Jason-1
EO
Atmosphere
Ocean
NASA
Quick facts
Overview
Mission type | EO |
Agency | NASA, CNES |
Mission status | Mission complete |
Launch date | 09 Dec 2001 |
End of life date | 03 Jul 2013 |
Measurement domain | Atmosphere, Ocean, Land, Gravity and Magnetic Fields |
Measurement category | Gravity, Magnetic and Geodynamic measurements, Atmospheric Humidity Fields, Landscape topography, Ocean topography/currents, Ocean surface winds, Ocean wave height and spectrum |
Measurement detailed | Atmospheric specific humidity (column/profile), Land surface topography, Wind speed over sea surface (horizontal), Significant wave height, Geoid, Sea level, Ocean dynamic topography, Gravity field |
Instruments | DORIS-NG, POSEIDON-2 (SSALT-2), LRA, TRSR, JMR |
Instrument type | Precision orbit, Imaging multi-spectral radiometers (passive microwave), Radar altimeters, Atmospheric temperature and humidity sounders |
CEOS EO Handbook | See Jason-1 summary |
Jason-1 (Joint Altimetry Satellite Oceanography Network) Mission
Jason is the name of a joint CNES/NASA oceanography mission series with the objective to monitor global ocean circulation, discover the tie between the oceans and atmosphere, improve global climate predictions, and to monitor events such as El Niño conditions and ocean eddies. The oceanography mission series is considered a cornerstone of GCOS (Global Ocean Observing System), a concept advocated by WMO (World Meteorological Organization), IOC (Intergovernmental Oceanographic Commission of the UNESCO), UNEP (United Nations Environmental Program), and ICSU (International Council of Scientific Unions). 1) 2) 3) 4) 5)
Note: Jason-1 is named after the mythological hero who led the Argonauts on the adventurous and hazardous search for the Golden Fleece which they found and returned. “Jason” symbolizes both the hard-fought quest for a worthy goal and civilization's fascination with the ocean and its mysteries.
Background: In December 1996, CNES and NASA formally agreed in a Memorandum of Understanding (MOU) to jointly participate in the Jason mission to design, build, deploy, and operate a satellite to continue the collection of seasurface elevation measurements originally begun by the TOPEX/Poseidon mission. The TOPEX/Poseidon (T/P) mission, launched in August 1992, was the first such mission in a world-wide effort to study and describe global ocean dynamics and its relationship to the Earth's environment and climate change. The unprecedented success of T/P led mission planners to recognize the need to sustain the high accuracy measurements of sea surface elevation in order to integrate this information into climate models for long-term climate prediction.
Jason-1 was successfully launched in December 2001, and since then is delivering geophysical data at a level of performance identical to the T/P mission (note: the T/P mission ceased operations in the fall 2005 after 13 years of operations). Jason-1 is noticeably different from T/P in that the measurements are no longer experimental in nature; near-real-time products derived from the altimetric measurements are being disseminated on a routine basis. 6)
Spacecraft
The Jason-1 minisatellite carries a radar altimeter, a follow-on mission to the TOPEX/Poseidon mission. Jason-1 is the first spacecraft to use the Proteus minisatellite bus, a multimission platform of CNES/Alcatel Space Industries (partnership). The overall architecture uses redundancy by sharing two half satellites, each under the control of one data handling unit. The satellite consists of the payload module (which accommodates the various instruments) and the Proteus platform, a boxlike structure of 1 m side lengths, which provides on-board services. The hydrazine propellant system uses a 28 l tank with 4 x 1 N thrusters (propulsion is only used for orbit acquisition and maintenance).
The normal in-orbit attitude control is based on a gyro-stellar sensing concept for fine attitude sensing, consisting of two 3-axes star trackers (STR) and three 2-axes gyrometers (GYR). The star trackers, called CALTRAC™, were manufactured by CAL Corp, Canada. The coarse sensor set consists of three-axis magnetometers and eight coarse sun sensors, permitting a 4 pi FOV. Four reaction wheels are used as actuators (they are desaturated using magneto-torquers). Power (450 W) is provided by two solar panels (silicon cells, total array area of 9.5 m2). Power is distributed by a non-regulated primary electrical bus, using a single battery (40 Ah NiCd). S/C mass = 500 kg (270 kg bus dry mass, 180 kg payload mass), the S/C bus size is: 954 mm x 954 mm x 1000 mm, the payload module size is: 954 mm x 954 mm x 1218 mm, S/C design life of 3 years with a goal of 5 years, data storage capacity of 2 Gbit (EOL), S/C pointing accuracy = 0.035º. 7) 8)
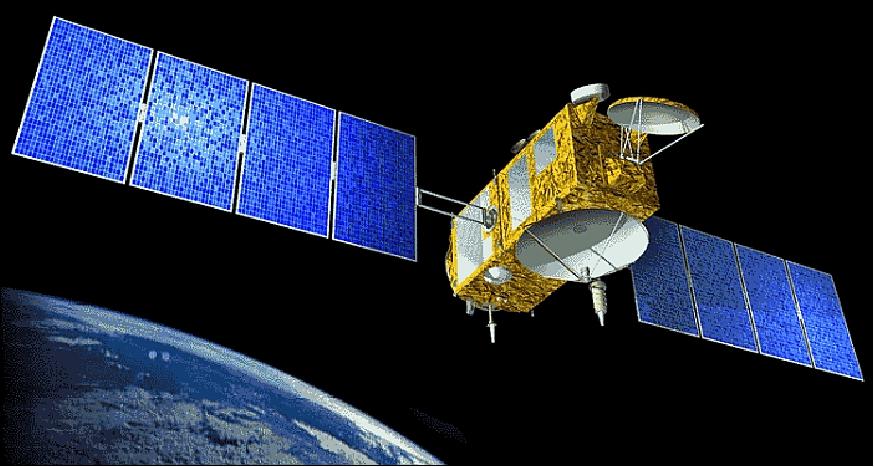

Redundancy concept: The functional redundancies are fully ensured at the satellite level. As far as the hardware is concerned, the equipment units are either one-to-one or “n” out-of “m” redundant (for example: 2 gyros out of 3, 3 reaction wheels out of 4...). However, the most important point to mention is the one to one Processor Module / Data Handling Unit (DHU) redundancy concept referred to satellite’s halves. This concept is illustrated in the Table 1 below showing the shared elements versus the ones separated between the two halves. For instance, it is important to understand that a loss of transmitter “Tx1” will yield to the complete loss of the “A” side even though the Processor Module is still “alive”.
