FASTSat-HSV
EO
Mission complete
DoD (USA)
In situ
Quick facts
Overview
Mission type | EO |
Agency | DoD (USA) |
Mission status | Mission complete |
Launch date | 20 Nov 2010 |
End of life date | 01 May 2013 |
Instrument type | In situ |
CEOS EO Handbook | See FASTSat-HSV summary |
FASTSat-HSV (Fast Affordable Science and Technology Satellite-Huntsville)
FASTSat-HSV is a small satellite and low-cost mission of the US DoD (Department of Defense) with the goal to demonstrate and test new technologies in space on a rapidly built spacecraft platform. On December 12, 2008, VCSI (Von Braun Center for Science Innovation, Huntsville, AL) and NASA/MSFC (Marshall Space Fight Center, Huntsville, AL) signed a Flight Opportunity Agreement with the STP (Space Test Program) of DoD that enables collaborative projects between the two organizations. In February 2009, the industrial aerospace firm Dynetics of Huntsville teamed up with VCSI and NASA/MSFC to develop FASTSat-HSV. 1) 2) 3)
The objective is to develop and launch a spacecraft bus with six payloads: 4)
• Three multi-agency technology demonstrations from NASA, AFRL (Air Force Research Laboratory), and the Army:
- Threat Detection System
- MST (Miniature Star Tracker)
- NanoSail-D, developed and built by MSFC with key support from the Space and Missile Defense Command in Huntsville and the NASA7ARC (Ames Research Center), Mountain View, CA. NanoSail-D is a reflight due to a launch failure on the third SpaceX Falcon 1 from Omelek Island (Marshall Islands, Pacific).
• Three atmospheric instruments built at NASA/GSFC (Goddard Space Flight Center), Greenbelt, MD, in partnership with the U.S. Naval Academy (USNA), Annapolis, MD.
- TTI (Thermosphere Temperature Imager)
- MINI-ME, a low-energy neutral atom imager to detect neutral atoms formed in the plasma population of the Earth's outer atmosphere to improve global space weather prediction
- PISA (Plasma and Impedence Spectrum Analyzer).

FASTSat-HSV-1, and all six payloads (experiments/instruments) flying on the STP-S26 multi-spacecraft/payload mission, have been manifested for launch in 2010 and have been approved by the DoD Science and Experiments Review Board (SERB).
Spacecraft
A small standardized minisatellite bus is being used which supports NASA's program vision to develop responsive, low-cost civil space science capability enabled by satellite miniaturization and low-cost launchers.
FASTSat-HSV-1 uses a passive ADCS (Attitude Determination and Control Subsystem).
The three partner organizations, NASA/MSFC, Dynetics, and VCSI completed the environmental testing of the FASTSat-HSV spacecraft 10 months from the start of construction. The test will ensure before actual flight that the satellite can withstand the rigors of liftoff and the extreme thermal conditions of space. 5)
The mass of the FASTSat minisatellite is ~180 kg.
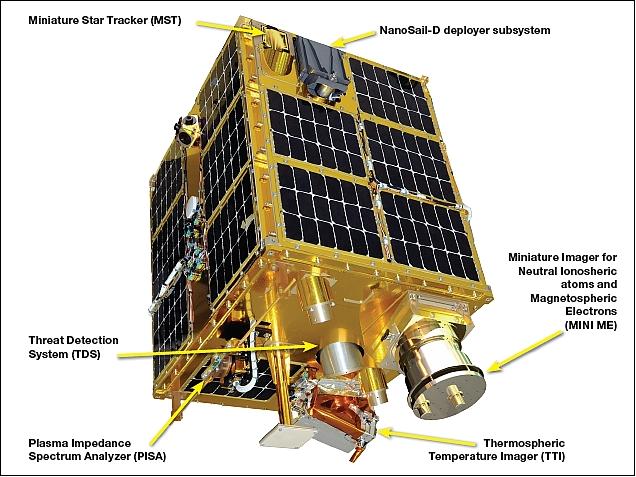
Spacecraft launch mass, size | ~180 kg (minisatellite), approximately 61 cm x 71 cm x 97 cm |
Payload mass | 20-40 kg |
Spacecraft power | 90 W (peak) |
Pointing accuracy | 1º (1 axis), 2º 3 axes) |
Pointing knowledge | 0.02º |
RF communications | S-band, downlink data rate of 1 Mbit/s, on-orbit data storage of 600 MByte |
Payload interfaces | 6 x RS-422 |
Programmable scripts | CubeSat Deployer (P-POD) |
Launch
The FASTSat-HSV minisatellite was launched as a secondary payload on Nov. 20, 2010 (UTC) from Kodiak Island, Alaska, on a Minotaur-4-HAPS (Hydrazine Auxiliary Propulsion System) expandable launch vehicle of OSC. The primary payload of this mission, known as STP-S26, is STPSat-2 (Space Test Program Satellite-2) of DoD, the first satellite based on the STP-SIV (Standard Interface Vehicle). The HAPS capability of precise orbit insertion permits the deployment of small payloads into dual orbits.7) 8) 9)
Note: The multi-payload mission was designated “S26” to correspond to the 26th small launch vehicle mission in STP's 40+year history of flying DoD space experiments. The mission will launch four satellites and three cubesats into low earth orbit. The S26 launch is managed by the Space Development and Test Wing at Kirtland Air Force Base, NM.
