Landsat-7
EO
Atmosphere
Ocean
Cloud type, amount and cloud top temperature
Launched in April 1999, Landsat-7 is part of NASA’s Earth Science Enterprise (ESE) program, a joint venture by NASA and USGS (United States Geological Survey). As a successor of Landsat-6, which failed upon launch, the objective of Landsat-7 is to enhance the medium-resolution multispectral imagery of Earth’s continental surfaces provided by previous Landsat satellites. Although it had an initial design life of seven years, Landsat-7 remains operational as of July 2022 and was joined by Landsat-8 in February 2013, and Landsat-9 in September 2021.
Quick facts
Overview
Mission type | EO |
Agency | NASA, USGS |
Mission status | Operational (extended) |
Launch date | 15 Apr 1999 |
Measurement domain | Atmosphere, Ocean, Land, Snow & Ice |
Measurement category | Cloud type, amount and cloud top temperature, Ocean colour/biology, Multi-purpose imagery (ocean), Radiation budget, Multi-purpose imagery (land), Surface temperature (land), Vegetation, Albedo and reflectance, Sea ice cover, edge and thickness, Snow cover, edge and depth, Inland Waters |
Measurement detailed | Ocean imagery and water leaving spectral radiance, Ocean chlorophyll concentration, Cloud cover, Cloud imagery, Land surface imagery, Fire temperature, Vegetation type, Fire fractional cover, Earth surface albedo, Short-wave Earth surface bi-directional reflectance, Leaf Area Index (LAI), Land cover, Land surface temperature, Sea-ice cover, Snow cover, Normalized Differential Vegetation Index (NDVI), Iceberg fractional cover, Fraction of Absorbed PAR (FAPAR), Glacier motion, Glacier cover, Sea-ice surface temperature, Above Ground Biomass (AGB), Active Fire Detection, Long-wave Earth surface emissivity, Permafrost, Evapotranspiration, Cloud mask, Surface Water Extent, Mineral Type |
Instruments | ETM+ |
Instrument type | Imaging multi-spectral radiometers (vis/IR) |
CEOS EO Handbook | See Landsat-7 summary |
Related Resources
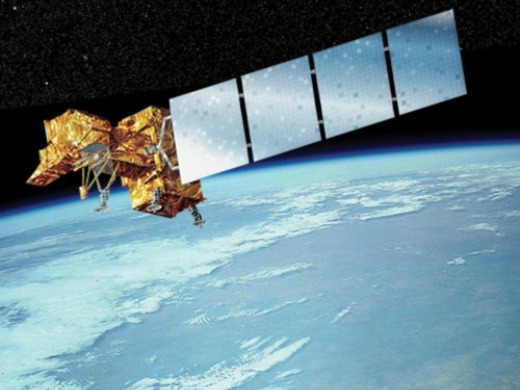
Summary
Mission Capabilities
Landsat-7 features the Enhanced Thermal Mapper Plus (ETM+) developed by Raytheon SBRS (Santa Barbara Remote Sensing) which builds upon the Thermal Mapper (TM) onboard Landsat-4 & -5. ETM+ is a whiskbroom scanning radiometer capable of imaging in eight distinct bands including four visible and near-infrared (VNIR), two short wave infrared (SWIR), one thermal infrared (TIR) and one panchromatic (PAN) band. The addition of the PAN band, improved resolution and two 8-bit “gain” ranges in ETM+ have led to Landsat data progressing into use for global scale studies. Data acquired using ETM+ has applications in land cover/change monitoring, agricultural forecasting, disaster response, urban planning, land and water resource management, and ecosystem monitoring.
Performance Specifications
ETM+ images with a spatial resolution of 15 m for the PAN band, 30 m for VNIR/SWIR bands and 60 m for the TIR band, each covering a swath of 185 km. The scanner contains two focal planes and detector line arrays oriented in the long-track direction. This arrangement provides parallel coverage of 480 m along-track in one scan sweep (cross-track direction). The wide along-track coverage permits sufficient integration time for all cells in each scan sweep.
Landsat-7 undergoes a sun-synchronous polar orbit at an altitude of 705 km with an inclination of 98.2°. The nominal descending equator crossing time is at 1000-1015, with a period of 99 minutes and repeat coverage of 16 days.
Space and Hardware Components
Built by LMMS (Lockheed Martin Missiles and Space), the Landsat-7 spacecraft uses a very similar design to its predecessor, Landsat-6. With a mass of 2200 kg, the satellite features the three-axis stabilised Landsat-6 bus with an onboard recorder in solid state memory. Attitude control is provided by four reaction wheels and two torque rods, sensed with a static Earth sensor, two magnetometers and gyroscopes. The satellite is powered by a four-panel silicon cell solar array and two nickel hydrogen batteries. Orbit control and backup momentum is provided through a blow-down monopropellant hydrazine system with a single tank containing 122 kg of hydrazine.