Subsystem | Unit | Nominal | Redundancy | Remarks |
DHS | Data Handling Unit | PMA | PMB | Half Satellite |
Mass memories | MM0, MM1, MM2, MM4 | MM3 cold redundancy | Shared | |
TTCS | TT&C Transmitter | Tx1 | Tx2 | Half Satellite |
TT&C Receiver | Rx1, Rx2 | 1 Rx loss allowed | Hot redundancy | |
AOCS | GPS Receiver | GPS1, 4 or more satellites 99% of the time | GPS2 | Half Satellite |
Reaction Wheels | RW1 to RW4 | 1 RW loss allowed | Shared; no aging effects detected | |
Magneto-Torques | MTB1 to MTB3 nominal coils | MTB1 to MTB3 redundant coils | Half Satellite | |
SA Drive Mechanism | SADM1&2 nominal motor | SADM1&2 redundant motor | Half Satellite; accuracy > 1% | |
Thrusters | THR1 to THR4 | 1 THR loss allowed | Shared (not used in NOM) | |
Gyrometers | GYR1, GYR2 | GYR3 cold redundancy | Shared; GYR3 checked every 6 months | |
Star Trackers | STR1 accurate>99.45% per orbit | STR2 cold redundancy | Shared; STR2 checked periodically | |
Magnetometers | MAG1 No anomaly | MAG2 | Half satellite (not used in NOM; checked every 6 months) | |
Coarse Sun Sensors | CSS1 to CSS8 | 1 CSS loss allowed | Shared (not used in NOM; checked every 6 months), aging effect <2% | |
EPS | Power | 12 section available | 1 section loss allowed | Shared |
Nominal TM/TC | Redundant TM/TC | Half Satellite | ||
Battery Electronic Unit | BEU Nominal | BEU Redundant | Half Satellite | |
Solar Array | 32 strings of 100 cells each | 4 strings loss allowed | Shared (4 strings = 1 panel section) Aging measurement is 0.75% per year versus expected 1.1% | |
Battery | 9 packs of 3 Lithium-ion cells | 1 pack loss allowed | Shared |
Launch
Jason-1 was launched on a Delta-2 7920 vehicle of Boeing from VAFB, CA, on Dec. 7, 2001 (along with the TIMED spacecraft of NASA). The Jason-1 launch was provided by NASA.
Orbit: Circular non-sun-synchronous orbit; 1336 km altitude (2 hour period), inclination = 66.038º, 9.9-day repeat orbits (127 revolutions), ground track repeatability = ±1 km cross-track at the equator. The drift of the orbital plane with respect to the inertial reference frame is -2º per day. The orbit inclination of 66º implies that the coverage of Jason-1 observations is limited to ±66º in latitude.
The Jason-1 operational orbit follows an exact repeat ground track every 127 revolutions in 10 days with the same characteristics than that of Topex/Poseidon (identical orbital tracks (about a minute apart) to perform cross calibration). In this tandem mission setup, Jason-1 is located one minute ahead of Topex/Poseidon. 9)
Jason-1, although a minisatellite, is a true ocean observatory providing SSH (Sea Surface Height) and sea-state measurements in near-real time (within 3 hours) to an international user community. The major science objectives of Jason-1 mission applications are:
• Oceanography and ocean forecasting. An operational application example is the prediction of ocean currents including eddies in ocean circulation spanning distances up to 100 km.
• Climatology and climate prediction. Altimetric data yield vital information for studying and predicting climate, in particular climatic phenomena such as El Niño. Jason-1's ability to measure mean sea level with millimeter accuracy is a key asset for monitoring climate change.
• Marine meteorology and atmospheric studies. Jason-1 delivers sea-state data (wave heights and wind speed) within three hours. This information helps to better understand and predict weather conditions over the oceans.
• Geophysics. The Earth's gravity field affects sea level. By measuring the ocean's dynamic topography, we can learn more about plate tectonics, bottom topography, movements of the Earth's mantle and many other geophysical phenomena. Altimetric data are also used to study ice, lakes and rivers, and relief in desert zones.
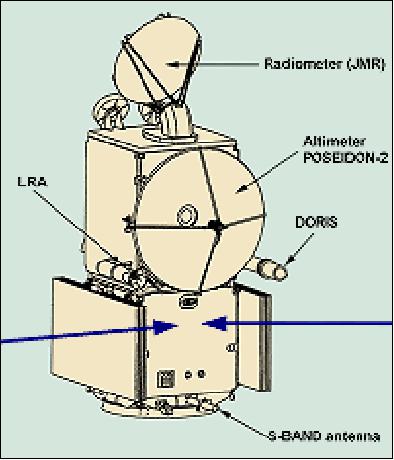
RF communications: downlink data rate at 650 kbit/s (S-band, QPSK modulation), uplink at 4 kbit/s (S-band). The CCSDS communication protocol standard is used in the forward and return link mode (use of virtual channels). Convolutional coding is also applied to telemetry.
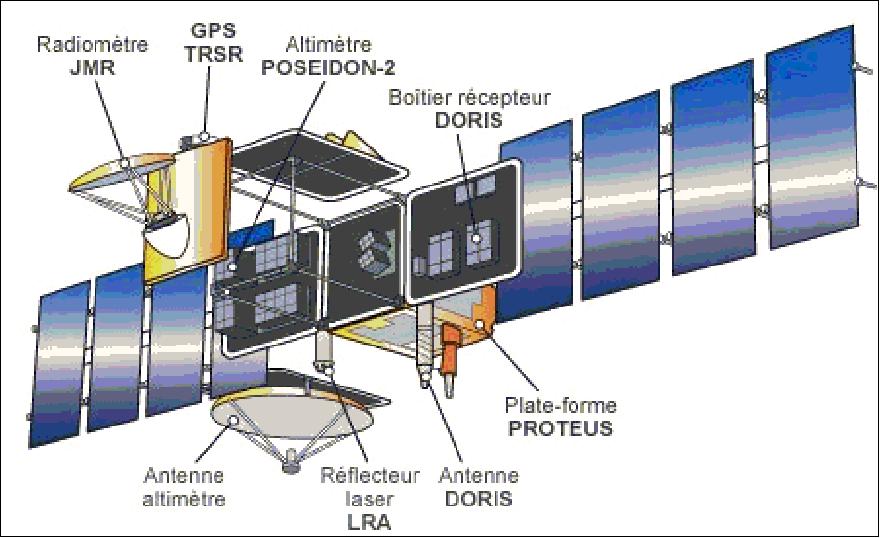
Parameter | Topex/Poseidon | Jason-1 |
S/C mass | 2500 kg | 500 kg |
S/C power | 1000 W | 450 W |
Platform mass | 980 kg | 245 kg |
Platform power | 500 W | 300 W |
Payload mass | 385 kg | 120 kg |
Payload power | 380 W | 147 W |
Altimeter mass | 230 kg | 70 kg |
Altimeter power | 260 W | 78 W |

Mission Status
• Dec. 29, 2015: It has been said that we have more complete maps of the surface of Mars or the Moon than we do of Earth. Close to 70 percent of our planet is covered by water, and that water refracts, absorbs, and reflects light so well that it can only penetrate a few tens to hundreds of meters. To humans and most satellite eyes, the deep ocean is opaque. 12) 13)
- But there are ways to visualize what the planet looks like beneath that watery shroud. Sonar-based (sounding) instruments mounted on ships can distinguish the shape (bathymetry) of the seafloor. But such maps can only be made for places where ships and sonar pass frequently. The majority of such measurements have been made along the major shipping routes of the world, interspersed with results from scientific expeditions over the past two centuries. About 5 to 15 percent of the global ocean floor has been mapped in this way, depending on how you define “mapped.”