This launch of secondary payloads is also the result of an NSF (National Science Foundation) program, the objective is to develop and fly CubeSat-based science missions for space weather and atmospheric research.
Secondary payloads of the mission are:
• FASTSat-HSV-1 (~180 kg)
• O/OREOS (Organism/ORganic Exposure to Orbital Stresses), a NASA/ARC nanosatellite mission of 5.2 kg mass (triple CubeSat). 10)
• FalconSat-5, a microsatellite of USAFA (United States Air Force Academy) at Colorado Springs, CO.
• RAX (Radio Aurora Explorer), a triple CubeSat mission of NSF (National Science Foundation) - a CubeSat-based Ground-to-Space Bistatic Radar Experiment — Radio Aurora Explorer, being developed by a team comprised of University of Michigan and SRI. 11) 12)
• FASTRAC-1 and -2 (Formation Autonomy Spacecraft with Thrust, Relnav, Attitude and Crosslink). FASTRAC is a nanosatellite pair whose design and integration has been undertaken by students of the University of Texas at Austin (UTA). The project is sponsored by AFRL.
Note: All S26 missions have been described separately on the eoPortal.
Orbit: Circular orbit, altitude = 650 km, inclination = 72º, with an orbital period of 97.7 minutes.
Mission Status
• The FASTSAT spacecraft was decommissioned in May 2013, following thirty months of mission operations. 13)
• After two successful years of on-orbit operations, NASA's FASTSAT mission is coming to an end. 14) 15)
• In the fall of 2012, FASTSAT HSV-01 is still in operation (with two extensions), with an expected mission conclusion in November 2012. 16) 17)
• June 20, 2011: The PISA (Plasma Impedance Spectrum Analyzer) instrument has now completed more than 120 days over the course of six months since it was first turned on. That corresponds to more than 1,600 hours of observations and more than 2 GB of raw data taken over a wide range of locations and environments (Ref. 18).
• The TTI (Thermosphere Temperature Imager) has been operating continuously since March 22, 2011. Engineers turned it off for three weeks to protect it from intense heat during the portion of FASTSAT's orbit when it is in continual daylight. 18)
• As of mid-March 2011, the MINI-ME instrument has acquired more than 29 days of observations at optimal performance level. The instrument has flown during periods of magnetic activity and observed neutral atoms over a wide range of geomagnetic levels. 19)
- The TTI (Thermosphere Temperature Imager) continues to operate as planned, detecting FUV (Far Ultraviolet) airglow and FUV auroral signatures. The TTI is on track for achieving full science mode sometime in late April.
- The PISA (Plasma and Impedence Spectrum Analyzer) instrument also continues to operate well. PISA is testing new measurement techniques for the thermal electron populations in the ionosphere, calculating their density structure, which can interfere with or scatter radio signals used for communication and navigation.