In May 2003 the Scan Line Corrector (SLC) on ETM+ failed. The function of the SLC was to compensate for the forward motion of the satellite during data acquisition, thus as a consequence of its failure, individual image scans overlap and leave large physical gaps near the edge of each picture. Overall, approximately 30% of the total image is missing in each downlinked picture.
Landsat-7
Spacecraft Launch Mission Status Ground Segment References
The Landsat-7 satellite is part of NASA's ESE (Earth Science Enterprise) program, a joint venture of NASA and USGS (United States Geological Survey). The overall mission objective is to extend and improve upon the long-term record of medium-resolution multispectral imagery of the Earth's continental surfaces provided by the earlier Landsat satellites. 1)
Following the loss of Landsat-6 during launch in 1993, Landsat-7 was placed on a fast track for launch in 1998, but was ultimately launched on 15 April 1999 (a one-year delay resulted from having to replace some faulty electronics inside the ETM+ sensor).
Spacecraft
The S/C and payload were developed under NASA/GSFC management/procurement responsibility (Landsat Project Scientist: D. Williams). The LS-7 satellite was built by LMMS (Lockheed Martin Missiles and Space) at the facility in Valley Forge, PA. The S/C features the Landsat-6 bus; an onboard recorder in solid state memory (378 Gbit capacity to capture data beyond the range of ground receiving stations, recording rates of 150 Mbit/s, playback with 300 Mbit/s), and a single observation instrument: ETM+. 2) 3)
The Landsat-7 spacecraft is very similar in design to the Landsat-6 satellite. It features three-axis stabilization with a pointing capability of 180 arcsec (3σ) and a pointing knowledge of 45 arcsec (1σ). Attitude control is provided with four reaction wheels and two torque rods, attitude is sensed with a static Earth sensor, 2 magnetometers and gyros. Orbit control and backup momentum unloading is provided through a blow-down monopropellant hydrazine system with a single tank containing 122 kg of hydrazine.

S/C mass = 2200 kg, dimensions: 4.3 m in length and 2.8 m in diameter, power = 1550 W [about 1000 W average, provided by a silicon cell solar array (4 panels each of size 1.88 m x 2.26 m) and two nickel hydrogen batteries each of 50 Ahr capacity]. S/C design life = 7 years.

The onboard processor performs autonomously executed functions for wideband communications, electrical power management, and satellite control. These include attitude control, redundancy management, antenna steering, battery management, solar array pointing maintenance, thermal profile maintenance, and stored command execution.
RF communications: All data communication is CCSDS compliant. An onboard SSR (Solid State Recorder) is used to capture wideband data from the ETM+ (storage capacity of 378 Gbit, equivalent for about 100 scenes or 42 minutes of instrument data).
• S-band (2 omni-directional antennas), 5 W, for TT&C data with real-time telemetry data rates of 1.2 kbit/s and 4.8 kbit/s, and 256 kbit/s of playback data, 2 kbit/s of command data. S-band frequencies of 2106.4 MHz (uplink) and 2287.5 MHz (downlink). The zenith antenna is used for TDRS (Tracking Data and Relay Satellite) communications; the nadir antenna is used for Landsat Ground Network (LGN) communications. Each antenna provides essentially hemispherical coverage.
• X-band (3 steerable antennas), 3.5 W; each antenna transmits data on two channels, with each channel carrying 75 Mbit/s (total of 150 Mbit/s per antenna); up to three separate links are supported. X-band frequencies: 8082.5 MHz, 8212.5 MHz, 8342.5 MHz. The downlink beam width of each antenna is 1.2º.
Ground sites exist at Sioux Falls, South Dakota (Landsat Ground Station, or LGS), Poker Flat, Alaska (Alaska Ground Station, or AGS), Wallops Virginia (WPS), and Svalbard, Norway (SGS). All ground sites are equipped with 11 meter antennas. AGS, SGS, and LGS are capable of receiving both S-band (TT&C) at a downlink rate of 4 kbit/s and X-band (payload) data simultaneously.