- There is another way to see the depths of the ocean: by measuring the shape and gravity field of Earth, a discipline known as geodesy. David Sandwell of SIO (Scripps Institution of Oceanography) and Walter Smith of NOAA (National Oceanic and Atmospheric Administration) have spent much of the past 25 years negotiating with military agencies and satellite operators to allow them acquire or gain access to measurements of the Earth’s gravity field and sea surface heights. The result of their collaborative efforts is a global data set that tells where the ridges and valleys are by showing where the planet’s gravity field varies.

Legend to Figure 6: Shades of orange and red represent areas where seafloor gravity is stronger (in milligal) than the global average, a phenomenon that mostly coincides with the location of underwater ridges, seamounts, and the edges of Earth’s tectonic plates. Shades of blue represent areas of lower gravity, corresponding largely with the deepest troughs in the ocean.

- The maps were created through computer analysis and modeling of new satellite altimetry data from the European Space Agency’s CryoSat-2 and from the NASA/CNES Jason-1, as well as older data from missions flown in the 1980s and 90s. CryoSat-2 was designed to collect data over Earth’s polar regions, but it also collected measurements over the oceans. Jason-1 was specifically designed to measure the height of the oceans, but it had to be adjusted to a slightly different orbit in order to acquire the data needed to see gravity anomalies.
- But how does the height of the sea surface (which is what the altimeters measured) tell us something about gravity and the seafloor? Mountains and other seafloor features have a lot of mass, so they exert a gravitational pull on the water above and around them; essentially, seamounts pull more water toward their center of mass. This causes water to pile up in small but measurable bumps on the sea surface. (If you are wondering why a greater mass would not pull the water down, it is because water is incompressible; that is, it will not shrink into a smaller volume.)
- The new measurements of these tiny bumps on the sea surface were compared and combined with previous gravity measurements to make a map that is two- to four times more detailed than before. Through their work, Sandwell, Smith, and the team have charted thousands of previously uncharted mountains and abyssal hills. The new map gives an accurate picture of seafloor topography at a scale of 5 km/pixel.
- From these seafloor maps, scientists can further refine their understanding of the evolution and motion of Earth’s tectonic plates and the continents they carry. They can also improve estimates of the depth of the seafloor in various regions and target new sonar surveys to further refine the details, especially in areas where there is thick sediment. The map of Figure 8 shows the gravity data as a cartographer would represent the seafloor, with darker blues representing deeper areas.
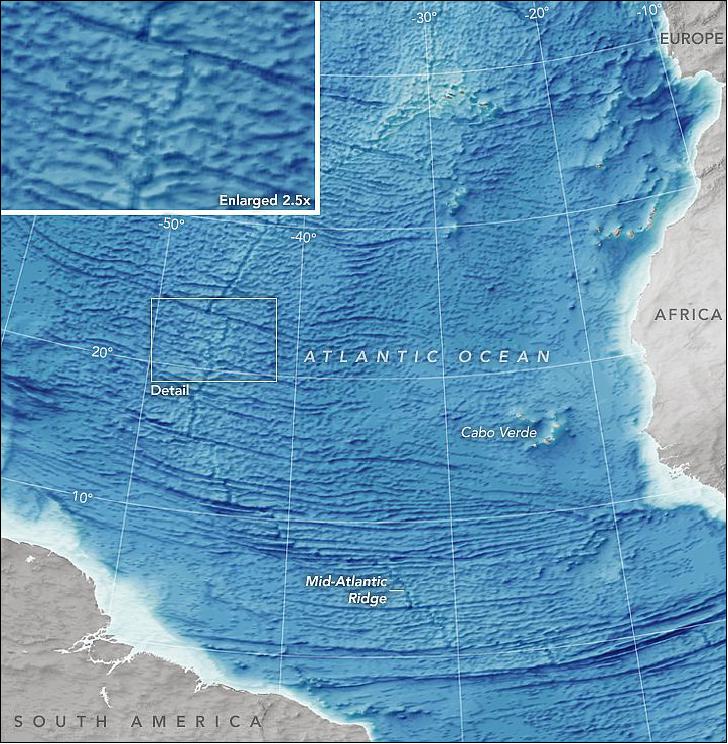
• Spring 2014: Despite the robustness of the platform -initially designed for 3 years- Jason-1 underwent several hardware setbacks over the course of its extended lifetime, most of them due to the expected harsh radiative environment. Some of them could have turned to be life-threatening for its mission, but each time an operational work-around solution has been found to push the envelope a little further. This was made possible thanks to the deep knowledge of the platform by TAS (Thales Alenia Space) and CNES experts, as well as to the awareness, preparedness, reactivity and creativity of the operational teams. 14) 15)
Each time, the operational teams had to think out of the box to find a way to mitigate the issue, either by conveniently using available redundancies, developing software patches, or adapting operational strategies (or a combination of those, most of the time), without compromising on the safety of decommissioning operations.
Another factor to take into account was the sharing of operations between JPL (routine and first step of Safe Hold Mode recovery) and CNES (contingency operations and steps 2/3 of Safe Hold Mode recovery): for these joint operations to work smoothly, it was crucial to put in place simple and robust procedures for emergency operations to be performed by JPL.
Thanks to this fruitful cooperation, these contingencies were almost transparent to the mission, and Jason-1 was able to deliver great science and fully satisfy the scientific community for over 11 years. On top of it, many lessons learned have been taken for the remainder of the ageing PROTEUS fleet (especially for Jason-2 and the upcoming Jason-3): some solutions elaborated on Jason-1 will be directly applicable, or will have to be slightly adapted based on the particular on-board context, and it will be possible to anticipate and act as early as possible when a problem occurs.
• End of the Jason-1 mission in late June 2013 after 11 ½ years of operations: The successful joint NASA/CNES Jason-1 ocean altimetry satellite was decommissioned on July 1, 2013 following the loss of its last remaining transmitter. From 2001 to 2013, Jason-1 provided a major contribution to the monitoring of sea level rise, an essential climate variable. This was due to its excellent measurement accuracy, the long-term stability of its instruments, and the continuous effort of calibration-validation performed on the ground. 16) 17)
- Launched on Dec. 7, 2001, and designed to last three to five years, Jason-1 helped create a revolutionary 20-plus-year climate data record of global ocean surface topography that began in 1992 with the launch of the NASA/CNES TOPEX/Poseidon satellite. For more than 53,500 orbits of our planet, Jason-1 precisely mapped sea level, wind speed and wave height for more than 95 percent of Earth's ice-free ocean every 10 days. The mission provided new insights into ocean circulation, tracked our rising seas and enabled more accurate weather, ocean and climate forecasts.
- Contact was lost with the Jason-1 satellite on June 21, 2013 when it was out of visibility of ground stations. At the time of the last contact, Jason-1 and its instruments were healthy with no indications of any alarms or anomalies. Subsequent attempts to re-establish spacecraft communications from U.S. and French ground stations were unsuccessful. Extensive engineering operations undertaken to recover downlink communications also were unsuccessful.