• January 26, 2011: During December and January, tests have continued on two of FASTSAT's three onboard science instruments: PISA (Plasma Impedance Spectrum Analyzer) and MIMI-ME (Miniature Imager for Neutral Ionospheric Atoms and Magnetospheric Electrons). Both are operating nominally and are beginning to collect data. 20)
MINI-ME achieved full science mode on January 20, 2011 and has been collecting science data since that time.
• NASA ground stations are tracking the spacecraft and the spacecraft has been activated. The small satellite command center, located at the Huntsville Operations and Science Control Center at NASA's Marshall Space Flight Center in Huntsville, AL, is continuing commissioning operations of the satellite. 21)
Sensor Complement
The experiments/payloads on FASTSat-HSV-1 are cosponsored by NASA and the Department of Defense. TDS and MST are technology demonstration experiments, both are managed by the AFRL (Air Force Research Laboratory) at Kirtland Air Force Base, NM. 22)
Three instruments of the sensor complement, namely PISA, TTI and MINI-ME, were developed in joint collaboration by NASA/GSFC and USNA (US Naval Academy), Annapolis, MD. As part of a rapid-development, low-cost instrument design and fabrication program, these instruments were a perfect match for FASTSAT, which was designed and built in less than one year. These instruments, while independently developed, provide a collaborative view of important processes in the upper atmosphere relating to solar and energetic particle input, atmospheric response, and ion outflow. PISA measures in-situ irregularities in electron number density, TTI provides limb measurements of the atomic oxygen temperature profile with altitude, and MINI-ME provides a unique look at ion populations by a remote sensing technique involving neutral atom imaging. 23)
Together with other instruments and payloads on the STP-S26 mission, such as the NSF RAX mission, FalconSat-5, and NanoSail-D (launched as a tertiary payload from FASTSAT), these instruments provide a valuable "constellation of opportunity" for following the flow of energy and charged and neutral particles through the upper atmosphere. Together, and for a small fraction of the price of a major mission, these spacecraft will measure the energetic electrons impacting the upper atmosphere, the ions leaving it, and the large-scale plasma and neutral response to these energy inputs. The result will be a new model for maximizing scientific return from multiple small, distributed payloads as secondary payloads on a larger launch vehicle (Ref. 23).
TDS (Thread Detection System)
The TDS and the Miniature Star Tracker (MST) are managed by AFRL (Air Force Research Laboratory) at Kirtland AFB, Albuquerque, NM. The objective of the Threat Detection System is to evaluate techniques for measuring the atmospheric propagating characteristics on coherent light generated from known ground stations.

MST (Miniature Star Tracker)
MST is based on the design of CAA (Comtech AeroAstro) - a small, low power (< 2 W, mass = 375 g not including baffle, size: 6 cm x 7.6 cm x 7.6 cm) star tracker, with an accuracy of better than ±90 arcsec in all three axes (1σ). MST is a low-cost COTS version tolerant up to 30 krad. The MST features a user-definable star catalog and powerful hybrid processor. With a 1 Mpixel CMOS array, the star tracker is sensitive up to 4th magnitude stars (Ref. 3). 24)
The MST has a 24º x 30º (Field of View) and a tracking update rate of 1 Hz. The MST is available in a low-cost commercial-off-the-shelf version tolerant up to 30 krad and may be modified for higher radiation tolerance.
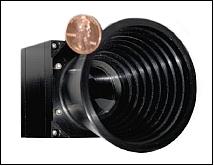
PISA (Plasma and Impedence Spectrum Analyzer)
PISA is an in situ sensor that provides local measurements of electron number density at spatial scales down to 500 m, and electron temperature on 50 km spatial scales. PISA operates over a very wide range of electron densities, from 100 cm-3 to 1.2 x 106 cm-3, providing very accurate measurements of these plasma parameters.
The objective of PISA is to demonstrate a new measurement technique for the thermal electron populations in the ionosphere, and their density structuring, which can interfere with or scatter radio signals used for communication and navigation. The analyzer will determine when and where the ionosphere becomes structured or turbulent, permitting better predictive models of space weather effects on GPS signals.