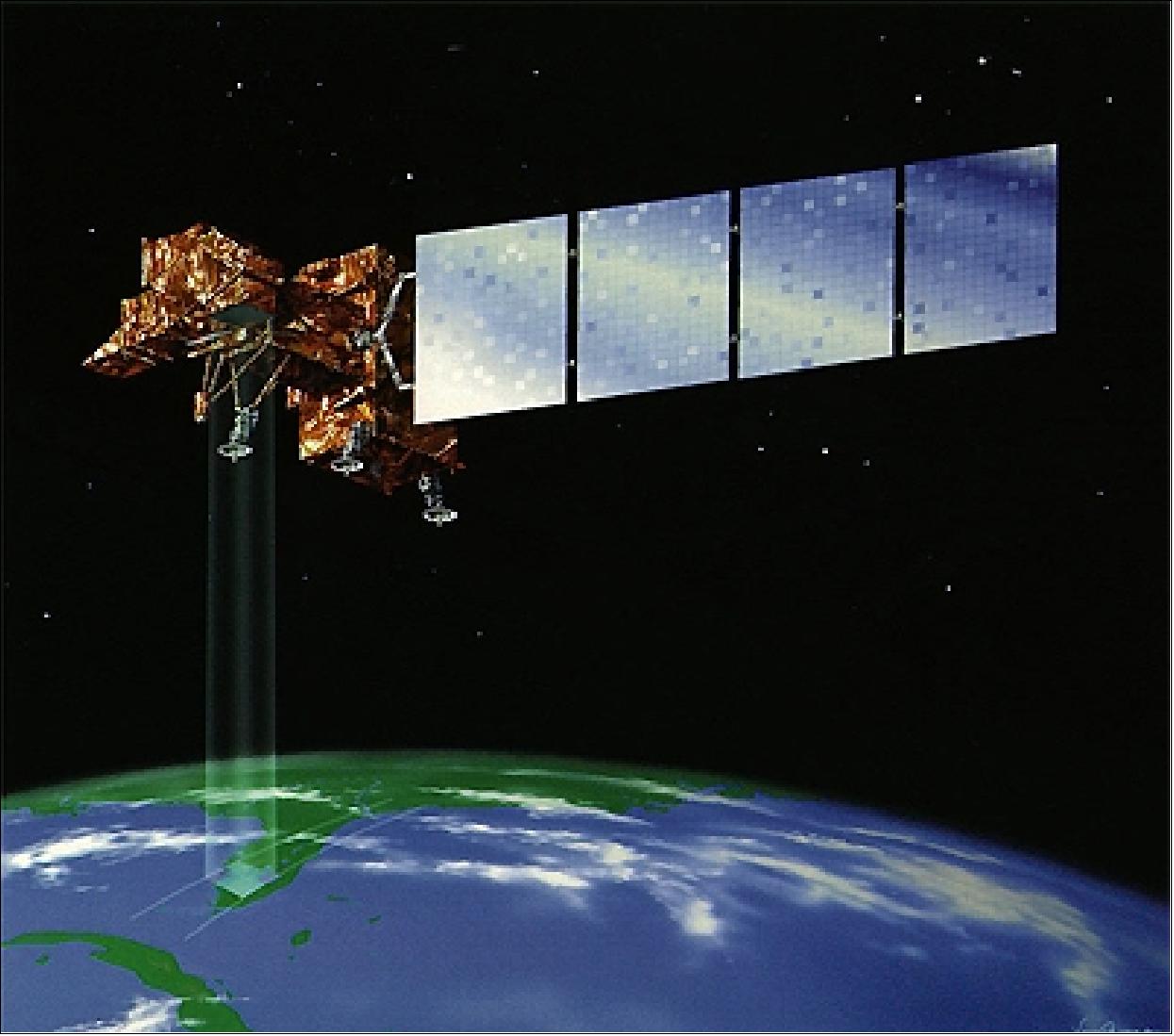
Launch
Launch: A launch of Landsat-7 took place on a Delta 2 vehicle from VAFB, CA on April 15, 1999.
Orbit: Sun-synchronous polar orbit (AM orbit), altitude = 705 km, inclination = 98.2º, period = 99 minutes, repeat coverage = 16 days, the nominal descending equator crossing time is at 10:00 to 10:15 hours.
The ground track is referenced to WRS (Worldwide Reference System) with a repeat accuracy of ±5 km. The WRS indexes orbits (paths) and scene centers (rows) into a global grid system (daytime and night time) comprising 233 paths by 248 rows. 4)
As of early 2001 Landsat-7 is flying in the so-called “morning constellation,” also referred to as morning train with EO-1 (a few minutes apart), SAC-C and Terra. The objective is to compare coincident imagery from the ETM+ and ALI instruments. The “paired scene” images are used to evaluate the performance of ALI. In fact, the EO-1 and SAC-C spacecraft joined the constellation already on Nov. 21, 2000. The overall objective is to obtain synergistic effects for data interpretation and analysis.

Sensor Complement
ETM+ (Enhanced Thematic Mapper Plus)
ETM+ was built by Raytheon SBRS (Santa Barbara Remote Sensing), Goleta, CA. ETM+ is an 8-band whiskbroom scanning radiometer consisting of:
• A primary mirror that sweeps side-to-side (cross-track) to produce forward and revers image scans, and
• A scan line corrector (SLC) mirror assembly that sweeps forward-to-aft to compensate for the forward motion of the spacecraft during integration time. The motion of these mirrors deviates from an ideal line profile, introducing along- and cross-track geometric distortions that require compensation.