- After consultation with the spacecraft and transmitter manufacturers, it was determined a non-recoverable failure with the last remaining transmitter on Jason-1 was the cause of the loss of contact. The spacecraft's other transmitter experienced a permanent failure in September 2005. There now is no remaining capability to retrieve data from the Jason-1 spacecraft.
- On July 1, 2013, mission controllers commanded Jason-1 into a safe hold state that reinitialized the satellite. After making several more unsuccessful attempts to locate a signal, mission managers at CNES and NASA decided to proceed with decommissioning Jason-1. The satellite was then commanded to turn off its magnetometer and reaction wheels. Without these attitude control systems, Jason-1 and its solar panels will slowly drift away from pointing at the sun and its batteries will discharge, leaving it totally inert within the next 90 days. The spacecraft will not reenter Earth's atmosphere for at least 1,000 years.
During parts of its mission, Jason-1 flew in carefully coordinated orbits with both its predecessor, TOPEX/Poseidon, and its successor, Jason-2/OSTM ( Ocean Surface Topography Mission), launched in 2008. These coordinated orbit periods, which lasted about three years each, cross-calibrated the satellites, making possible a 20-plus-year unbroken climate record of sea level change. These coordination periods also doubled data coverage.
Combined with data from the ESA's Envisat mission, which also measured sea level from space, these data allow scientists to study smaller-scale ocean circulation phenomena, such as coastal tides, ocean eddies, currents and fronts. These small-scale features are thought to be responsible for transporting and mixing heat and other properties, such as nutrients and dissolved carbon dioxide, within the ocean.
The in orbit Jason-2 mission, operated by the meteorological agencies NOAA and EUMETSAT in collaboration with NASA and CNES, is in good health and continues to collect science and operational data. This same U.S./European team is preparing to launch the next satellite in the series, Jason-3, in March 2015 (Ref. 16).
• The Jason-1 mission is operating nominally in 2013 (extended mission in a drifting geodetic orbit). 18)
• Jason-1 completed 50,000 orbits on September 18, 2012. Jason-1 continues to make an essential contribution to ocean surface topography and to geodesy. 19)
• Jason-1 has been moved to a lower orbit and began its geodetic mission on 7 May 2012. The core payloads were switched ON on May 4th and after some POSEIDON-2 radar (PRF) adjustments, the mission was resumed on May 7th at 15:12:48 UTC. 20) In this new operational phase the Jason-1 mission is in a drifting geodetic orbit. 21)
- No longer on the interleaved track
- Revisit time > 400 days (end of the 10-day exact repeat cycle).
Semi major axis | 7702.437 km |
Eccentricity | 1.3 to 2.8 x 10-4 |
Altitude at equator | 1324.0 km |
Orbital period | 6630 s (or 1 h 52' 10'') |
Inclination | 66.042º |
Cycle | 406 days |
Sub-cycles | 3.9, 10.9, 47.5, 179.5 days |
• April 23, 2012. The Jason-1 project teams at CNES and NASA/JPL have now completed all prerequisite tasks required to enable a safe recovery of the mission from the safehold it has been in since March 3, 2012. 22)
• At the end of February and in early March 2012, Jason-1 encountered an anomaly putting the spacecraft into safehold mode. CNES and NASA management, through the Joint Steering Group, have directed the Jason-1 Project to begin a series of maneuvers to reduce the orbit on a drifting geodetic orbit at 1324.0 km. This orbit is a drifting orbit with a cycle of 406 days and sub-cycles of 3.9 - 10.9 - 47.5 - 179.5 days. 23)
• The Jason-1 mission is operating nominally in 2012. Jason-1 continues to acquire high quality data and the interleaved data of Jason-1 and Jason-2/OSTM are supporting important operational applications and new scientific investigations of mesoscale variability. Additional science contributions will occur when Jason-1 moves to a geodetic orbit (1336 km) to provide estimates of the marine geoid and ocean bottom topography (this may occur after the AltiKa data of the SARAL missionhave been verified by Jason-2/OSTM, sometime in 2012).
In June 2011, the NASA Earth Science Senior Review recommended an extension of the Jason-1 mission as baseline to 2013 and for augmentation to 2015. 24)
• On Dec. 7, 2011, the Jason-1 satellite celebrated 10 years on-orbit, adding to a 20-year continuous satellite record of global sea level rise and monitoring the waxings and wanings of El Nino and La Nina. 25) 26)
• Jason-1 is operating nominally in 2011 (extended mission in its 10th year on-orbit). The Jason-1 retirement depends on the SARAL/AltiKa post-launch readyness date. 27)
Note: The SARAL/AltiKa mission (ISRO and CNES) is scheduled for launch on PSLV-C20 in the spring of 2012.
Jason-1 continues to exceed all Level-1 science requirements, despite:
- Loss of reaction wheel No 1 in November 2003
- Loss of half-satellite (PMB) in September 2005 (Tx)
- Loss of gyro No 1 in March 2010 (Ref. 27) And switch to gyro No 3 in April 2010.
• Jason-1 is operating nominally in 2010 (well beyond its design life). In 2009, a mission extension was given to 2011. 28)
• In late July 2010, an operation of tank emptying was started. For data quality reasons and due to the importance of that mission, the project wants to operate Jason-1 on this operational orbit in conjunction with Jason-2 up to arrival of new oceanography satellites such as SARAL. 29)
• Jason-1 is operating nominally in 2009 (completion of 7th year in orbit on Dec. 7, 2008). However, after providing services on the nominal ground track for 7 years, the Jason-1 satellite was moved to a new interleaved orbit with Jason-2 at the end of repeat cycle 259 (Jan. 26, 2009) - the TOPEX/Poseidon mission used this same ground track in the period 2002-2005. 30) 31)
The Jason-2 satellite will continue the long-term climate data record on the primary TOPEX/Poseidon / Jason-1 / Jason-2 ground track. The interleaving of the Jason-1 with the Jason-2 orbits will provide significant advantages for operational applications.
Repeat cycle 262 (mid-February 2009) was the first Jason-1 cycle on the new interleaved ground track. The new orbit is phased 162º ahead of Jason-2 with a time lag of approximately five days. The first complete science cycle in the new Jason-1 orbit (Cycle 263) started at 04:18 UTC on Feb 20, 2009. Nominal production of all data products in the new orbit resumed on Feb. 14, 2009. 32) 33) 34)
• Current estimates (Nov. 2008) of CNES/Thales are that the Jason-1 spacecraft will provided its services beyond April 2010 with a probability of 77% (Ref. 48).
• Safe hold an recovery: On Aug. 7, 2008, Jason-1 went to a safe hold after experiencing a SEU on a relay leading to reaction wheel #3 (RW3). Recovery was completed on Aug. 13, 2008 and nominal operations were resumed (Ref. 48).