PISA directly measures the local electron number density and temperature by stimulating a dipole antenna (shown in Figure 5) with a white noise voltage signal, and measuring the antenna-plasma impedance over a frequency range from 10 kHz to 10 MHz. By measuring the complete spectrum of the complex impedance, multiple resonance frequencies can be identified, allowing very precise (1%) determination of the electron plasma frequency and other resonances which depend on the electron temperature. This technique is very suitable for small spacecraft, especially those without three-axis stabilization, as the impedance probe technique is less sensitive to ram/wake variations (Ref. 23).
The instrument can also be further miniaturized below the 3 kg mass developed for FASTSAT, making it a good choice for future nanosatellites and satellite constellations for multipoint measurements of ionospheric structure.

The components of the PISA instrument (Figure 6) demonstrate many of the common design features shared by these three instruments. PISA consists of common design electronics (Low Voltage Power Supply (d), Engineering Data System Board (a), Command and Data Handling Board (c), and Backplane (e)), and instrument-specific components (PISA drive board (b) and sensor (f)).
The power supply engineering data system, Command and Data Handling board, and backplane are the common elements shared with the other instruments (the version of MINI-ME flown on FASTSAT actually uses a different backend, for historical reasons, but a fully functional modular design has been built and tested for MINI-ME, as well).

The modular design included designing and building boards that were large enough to perform the tasks required by all four instruments – e.g. the Command and Data Handling Board has an FPGA with enough resources to handle PISA, which drives the data handling and throughput requirements, and extra circuitry for driving the TTI sensor which is not populated in the implementation of the other three instruments. In addition, the power supplies are only about 55% efficient, due to having to build a single design that worked well for all four instruments.
PISA employs a 6.35 mm diameter dipole antenna that is 70.25 cm long (tip to tip) to measure the antenna-plasma impedance. PISA applies two signals to this antenna: a “DC bias” voltage to counter the effects of spacecraft charging and bring the antenna closer to the ambient plasma potential, in order to minimize the effects of the ion sheath, and a “white noise” signal generated by a shift register pseudo-random number generator. The DC bias voltage can be varied from -2 V to +3 V relative to spacecraft ground. The nominal operating range is +0.5 to +1.25 V.
The PISA instrument contains two additional sensors, for making important contextual and validation measurements. The first of these is a “flat-plate” electrometer system, that holds a 3 cm diameter circular plate in the spacecraft ram direction, and measures the current produced as the spacecraft “scoops up” thermal ions, as it plows through the background ionosphere at ~8 km/s. The measurement range of this system is 60 pA to 2 µA, corresponding to approximately 70 ions / cm3 to 2.3 x 106 ions / cm3.
Unusually for this type of instrument, a linear amplifier instead of a log amp is used. This was designed in order to allow the measurement of very small fluctuations (<= 0.01%) on top of a large background signal, which is not typically possible with log amps. The electrometer is surrounded by a 1 cm thick annular guard, to minimize edge effects. The geometry was chosen to minimize the effects of ion temperature and composition, but the guard thickness is not large enough (it should be several cm, or several Debye lengths), and the project does see variations in ion current (due to different electrostatic attraction for H+ relative to O+) as the electrometer DC bias voltage is varied from -1.25 V to -5 V relative to the spacecraft ground. In any event, the electrometer is sampled at 16 samples/s (500 m spatial resolution) and provides an independent estimate of the electron plasma frequency, fpe.
In addition, for estimating the local magnetic field strength, as well as the angle between the dipole antenna and the magnetic field, PISA contains a Honeywell HMR3400 anisotropic magnetoresistive magnetometer. This unit has a sensitivity of about 100 nT, which means it is not a “science magnetometer” but more a “diagnostic” magnetometer. This sensor is sampled once every 4 seconds, and provides an independent measurement of the electron cyclotron frequency, fce as well as the instrument orientation relative to the Earth’s magnetic field (with ~1º accuracy).
TTI (Thermosphere Temperature Imager)
The three GSFC and USNA instruments, PISA, TTI, and MINI-ME, each has a mass of about 3 kg; the objective is to study different processes in Earth’s upper atmosphere and the effects of solar activity in this region — an important scientific line of inquiry given the fact that intense solar activity can disrupt communications and navigation satellites and exert an aerodynamic drag on LEO (Low Earth Orbiting) satellites and other space assets. This is an area of intense interest to both NASA and DoD. Both are seeking a greater scientific understanding of Earth’s atmosphere and knowledge on how it affects technological systems like GPS and cell phone networks. 25)
TTI is a limb-profiling imager. The objective is to measure spacecraft drag and to provide the first global-scale measurements of thermospheric temperature profiles in the 90-260 km altitude region. The temperature profile sets the scale height of the thermosphere which determines the density profiles at orbital altitudes and therefore the aerodynamic drag experienced by LEO spacecraft. TTI operates both night and day, and fills a large hole in our understanding of thermospheric structuring and density enhancements.