The principal functional differences between the ETM and the former TM series are the addition of a 15 m resolution panchromatic band and two 8-bit “gain” ranges. The ETM+ adds a 60 m resolution thermal band, replacing the 120 m band on ETM/TM (band No. 6). Design life = 7 years. 5)
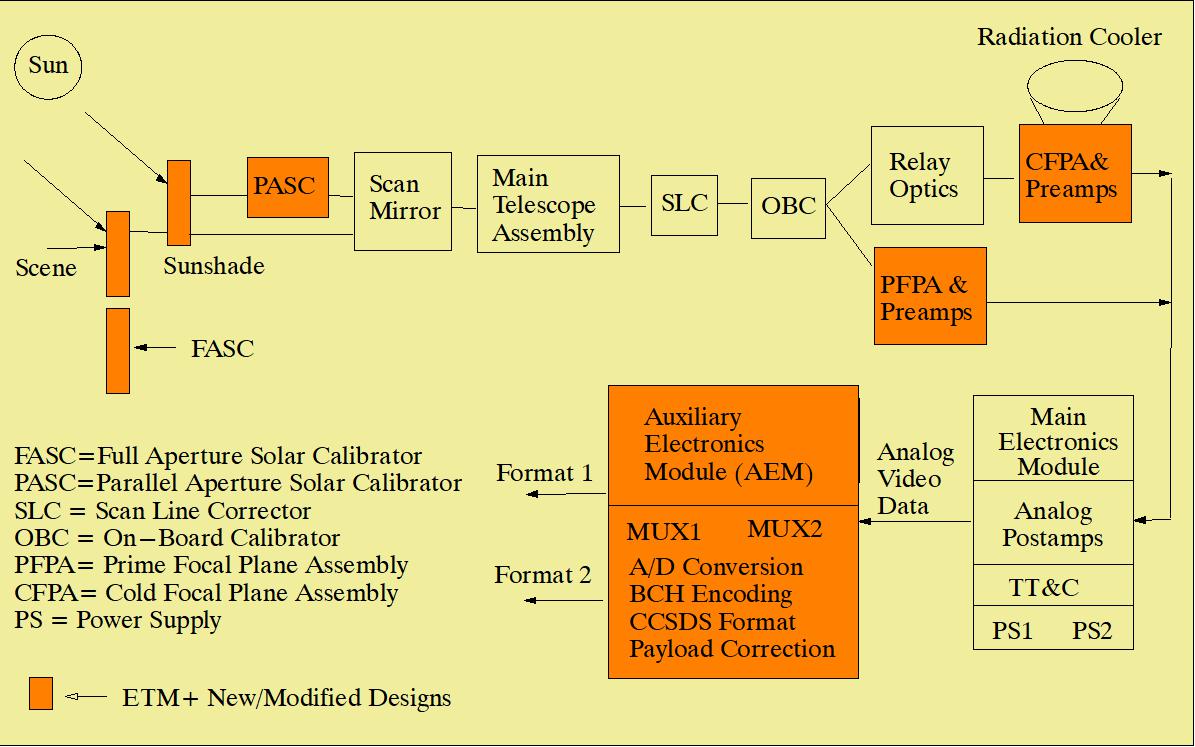
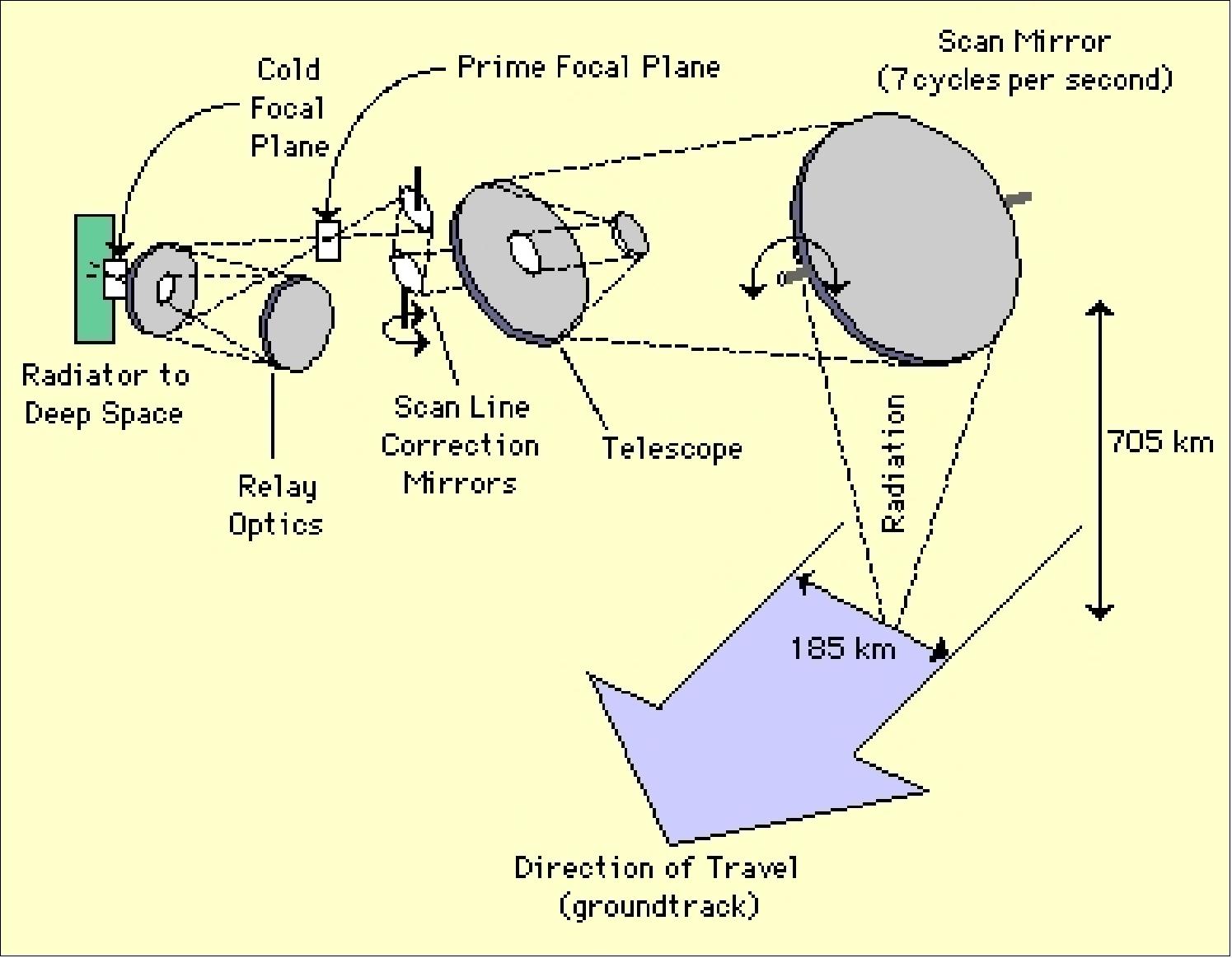
The Scan Mirror Assembly (SMA) provides the cross-track scanning motion to develop the 185 km long scene swath. The SMA consists of a flat mirror supported by flex pivots on each side (which have compensators to equalize pivot reaction torque), a torquer, a scan angle monitor (SAM), 2 leaf spring bumpers and scan mirror electronics (SME). The bi-directional SMA sweeps the detector's line of sight in west-to-east and east-to-west directions in cross-track direction, while the spacecraft's orbital path provides the north-south motion.
The ETM+ scanner contains 2 focal planes that collect, filter, and detect the scene radiation in a swath, 185 km wide. The primary focal plane consists of optical filters, detectors, and preamplifiers for 5 of the 8 ETM+ spectral bands (bands 1-4, 8). The second focal plane is the cold focal plane which includes the optical filters, infrared detectors, and input stages for ETM+ spectral bands 5,6, and 7. The temperature of the cold focal plane is maintained at 91 K using a radiative cooler. The detector line arrays (16 for VNIR bands, 32 for PAN, and 8 detectors for TIR) of the whiskbroom scanner are oriented in the along-track direction. This arrangement provides a parallel coverage of 480 m along-track in one scan sweep (cross-track direction). The wide along-track coverage permits sufficient integration time for all cells in each scan sweep.