• The Jason-1 spacecraft was kept on the same ground track as TOPEX/Poseidon (launch Aug. 10, 1992) until August 15, 2002. Then, through some orbit maneuvers, TOPEX/Poseidon was slowly shifted to be midway between its former tracks (with only 1 minute separation between the two spacecraft), which it reached on September 20, 2002. This orbital configuration provided interleaved ground tracks with a 1.4º longitude spacing from the Jason-1 tracks - permitting frequent cross-calibrations which resulted in ocean topography data with unprecedented accuracy (better than either S/C could attain by itself). The inter-satellite calibration has determined the relative bias to an accuracy of 1.6 mm. Results from the merging of T/P and Jason-1 data have confirmed the potential of an optimized two-satellite configuration for mesoscale variability studies. - The tandem mission of Jason-1 and TOPEX/Poseidon lasted until Oct. 2005, when the TOPEX/Poseidon mission ended due to a failure in a pitch reaction wheel. 35) 36) 37) 38) 39)
• In Dec. 2004, Jason-1 achieved its primary mission goal of 3 years of operations (mission design life). This is now being followed by the extended Jason-1 mission. The extended mission of Jason-1 is expected to last to overlap with the Jason-2 mission with an expected launch in mid-2008 (to provide a cross-calibration opportunity).
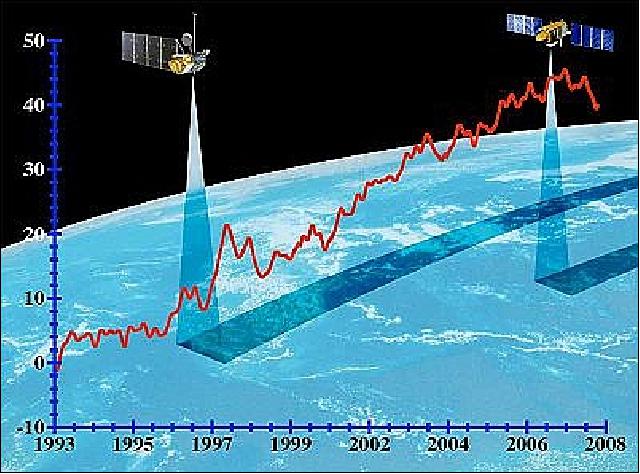
Legend to Figure 9: The global sea level has risen about 3 mm per year since Topex/Poseidon (on the left) began its precise measurement of sea surface height in 1993 and was followed by Jason-1 in 2001. In this Figure, the vertical scale represents the globally averaged sea level (mm). Seasonal variations in sea level have been removed to show the underlying trend.
• On November 19, 2003 at 03:58, Jason-1 transitioned to Safe Hold Mode following the triggering of the onboard “Reaction Wheel Continuity monitoring” FDIR (1Hz monitoring, filter set to 3). — A group of experts was formed to gather all relevant observations and previous analyses performed in-flight or during the development phase, to research the most probable causes, to assess impacts on on-board systems robustness, and proposed recommendations (operational ones on Jason-1, as well as for the development of Proteus satellites at that time, Ref. 14).
Sensor Complement
The payload module provides mechanical, electrical, thermal and dynamical support to the Jason instruments.
Poseidon-2 (Solid-State Radar Altimeter)
Poseidon-2 is a dual-frequency nadir-looking radar altimeter with the objective to map the topography of the sea surface for calculating ocean surface current velocity and to measure ocean wave height and wind speed. Poseidon-2 is a CNES-sponsored instrument designed and developed by Alcatel Alenia Space (formerly Alcatel Espace) as prime contractor. Poseidon-2 is of SSALT/Poseidon-1 heritage on TOPEX/Poseidon.
The Poseidon-1 instrument is being upgraded for the Jason radar altimeter mission by adding a second C-band frequency of 5.3 GHz (Ku-band at 13.575 GHz), as well as changing to digital technology and using a new radiation-hardened microprocessor (the altimeter electronics are split into two boxes, the PCU (Processing & Control Unit), and the RFU (Radio Frequency Unit). The PCU includes a chirp generator, baseband demodulator, spectrum analyzer, instrument control unit and interfaces. The RFU performs the up-conversion to Ku- and C-bands, the high power solid-state amplification, the low-noise amplification of the received echoes, and its mixing with a reference chirp. The C-band channel provides direct ionospheric correction for the primary Ku-band measurement and uses a 1.2 m antenna on the nadir side of the spacecraft. 40) 41) 42) 43)
Instrument parameters: transmitted pulse width of 105 μs; PRF of 2100 Hz (1800 for Ku-band and 300 for C-band); maximum RF output power to antenna of 38.4 dBm (Ku-band) and 42 dBm (C-band); instrument mass = 70 kg for dual-frequency configuration; duty cycle = 100%; power = 78 W, thermal control by conduction to mounting surface and by radiation within the instrument; thermal operating range = -5 to -35ºC; IFOV = 20º cone centered at nadir.
The major evolutions of the Poseidon-2 altimeter with respect to SSALT/Poseidon-1 are:
• Addition of the second frequency (C Band) to obtain direct ionospheric correction.
• The digital chirp generator to allow a selectable bandwidth necessary for the C band channel and a better phase knowledge
• Increase of the emitting power to keep an acceptable link budget (the size of the antenna has been decreased with respect to Topex/Poseidon)
• A 128 points FFT transform to obtain a better mispointing evaluation and then reduce the pointing requirements
• Use of a rad hard microprocessor to provide a better radiation tolerance and thus increase the operational availability of the sensor. This microprocessor is also more powerful to increase the on-board processing capability of the altimeter.
Transmission frequencies | 5.3 GHz (C-band), 13.575 GHz (Ku-band) |
Transmitted pulse width | 105.6 μs |
Bandwidth | 320 MHz (Ku-band), 320/100 MHz (C-band) |
PRF (Puls Repetition Frequency) | 1800 Hz (Ku-band), 300 Hz (C-band) |
Peak output power | 8 W for Ku-band, 25 W for C-band |
Max. RF power output to antenna | 38.4 dBm Ku-band, 42 dBm for C-band |
Noise figure | 3.2 dB (Ku-band), 0.9 dB (C-band) |
Data rate | 22.5 kbit/s including waveform data and onboard parameters |
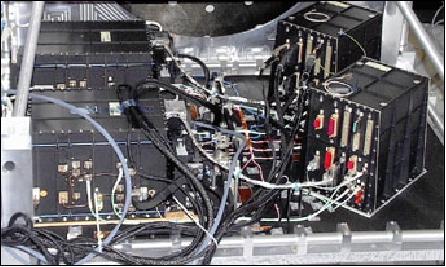

JMR (Jason Microwave Radiometer)
JMR is a JPL instrument of TMR heritage. JMR is a passive microwave radiometer measuring the brightness temperatures in the nadir column at 18.7, 23.8, and 34 GHz, providing path delay correction for the altimeter (the brightness temperatures are converted to path-delay information). The 23.8 GHz channel is the primary water vapor sensor, the 34 GHz channel provides a correction for non-raining clouds, and the 18.7 GHz channel provides the correction for effects of wind-induced enhancements in the sea surface background emission. 44) 45) 46)

The JMR receivers employ MMIC (Microwave Monolithic Integrated Circuit) technology (built by TRW) for high reliability, low power and low mass. Three temperature controlled noise diodes are used for gain calibration in all the radiometers. JMR consists of a collecting aperture shared with Poseidon-2, a multifrequency feed assembly that illuminates the collecting aperture, multichannel microwave receivers, a data unit, power supplies, and ground support equipment. Instrument parameters: beamwidth = 1.2º at 18.7 GHz, 1.0º at 23 GHz, and 0.7º at 34 GHz; temperature resolution <1 K; system temperature = 800 K; mass=27 kg; power = 31 W; data rate = 100 bit/s; thermal control by conduction through the mounting feet of the satellite structure; thermal operating range = 5-35ºC.