In the dayside ionosphere, where photochemistry is a rapid process, electron density is controlled by O and the molecular species N2 and O2. Consequently, observations of O/N2 variations in the thermosphere show where large electron density variations exist in the ionosphere. Observations of Earth with a broadband FUV imager, and better yet with spectrally distinct FUV bands such as with TTI, can be mapped to changes in the ratio of column density of O to N2.
Figure 8 shows an example of such observations from the broadband FUV sensitive Earth camera of the Polar/Visible Imaging System (VIS). The ionosphere-thermosphere layer is where space weather has its largest impact on society. Knowledge of the temperature, density and extent of the thermosphere is critical for accurate predictions of atmospheric drag on low earth orbiting spacecraft.
TTI remotely measures the temperature profile through observation of the Doppler-broadened line width of the upper atmospheric atomic oxygen emission at 135.6 nm and the molecular nitrogen emission at 135.4 nm. TTI will make simultaneous O and N2 composition and density determinations.
Figure 8 illustrates the typical concept of operations for the TTI instrument with an example of O/N2 observations from Polar VIS. TTI measures the chosen atomic oxygen and molecular nitrogen emissions at the tangent point, and maps these as a function of look angle. The look angle profile can then be mapped to an altitude profile of neutral temperature, neutral density, and neutral composition (O and N2 species).
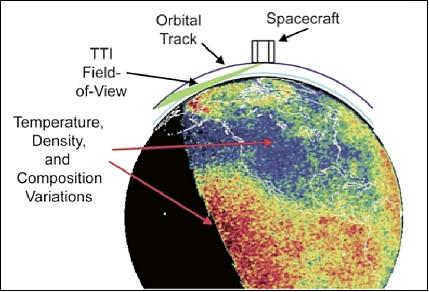
TTI is mounted on the anti-ram side of the spacecraft to minimize ram scouring of the lens coating. TTI consists of two boxes: the optics box (Figure 9) and the electronics box, which contains the shared, modular design in common with PISA. The electronics box is mounted inside the spacecraft, in a stable thermal environment, while the optics box is mounted on the nadir deck, with an unobstructed field of view of the limb, and thermally isolated from the deck so that it can equilibrate to the necessary low temperature to reduce noise in the EMCCD (Electron Multiplying Charge Coupled Device). The nominal operating temperature for the EMCCD sensor is -30ºC, and TTI contains a TEC (Thermoelectric Cooler) that was designed to assist in maintaining the EMCCD at cold temperatures. TTI employs a door that will deploy upon ground command once the spacecraft commissioning is complete, and that protects the instrument from outgassing or other contaminants.
The instrument operation is based on a combination of a fine diffraction grating (2 x the line density of anything flown in space previously), a Fabry-Perot interferometer designed to measure the chosen linewidths (0.0004 nm resolution), and a very sensitive EMCCD for imaging the interference lines as a function of altitude. The whole assembly is thermally isolated, and coupled to a radiator that views deep space, providing temperatures on the orders of -30ºC to minimize EMCCD noise (Ref. 23).

MINI-ME (Miniature Imager for Neutral Ionospheric Atoms and Magnetospheric Electrons)
MINI-ME of NASA/GSFC and USNA is a low energy neutral atom imager (~10 eV to 10 keV). The objective is to observe neutral atoms flowing up from Earth’s ionosphere and into the magnetosphere, the magnetic field that surrounds the planet and protects it from the harmful effects of solar wind. The ENA (Energetic Neutral Atom) imaging technique allows detection of these charged particle populations remotely.