Band No. | Wavelength (µm) | Detectors | IFOV (µrad) | GSD (m) | SNR (at min signal radiance) |
8 PAN | 0.52 - 0.90 | SiPD (32) | 18.5 x 21.3 | 13 x 15 | 15 |
1 VIS | 0.45 - 0.52 | SiPD (16) | 42.5 | 30 x 30 | 32 |
2 VIS | 0.53 - 0.61 | SiPD (16) | 42.5 | 30 | 35 |
3 VNIR | 0.63 - 0.69 | SiPD (16) | 42.5 | 30 | 26 |
4 VNIR | 0.78 - 0.90 | SiPD (16) | 42.5 | 30 | 32 |
5 SWIR | 1.55 - 1.75 | InSb (16) | 42.5 | 30 | 25 |
7 SWIR | 2.09 - 2.35 | InSb (16) | 42.5 | 30 | 17 |
6 TIR | 10.4 - 12.5 | HgCdTe (8) | 85.2 | 60 | 0.5 K |
The ETM+ also includes a number of radiometric enhancements to achieve an absolute radiometric uncertainty of <5% (bands 1-4). Two new calibration devices were added: FAC (Full Aperture Calibrator), and PAC (Partial Aperture Calibrator). ETM+ uses three independent onboard calibration systems (plus preflight calibration), representing a significant step forward in absolute radiometric calibration accuracy. 6) 7)
• A full-aperture solar diffuser (FASC) on the inner surface of the aperture door that illuminates the focal planes with diffusely reflected solar energy when commanded into position
• A partial-aperture solar reflector (PASC) that illuminates the focal planes with attenuated solar energy, once per orbit
• Internal calibrator (IC). Calibration lamps that project calibrated energy onto the focal planes via the main calibration shutter, once per scan, during the scan mirror turnaround.
