinhardt, Axel Freier, Robert Lange, Knut Boehmer, Thomas Feldhaus, Juergen Mueller, Andreas Weichert, Peter Greulich, Stefan Seel, Rolf Meyer, Reinhard Czichy, “5.6 Gbps optical intersatellite communication link,” Proceedings of SPIE, Vol. 7199, San Jose, CA, USA, Jan. 28, 2009, doi:10.1117/12.812209
133) B. Hespeler, R. Braun, D. Dallmann, A. Freier, R. Kurz, “High Performance PowerPC based On-Board Computer Hardware and Software Qualification,” Proceedings of DASIA 2005 (Data Systems in Aerospace), Edinburgh, Scotland, May 30-June 2, 2005
134) S. Buckreuss, P. Mühlbauer, J. Mittermayer, W. Balzer, R. Werninghaus, “The TerraSAR-X Ground Segment,” Proceedings of EUSAR 2006, Dresden, Germany, May 16-18, 2006
135) E. Boerner, R. Lord.., J. Mittermayer., R. Bamler, “Evaluation of TerraSAR-X Spotlight Processing Accuracy based on a New Spotlight Raw Data Simulator,” IEEE/IGARSS 2003, Toulouse, France, July 21-25, 2003
136) R. Werninghaus, “The TerraSAR-X Mission: A German Public-Private Partnership Undertaking,” Proceedings of EUSAR 2006, Dresden, Germany, May 16-18, 2006
The information compiled and edited in this article was provided by Herbert J. Kramer from his documentation of: ”Observation of the Earth and Its Environment: Survey of Missions and Sensors” (Springer Verlag) as well as many other sources after the publication of the 4th edition in 2002. - Comments and corrections to this article are always welcome for further updates (eoportal@symbios.space).
Spacecraft Launch Mission Status Sensor Complement Secondary Payloads Ground Segment References Back to top