ENAs are produced when an energetic ion (greater than a few eV) undergoes a charge exchange reaction with a cold ionospheric neutral atom. The cold neutral gives up an electron to the ion, neutralizing it, and forming a cold ion and energetic neutral atom. The resulting ENA is no longer confined by the magnetic field, and travels ballistically from the location of the charge exchange reaction. The charge exchange reaction is generally energy- and momentum-conserving, which means that ENAs are emitted at an angle relative to the magnetic field that is consistent with the pitch angle of the parent ion. This process is illustrated in Figure 10. Measurement of ENA fluxes as a function of look angle and energy permits remote sensing of the parent ion population.
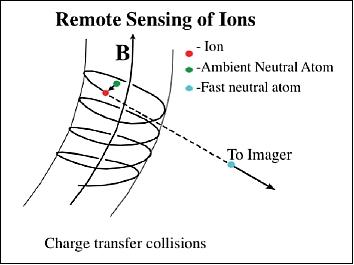
The MINI-ME instrument is heavily based on the highly successful LENA (Low-Energy Neutral-Atom Imager) instrument flown on the IMAGE mission of NASA, which was built at GSFC and returned data from 2000 to 2005. A cross-section of the MINI-ME instrument is shown in Figure 11.
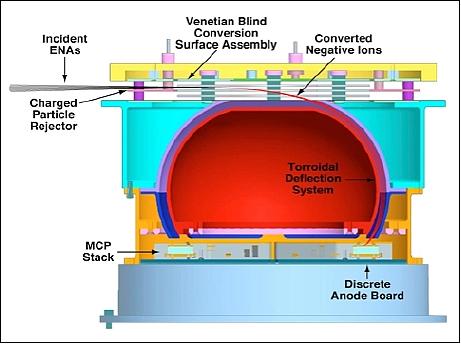
Legend to Figure 11: ENAs enter the instrument, which has cylindrical symmetry around the center axis, through a set of charged particle rejectors, which keep ions and electrons from entering. The neutral atoms then interact at a shallow incidence angle with a highly polished tungsten conversion surface where a small fraction (about 1%) of the incident neutral atoms are converted to negative ions. From this point forward, the instrument operates as a standard electrostatic analyzer, measuring negative ions where the voltage across the toroidal deflection system determines the energy of the incident energetic neutral atom.
The MINI-ME FOV is 10º x 360º, with the 360º azimuthal response divided into six pixels using six sets of microchannel plate stacks. The MINI-ME instrument has a mass of 2.84 kg, and is the smallest of the three GSFC payloads on FASTSat-HSV. The instrument draws about 4.3 W of power when in science mode. The data rate is ~20 Byte/s.
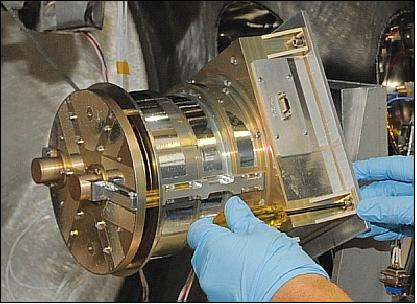
The instrument is gold iridited with portions covered by composite silver conductive coating for thermal control and portions of the internal ion optics plated with black nickel for light suppression. The electronics box at the bottom of the sensors holds three integrated electronics boards: a low voltage power supply board (LVPS), a high voltage power supply board (HVPS), and a command and data handling board (C&DH).
The LVPS uses two VPT DC-DC converters and a filter and also holds the RS422 chip. The HVPS board supplies the high voltages for the microchannel plate stacks, toroids, and ion rejectors, each powered by a separate EMCO C50 power supply and with their levels individually commanded. The C&DH board is based on an MMS (Magnetospheric Multiscale) mission FPI (Fast Plasma Instrument) design using an Aeroflex FPGA and associated electronics including DACs that supply the 0-5 V analog control to the EMCO power supplies and the voltage to the top cap in the upper conversion surface assembly (Ref. 23).
NanoSail-D
NanoSail-D, also referred to as NanoSail-D2, is a technology demonstration experiment with the primary objective to deploy a compact solar sail boom system in space.
In a previous attempt, a team from the NASA/MSFC, along with a team from the NASA/ARC (Ames Research Center), developed a solar sail mission called NanoSail-D which was lost in a launch failure aboard a Falcon 1 rocket on August 3, 2008.