From the initiation of the LS-7 system, greater attention was paid toward the long-term characterization and calibration of the data than for earlier LS missions. In particular, an IAS (Image Assessment System) was incorporated into the ground processing system. The objective of IAS is to characterize and calibrate the instrument data over the life of the mission. 8) 9) 10) 11) 12)
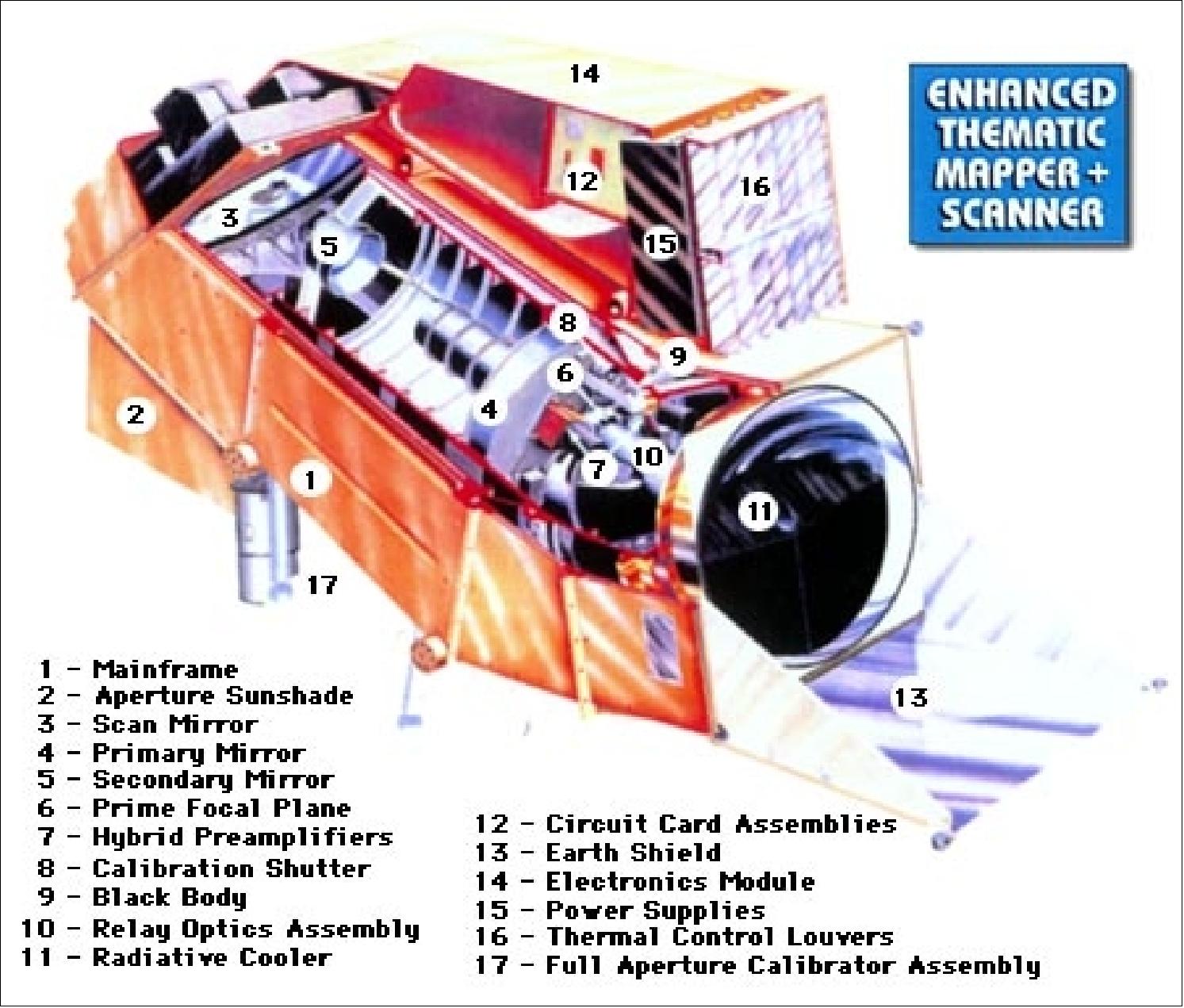
Scanning method | Bidirectional cross-track, scan frequency = 7 Hz |
Scan period | 142.9 ms, (scan frequency of 6.99 Hz) |
Swath width | 185 km (15º FOV from 705 km orbit) |
Telescope | 40.6 cm aperture diameter, Ritchey-Chretien configuration with a primary and secondary mirror and baffles; mirror material: ULE (Ultra Low Expansion) glass |
Effective focal length | 243.8 cm, (f/6.0) |
Instrument size | Scanner assembly: 1.5 m x 0.7 m x 2.5 m |
Instrument mass | Scanner assembly: 298 kg, AEM = 103 kg, cable harness = 20 kg |
Power | 590 W |
Data quantization | 9 bit A/D conversion, 8 bit/pixel transmitted (2 gain states) |
Data rate | 150 Mbit/s (2 x 75) by each of three directional X-band antennas, CCSDS format |
Cross calibration was performed between ETM+ and ALI [(Advanced Land Imager) on the EO-1 (Earth Observing-1) mission] image pairs using two approaches. One approach was based on image statistics of large common areas between the image pairs. The other approach was based on vicarious calibration that compares the measured radiance obtained from the sensor to the predicted at-sensor radiance using the surface measurements propagated to the sensor via radiative transfer code. 13) 14) 15)
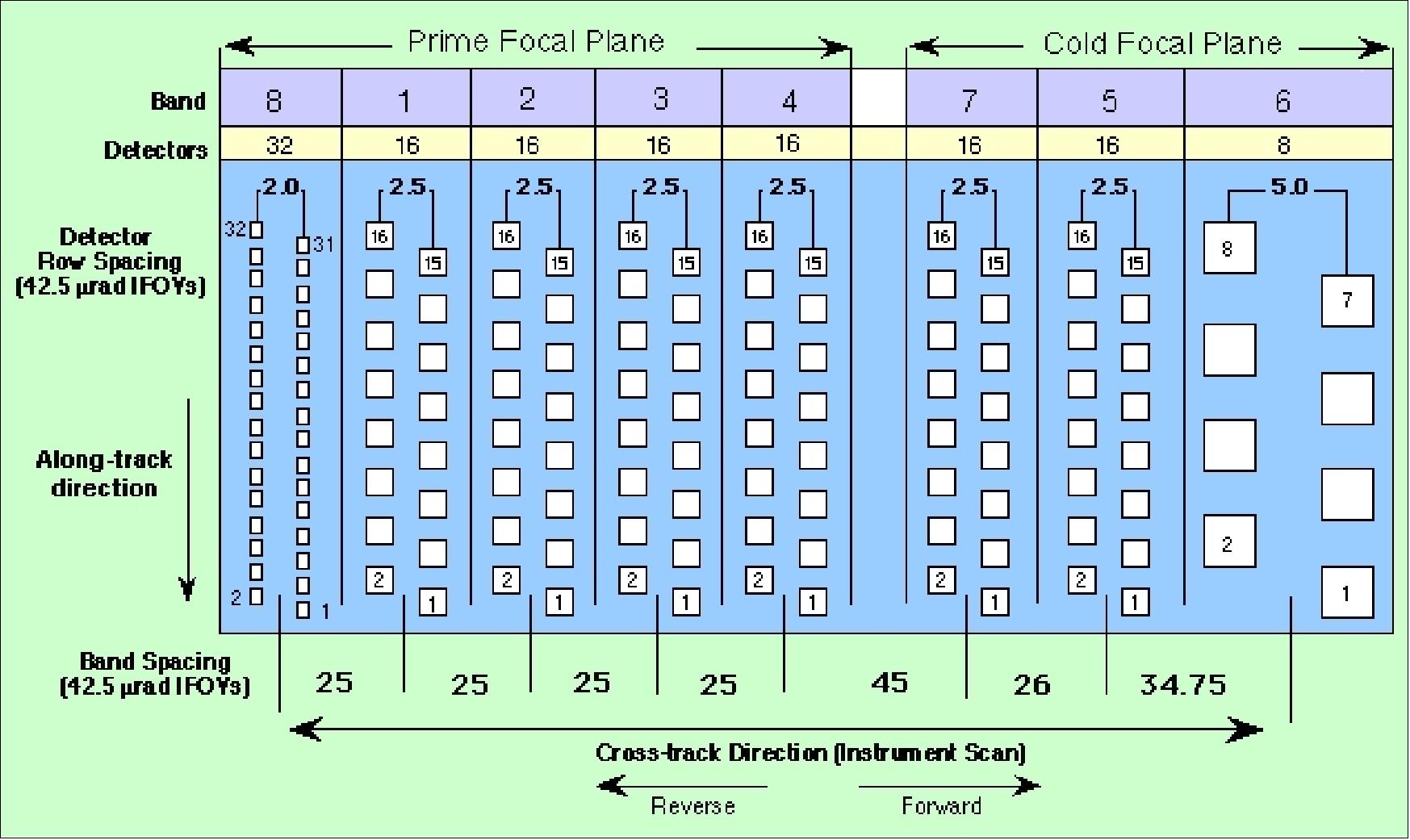