DORIS (Doppler Orbitography and Radiopositioning Integrated by Satellite)
DORIS is a CNES/Thomson development. DORIS is a precision orbit determination system providing position and ionospheric correction for Poseidon-2. Doris measurements are also used for geophysical studies, in particular through the International Doris Service (IDS). Doris is a dual-frequency instrument able to determine atmospheric electron content.
The DORIS flight segment consists of a two-channel, two-frequency (401.25 MHz and 2036.25 MHz) Doppler receiver capable of tracking signals from a worldwide network of about 50 ground beacons. The Jason DORIS receiver is the same second generation device as the one developed for the ENVISAT mission. Its main functional improvements over first-generation receivers are its capability to receive two beacons simultaneously and to produce onboard the orbit ephemeris in real time with a precision of 1 m. The receiver is controlled by an ultra-stable oscillator delivering the reference frequency with a stability of 5 x 10-13 over a 10-100 second interval and delivering an on-board time output within 0.1 ms accuracy. The DORIS instrument mass is 31 kg, power = 30 W.

BlackJack (GPS Flight Receiver)
BlackJack is also referred to as TRSR-2 (Turbo Rogue Space Receiver-2). The instrument is of GPS/MET (Microlab) heritage (of a design as flown on CHAMP) and is being provided by NASA/JPL and built by Spectrum Astro Inc. of Gilbert, AZ. BlackJack is a 16-channel GPS receiver with the objective to provide supplementary positioning data to DORIS in support of the POD (Precision Orbit Determination) function and to enhance and/or improve gravity field models. Radial accuracies of 1-2 cm are obtained in post-processing. BlackJack is a fully redundant unit (two independent receivers operating in cold redundancy). Each unit is comprised of an omnidirectional antenna, low-noise amplifier, crystal oscillator, sampling down-converter, and a baseband digital processor assembly, communicating through a 1553 bus interface. Instrument mass = 10 kg (2), power = 17.5 W.
In its current configuration, the BlackJack on Jason-1 can track up to 12 GPS satellites simultaneously in dual-frequency mode. From these signals, BlackJack acquires measurements of the GPS carrier phase providing range measurements with an accuracy of about 1 mm; the absolute pseudo range (defined as the absolute range plus receiver time offset from GPS time) has an accuracy of about 10 cm. BlackJack provides also onboard solutions for S/C position and time, accurate to about 50 m and 150 ns, respectively. 47)
Note. As fall of 2008, the life expectancies of the two TRSR receivers has been surpassed. 48)
- TRSR-2 (primary receiver) operates in a degraded mode, but still supports orbit determination (with significant reduced accuracies)
- The TRSR2 (primary receiver) experienced a critical failure during nominal operations on April 2009 and will also remain powered off (Ref. 30).
- TRSR-1 (redundant receiver) experienced a critical failure on Sept. 13, 2008 and is no longer usable.
The TRSR instruments were experimental and were not mission critical.

LRA (Laser Retroreflector Array)
a JPL instrument of TOPEX/Poseidon heritage, built by ITE Inc. under NASA/GSFC contract. LRA provides a reference target for satellite laser ranging (SLR) measurements, which are necessary to calibrate the POD system and the altimeter throughout the mission. The LRA is placed on the nadir face of the satellite. It is a totally passive unit that consists of nine quartz corner cubes arrayed as a truncated cone with one in the center and the other eight distributed azimuthally around the cone. This arrangement allows laser ranging at FOV (Field-of-View) angles of 360º in azimuth and 60º elevation around the perpendicular. The retroreflectors are optimized for a wavelength of 532 nm (green), offering a FOV of about 100º. The LRA instrument mass is 2.2 kg.

The LRA is a passive instrument that acts as a reference target for laser tracking measurements performed by ground stations. Laser tracking data are analyzed to calculate the satellite's altitude to within a few millimeters. However, the small number of ground stations and the sensitivity of laser beams to weather conditions make it impossible to track the satellite continuously. That is why other onboard location systems are needed.
Ground Segment
The Jason-1 ground segment is made up of three components providing the human (teams) and equipment resources required to ensure the mission's success: 49) 50)
• PGGS (Proteus Generic Ground Segment), based in Toulouse, France
• POCC (Project Operation Control Center), based in Pasadena, California, USA
• SSALTO (Altimetric and Orbitography Multi-Mission Center), Altimetric and Orbitography Mission Center.
The Jason-1 spacecraft has been monitored and controlled from CNES in Toulouse up to the end of the assessment phase (first 30/50 days of the mission). - Then the POCC (Project Operations Control Center) of JPL took over.
The baseline ground network includes stations at Aussaguel (France), Fairbanks (AK, Poker Flats location), Wallops Island, VA, and Hartebeesthoek, South Africa. In addition, the Altimetric and Orbitography Mission Center (SSALTO), located in Toulouse and the Jason Science Data System at JPL in Pasadena, form the mission ground segment.