Two units of the NanoSail-D satellites have been built. NASA/MSFC launched the backup NanoSail-D payload from a 3U CubeSat (nanosatellite) of ARC on the FASTSat-HSV mission. FASTSat is required to launch the nanosatellite before the NanoSail-D payload can be launched and deployed from the nanosatellite.
Plans call for the NanoSail-D2 to be deployed from FASTSat-HSV about 7 days after launch.
NanoSail-D consists of a sail subsystem stowed in a 3-element CubeSat. Shortly after deployment of the NanoSail-D, the solar sail will deploy and mission operations will commence. This demonstration flight has two primary technical objectives: 26) 27) 28)
• To successfully stow and deploy the sail
• To demonstrate deorbit functionality.
Note: Although NanoSail-D is a payload of FASTSAT-HSH, the mission of NanoSail-D has been described in a separate file on the eoPortal.

References
1) FASTSat-HSV, December 2008, URL: http://vcsi.org/fastsat.html
2) Steve Cook, Mike Graves, M. Kress, “On Orbit, On Demand Deployment of Nano Satellites for Expanded Mission Capabilities,” Proceedings of the 61st IAC (International Astronautical Congress), Prague, Czech Republic, Sept. 27-Oct. 1, 2010, IAC-10.B4.5.10
3) FASTSat Factsheet, URL: http://www.nasa.gov/centers/marshall/pdf/480065main_FASTSAT_Facts_Final.pdf
4) http://www.dynetics.com/fastsat/index.php?id=mission
5) Turner Brinton, “Government-Industry Team Builds, Tests Satellite in 10 Months,” Space News, January 4, 2010, p. 13
6) http://www.dynetics.com/descriptionpage.php?id=FastsatCapabilities&from=space
7) “NASA's Newest Microsatellite FASTSAT Launches Successfully,” Nov. 20, 2010, URL: http://www.nasa.gov/mission_pages/smallsats/fastsat/10-156.html
8) Dan Belden, “Space Test Program - Access to Space Road-show,” URL: http://www.kiss.caltech.edu/workshops/exoplanet2009/presentations/belden.pdf
9) “FastSat Ready for Shipping to Alaska,” NASA, June 7, 2010, URL: http://www.nasa.gov/topics/technology/features/fastsat-alaska.html
10) John W. Hines, “Nanosatellite Missions in the NASA-Ames Small Spacecraft Division,” Fifth Annual CubeSat Developer’s Workshop, April 11, 2008, Moffett Field, CA, URL: http://mstl.atl.calpoly.edu/~workshop/archive/2008/Spring/Day%203/1120%20-%20Hines%20-%20NASA%20Ames.pdf
11) Therese Moretto Jorgensen, “The NSF CubeSat program : A brief update,” Proceedings of the 2009 CubeSat Developers' Workshop, San Luis Obispo, CA, USA, April 22-25, 2009, URL: https://web.archive.org/web/20131218082223/http://mstl.atl.calpoly.edu/~bklofas/Presentations/DevelopersWorkshop2009/2_Science/5_Jorgensen-NSF.pdf
12) Matt Bennett, “RAX: The Radio Aurora eXplorer,” Proceedings of the 2009 CubeSat Developers' Workshop, San Luis Obispo, CA, USA, April 22-25, 2009, URL: http://mstl.atl.calpoly.edu/~workshop/archive/2009/Spring/Session%203%20-%20Space%20Weather/1545-Bennett-RAX.pdf
13) Mark Boudreaux, Steve Pearson, Joseph Casas, “The Fast, Affordable, Science and Technology Satellite (FASTSAT) Mission,” Proceedings of the 27th AIAA/USU Conference, Small Satellite Constellations, Logan, Utah, USA, Aug. 10-15, 2013, paper: SSC13-X-10, URL: http://digitalcommons.usu.edu/cgi/viewcontent.cgi?article=2982&context=smallsat
14) “NASA's First Minisatellite Mission Comes to Successful End,” NASA, Nov. 29, 2012, URL: http://www.nasa.gov/mission_pages/smallsats/fastsat/12-123.html