Landsat sensor | MSS | TM | ETM | ETM+ |
Spectral bands (all bands in µm) | 1) 0.5 - 0.6 | 1) 0.45 - 0.52 VNIR | P) 0.52 - 0.90 VNIR | P) 0.52 - 0.90 VNIR |
Swath width | 185 km | 185 km | 185 km | 185 km |
Spatial | 80 m | 30 m VNIR/SWIR | 15 m PAN, 30 m VNIR/SWIR, 120 TIR | 15 m PAN |
Radiometric resolution | 6 bit | 8 bit | 9 bit (8 bit transmitted) | 9 bit (8 bit transmitted) |
Band-to-band registration |
| 0.2 pixel (90%) | 0.2 pixel (90%) | 0.2 pixel (90%) |
Geodetic accuracy without ground control |
| 500 m (90%) | 1000 m (90%) | 400 m (90%) |
Data rate | 15 Mbit/s | 85 Mbit/s | 2 x 85 Mbit/s | 2 x 75 Mbit/s |
Instrument mass | 64 kg | 258 kg | 288 kg scanner, plus 81 kg AEM | 318 kg scanner, plus |
Average power | 50 W | 332 W | 490 W | 590 W |
Telescope aperture | 23 cm | 40.6 cm | 40.6 cm | 40.6 cm |
Sensor Status
The SLC (Scan Line Corrector) on the ETM+ instrument failed on May 31, 2003. The SLC function is to compensate for the forward motion of the satellite during data acquisition. As a consequence of this operational anomaly, individual image scans overlap and also leave large physical gaps near the edge of each picture. Only portions of the image near the center are left completely unfettered and valid. Overall, about 30 percent of the total image is missing in each downlinked picture.
Spacecraft controllers immediately suspended normal LS-7 operations and limited activity to just spacecraft housekeeping and operations related to the anomaly investigation and recovery effort. However, subsequent efforts to recover the SLC were not successful and the problem appears to be permanent. Without an operating SLC, the ETM+ line of sight now traces a zig-zag pattern along the satellite ground track (Figure 10). The resulting gaps in coverage range from none at the center of the scan to 14 pixels at the extreme edges of the scan.