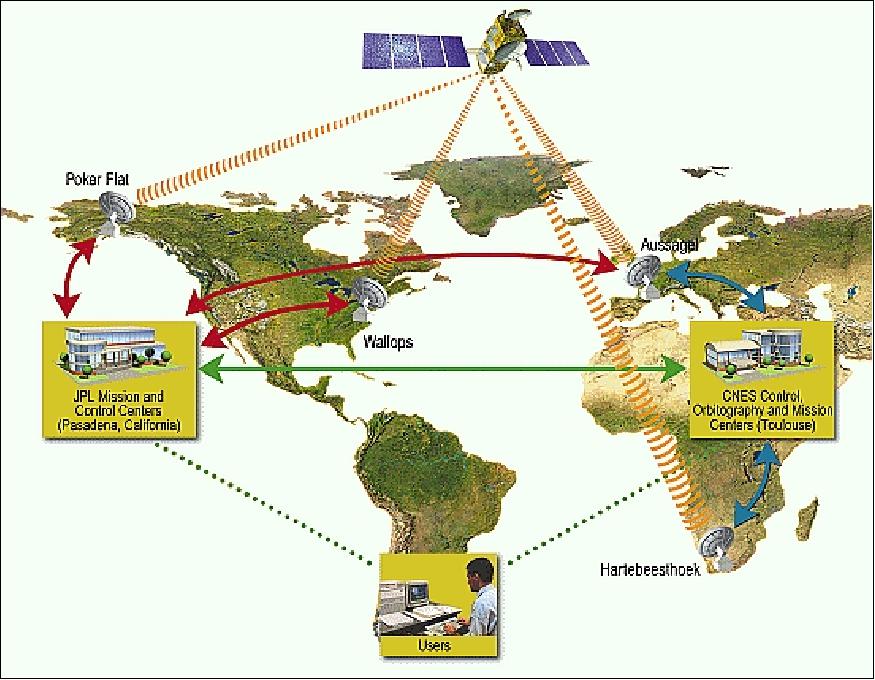
Major data products of the Jason-1 mission include:
• Sea surface topography
• Significant wave height
• Surface wind speed
• Ocean tides
• Vertically integrated atmospheric water vapor
• Vertically integrated ionospheric electron content
References
1) M. Lefebvre, “A New Voyage for Jason,” CNES/AVISO Newsletter Nr. 5, April 1997
2) F. Parisot, T. Lafon, “The JASON-1 Satellite Design and Development Status,” Proceedings of the 4th International Symposium on Small Satellites Systems and Services, Sept. 14-18, 1998, Antibes Juan les Pins, France
3) P. Escudier, G. Kunstmann, F. Parisot, R. Boain, T. Lafon, P. Hoze, S. Kaki, “Jason System Overview and Status,” URL: http://www.aviso.oceanobs.com/en/newsstand/newsletter/newsletter07/jason-system-overview-and-status/index.html
4) http://sealevel.jpl.nasa.gov/missions/jason1/
5) T. Lafon, F. Parisot, “The Jason-1 satellite design and development status,” Proceedings of the AIAA/USU Conference on Small Satellites, Logan, UT, Aug./Sept. 1998, SSC98-V-6
6) F. Parisot, “Satellite Altimetry: Current Status and Perspectives,” Proceedings of the 2005 EUMETSAT Meteorological Satellite Conference,” Dubrovnik, Croatia, Sept. 19-23, 2005, pp. 373-379
7) T. Lafon, “Jason-1: Lessons Learned from the Development and 1 Year in Orbit,” 4th IAA Symposium on Small Satellites for Earth Observation, Apr. 7-11, 2003, Berlin, Germany
8) M. Sghedoni, J. L. Beaupellet, et al. “Proteus/Jason-1 AOCS Flight Results,” 5th ESA Conference on Guidance Navigation and Control Systems, Oct. 22-25, 2002, Frascati, Italy, ESA SP-516, pp. 457-464
9) C. Salcedo, “Jason Orbit Acquisition and First Results of Station Keeping,” Proceedings of International Symposium on Formation Flying, Oct. 29-31, 2002, Toulouse, France
10) Y. Menard, Ph. Escudier, “Cruising the Ocean from Space with Jason-1,” EOS/AGU Transactions, Vol. 81, No 34, Aug. 22, 2000, p. 1 and pp. 390-391
11) “Mission /Program Status,” OSTST (Ocean Surface Topography Science Team) 2012 meeting presentations, Sept. 27, 2012, Venice, Italy, URL of presentation: http://www.aviso.oceanobs.com/fileadmin/documents/
OSTST/2012/oral/01_thursday_27/01_general/02_program_status_Parisot.pdf
12) ”Seafloor Features Are Revealed by the Gravity Field,” NASA Earth Observatory, Dec. 29, 2015, URL: http://earthobservatory.nasa.gov/IOTD/view.php?id=87189
13) David T. Sandwell, R. Dietmar Müller, Walter H. F. Smith, Emmanuel Garcia, Richard Francis, ”New global marine gravity model from CryoSat-2 and Jason-1 reveals buried tectonic structure,” Science, Oct. 3, 2014, Vol. 346 No 6205, pp: 65-67, DOI: 10.1126/science.1258213
14) Rémi Canton, Pierre Pélipenko, “JASON-1 (NASA-JPL/CNES) : A Successful Operational Story Throughout Hardware Ageing,” SpaceOps 2014, 13th International Conference on Space Operations, Pasadena, CA, USA, May 5-9, 2014, paper: AIAA 2014-1609, URL: http://arc.aiaa.org/doi/pdf/10.2514/6.2014-1609
15) Claude Audouy, Thierry Guinle, Glenn Shirtliffe, “JASON-1: Orbit change to combine end-of-life safety and new science objectives,” SpaceOps 2014, 13th International Conference on Space Operations, Pasadena, CA, USA, May 5-9, 2014, paper: AIAA 2014-1718, URL: http://arc.aiaa.org/doi/pdf/10.2514/6.2014-1718
16) Steve Cole, Alan Buis, Julien Watelet, “Long-Running NASA/CNES Ocean Satellite Takes Final Bow,” NASA Release 13-206, July 3, 2013, URL: http://www.nasa.gov/press/2013/july/long-running-nasacnes-ocean-satellite-takes-final-bow/#.UdREOdhi0gg
17) Alain Delrieu, Julien Watelet, Juliette Lambin, CNES Press Release, PR042-2013, July 3, 2013, “Jason-1 altimetry satellite stops after more than 11 years of continuous ocean monitoring,” URL: http://www.cnes.fr/automne_modules_files/pPressReleases/public/r7549_81_cp042-2013_-_jason-1_stops.pdf
18) “Jason-1,” O.S.C.A.R., http://www.wmo-sat.info/oscar/satellites/view/204
19) Glenn Shirtliffe, Thierry Guinle, “Jason-1 Project Status,” OSTST (Ocean Surface Topography Science Team) 2012 meeting, Sept. 27, 2012, Venice, Italy, URL: http://www.aviso.oceanobs.com/fileadmin/documents/
OSTST/2012/oral/01_thursday_27/01_general/03_jason1_status_Shirtliffe.pdf
20) “New Jason-1 Geodetic Mission,” NASA/JPL, May 14, 2012, URL: http://podaac.jpl.nasa.gov/announcements/2012-05-14_PODAAC_Jason-1_Geodetic_Mission
21) D. Sandwell, G. Dibarboure, “Future scientific outlook for Jason-1: Geodesy and Oceanography,” OSTST (Ocean Surface Topography Science Team) 2012 meeting presentations, Sept. 27, 2012, Venice, Italy, URL: http://www.aviso.oceanobs.com/fileadmin/documents/OSTST/2012/oral/
01_thursday_27/01_general/09_Dibarboure.pdf
22) “Jason-1 coming out of safehold and entering a new mission on May 4, 2012,” NASA/JPL, April 25, 2012, URL: http://podaac.jpl.nasa.gov/announcements/2012-04-25_PODAAC_Jason-1_Coming_Out_of_Safehold_and_Entering_New_Mission_4_May_2012
23) http://www.aviso.oceanobs.com/en/missions/current-missions/jason-1/index.html
24) George Hurtt (Chair), Ana Barros, Richard Bevilacqua, Mark Bourassa, Jennifer Comstock, Peter Cornillon, Andrew Dessler, Gary Egbert, Hans-Peter Marshall, Richard Miller, Liz Ritchie, Phil Townsend, Susan Ustin,“NASA Earth Science Senior Review 2011,” June 30, 2011, URL: http://science.nasa.gov/media/medialibrary/2011/07/22/2011-NASA-ESSR-v3-CY-CleanCopy_3x.pdf