15) Lee Roop, “NASA in Huntsville celebrates a breakthrough satellite called FASTSAT,” Nov. 26, 2012, URL: http://blog.al.com/breaking/2012/11/nasa_in_huntsville_celebrates.html
16) Steve Cook, Bill Hughes, Mike Graves, “FASTSAT Orbital Debris Removal Mission - An Affordable, Scalable and Responsive Flight Demonstration,” Proceedings of the 63rd IAC (International Astronautical Congress), Naples, Italy, Oct. 1-5, 2012, paper: IAC-12.A6.7.5
17) Mark Boudreaux, Steve Pearson, Joseph Casas, “FASTSAT a Mini Satellite Mission ......A Way Ahead,” 15th Annual Space & Missile Defense Conference, Huntsville, AL, USA, Aug. 13-16, 2012, URL: http://smdconf.com/T12%20FASTSAT%20a%20mini%20satellite.pdf
18) “FASTSAT Mission Update,” NASA, April 14, 2011, URL: http://www.nasa.gov/mission_pages/sunearth/news/fastsat-update.html
19) Susan Hendrix, “FASTSat Mission Update,” NASA/GSFC, March 11, 2011, URL: http://www.nasa.gov/mission_pages/sunearth/news/fastsat-update.html
20) “FASTSAT Mission Update,” NASA, Jan. 26, 2011, URL: http://www.nasa.gov/mission_pages/sunearth/news/fastsat-update012611.html
21) “NASA's Newest Microsatellite FASTSAT Launches,” Space Daily. Nov. 22, 2010, URL: http://www.spacedaily.com/reports/NASA_Newest_Microsatellite_FASTSAT_Launches_999.html
22) “Three FASTSAT Instruments Pass Tests,” Science Daily, March 11, 2010, URL: http://www.sciencedaily.com/releases/2010/03/100311175047.htm
23) Douglas E. Rowland, Michael R. Collier, John B. Sigwarth, Sarah L. Jones, Joanne K. Hill, Robert Benson, Michael Choi, Dennis Chornay, John Cooper, Steven Feng, Nathaniel Gill, Colby Goodloe, Lawrence Han, Holly Hancock, Floyd Hunsaker†, Noble Jones, John W. Keller, Jeffrey Klenzing, Igor Kleyner, Tom Moore, Keith Ogilvie, Robert Pfaff, Tracy Price, Joe Roman, Marcello Rodruiguez, Paul Rozmarynowski, Mark Saulino, Salman Sheikh, Ken Simms, Alvin Yew, Eric Young, Joseph Kujawski, Mark Boudreaux, Joseph Casas, David Myre, Billy Smith, “Science of Opportunity: Heliophysics on the FASTSAT Mission and STP-S26,” 2011 IEEE Aerospace Conference, Big Sky, MT, USA, March 5-12, 2011
24) “Miniature Star Tracker (MST),” CAA, URL: https://web.archive.org/web/20100416181226/http://www.aeroastro.com/index.php/components/minature-star-tracker-mst
25) Robert J. Gutro, “FASTSAT instruments shipped to NASA Marshall for tests and launch preparation,” NASA, Oct. 26, 2009, URL: http://www.eurekalert.org/pub_releases/2009-10/nsfc-fis102609.php
26) Les Johnson, Roy Young, Edward Montgomery, Dean Alhorn, “Status of the Solar Sail Technology within NASA,” Proceedings of the Second International Symposium on Solar Sailing, New York, NY, July 20-22, 2010, URL: https://web.archive.org/web/20160326185429/http://www.citytech.cuny.edu/isss2010/ISSS2010Proceedingsvx.pdf
27) http://www.nasa.gov/mission_pages/smallsats/nanosaild.html
28) D. C. Alhorn, J. M. Scheierl, “Feathersail - Design, Development and Future Impact,” URL: http://ntrs.nasa.gov/archive/nasa/casi.ntrs.nasa.gov/20100024167_2010023656.pdf
The information compiled and edited in this article was provided by Herbert J. Kramer from his documentation of: ”Observation of the Earth and Its Environment: Survey of Missions and Sensors” (Springer Verlag) as well as many other sources after the publication of the 4th edition in 2002. - Comments and corrections to this article are always welcome for further updates (eoportal@symbios.space).