25) “Jason-1 Achieves a One-Decade Landmark,” Space Daily, Dec. 9. 2011, URL: http://www.spacedaily.com/reports/Jason_1_Achieves_a_One_Decade_Landmark_999.html
26) Glenn Shirtliffe, “Jason-1 Project Status,” OSTST (Ocean Surface Topography Science Team) Meeting, San Diego, CA, USA, Oct. 19-21, 2011, URL: http://www.aviso.oceanobs.com/fileadmin/documents/
OSTST/2011/oral/01_Wednesday/Plenary/Program%20Status/07%
20JA1-OSTST2012-Shirtliffe-final.pdf
27) Glenn M. Shirtliffe, “Jason-1 Project Status,” 2010 OSTST (Ocean Surface Topography Science Team) meeting, Lisbon, Portugal, Oct. 18-20, 2010, URL: http://www.aviso.oceanobs.com/fileadmin/documents/OSTST/2010/oral/Shirtliffe.pdf
28) Steven A. Ackerman (chair), Richard Bevilacqua, Bill Brune, Bill Gail, Dennis Hartmann, George Hurtt, Linwood Jones, Barry Gross, John Kimball, Liz Ritchie, CK Shum, Beata Csatho, William Rose, Carlos Del Castillo, Cheryl Yuhas, “NASA Earth Science Senior Review 2009,” URL: http://nasascience.nasa.gov/about-us/science-strategy/senior-reviews/2009SeniorReviewSciencePanelReportFINAL.pdf
29) Bernard Cabrières, Fernand Alby, Christian Cazaux, “Satellite end of life constraints : technical and organisational solutions,” Proceedings of the 61st IAC (International Astronautical Congress), Prague, Czech Republic, Sept. 27-Oct. 1, 2010, IAC-10.B6.2.7
30) Glenn M. Shirtliffe, “Jason-1 Project Status,” Proceedings of the OSTST (Ocean Surface Topography Science Team) 2009 meeting, Seattle WA, USA, June 22-24, 2009, URL: http://www.aviso.oceanobs.com/fileadmin/documents/OSTST/2009/oral/Shirtliffe.pdf
31) http://sealevel.jpl.nasa.gov/missions/jason1/
32) “January 2009: Duacs switches from Jason-1 to Jason-2 as a reference mission,” AVISO, URL: http://www.aviso.oceanobs.com/en/data/product-information/duacs/presentation/updates/index.html#c6778
33) “Jason-1 on its new orbit,” AVISO, Feb. 18, 2009, URL: http://www.aviso.oceanobs.com/en/news-storage/news-
detail/index.html?tx_ttnews[tt_news]=450&tx_ttnews[backPid]=285&cHash=kgoqlpqvhb
34) G. Zaouche, “Jason-1 Orbit change preparation,” 2008 OSTST (Ocean Surface Topography Science Team Meeting) Meeting, Nice, France, Nov. 10-12, 2008, URL: http://www.aviso.oceanobs.com/fileadmin/documents/OSTST/2008/oral/zaouche_orbit_change.pdf
35) “Good News from Jason‐1,” CNES Magazine, No 15, April, 2002, p. 13
36) P. Y. Le Traon, G. Dibarboure, “An illustration of the unique contribution of the Topex/Poseidon - Jason-1 tandem mission to mesoscale variability studies,” URL:
http://www.aviso.oceanobs.com/fileadmin/documents/kiosque/newsletter/news10/letraon_uk.pdf
37) L.-L. Fu, D. Stammer, R. R. Leben, D. B. Chelton, “Improved Spatial Resolution of Ocean Surface Topography from the T/P-Jason-1 Altimeter Mission,” EOS Transactions of AGU, Vol. 84, No. 26, 2003, p. 241
38) http://www.aviso.oceanobs.com/en/missions/past-missions/topexposeidon/index.html
39) Information provided by Vinca Rosmorduc of AVISO, Toulouse, France
40) “Jason-1,” ESE Reference Handbook of NASA, 1999, pp. 120-123
41) L. Rey, G. Carayon, et al., “Poseidon-2, the new generation altimeter for JASON mission,” Proceedings of IGARSS'99, Hamburg, Vol. III, June 28-July 2, 1999, pp. 1503-1505
42) L. Phalippou, E. Caubet, L. Rey, P. Calvary, D. Murat, J. Richard, G. Angino, E. Thouvenot, G. Carayon, N. Steunou, C. Mavrocordatos, P. Escudier, “25 Years of Altimeter Development at Alcatel Alenia Space,” Symposium: 15 Years of Progress in Radar Altimetry, Venice, Italy, March 13-18, 2006
43) https://web.archive.org/web/20081121190804/http://www.altimetry.info/html/missions/jason1/instruments/welcome_en.html
44) Shannon Brown, Chris Ruf, Steve Keim, Ami Kitiyakara, “Jason Microwave Radiometer Performance and On-Orbit Calibration,” Marine Geodesy, Volume 27, Numbers 1-2, Numbers 1-2/2004 , pp. 199-220
45) C. Ruf, “Jason-1 Microwave Radiometer on Orbit Validation,” URL: http://sealevel.jpl.nasa.gov/science/ostscienceteam/scientistlinks/scientificinvestigations/ruf/
46) Shannon T. Brown, Shailen Desai, Wenwen Lu, Alan B. Tanner, “On the Long-Term Stability of Microwave Radiometers Using Noise Diodes for Calibration,” IEEE Transactions on Geoscience and Remote Sensing, Vol. 45, No 7, July 2007, pp.1908-1920
47) B. Haines, W. Bertiger, S. Desai, Da Kuang, “Initial Orbit Determination Results for Jason-1: Towards a 1 cm Orbit,” ION-GPS 2002, Portland, OR, Sept. 24-27, 2002
48) Glenn M. Shirtliffe, Jason-1 JPL Project Status,” 2008 OSTST (Ocean Surface Topography Science Team) Meeting, Nice, France, Nov. 10-12, 2008, URL: http://www.aviso.oceanobs.com/fileadmin/documents/OSTST/2008/oral/shirtliffe.pdf
49) “Jason-1 ground segment,” URL: http://www.aviso.oceanobs.com/en/missions/current-missions/jason-1/ground-segment/index.html
50) S. Coutin-Faye, J. Noubel, G. Boutonnet, “SSALTO: A new ground segment for a new generation of altimetry satellites,” URL: http://www.aviso.oceanobs.com/en/newsstand/newsletter/newsletter07/ssalto-new-ground-segment/index.html
The information compiled and edited in this article was provided by Herbert J. Kramer from his documentation of: ”Observation of the Earth and Its Environment: Survey of Missions and Sensors” (Springer Verlag) as well as many other sources after the publication of the 4th edition in 2002. - Comments and corrections to this article are always welcome for further updates (eoportal@symbios.space).