As of Sept. 16, 2003, LS-7 has resumed its normal Long Term Acquisition Plan (LTAP) scheduling of approximately 250 scenes per day, and all data will now be acquired in SLC-off mode. ETM+ is still capable of acquiring useful image data with the SLC turned off, particularly within the central portion of any given scene.
As of Oct. 2003, the USGS/NASA Landsat team is trying to develop means to compensate for the SLC malfunction, with image processing methods and acquisition strategies to exploit the remaining observation capability of the LS-7 system. The team is refining gap-filling techniques that merge data from multiple acquisitions. They are also developing modifications to the LS-7 acquisition scheme to acquire two or more clear scenes as near in time as possible to facilitate this gap-filling process. These merged images potentially resolve most, if not all, of the missing data problems.

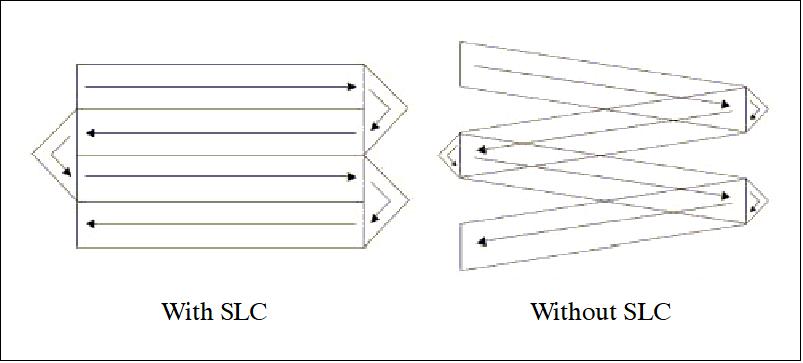
The USGS reinitiated routine collection of ETM+ data with the SLC turned off on July 14, 2003 and began distributing this data in late October 2003. Initially, the USGS offered data products with a fixed maximum interpolation of two pixels in their fully processed data products. Beginning in mid-Feb. 2004, the maximum amount of interpolation became user selectable. Starting in May 2004, USGS began providing the first in a series of data products to help make the SLC-off data more usable. 16) 17) 18)
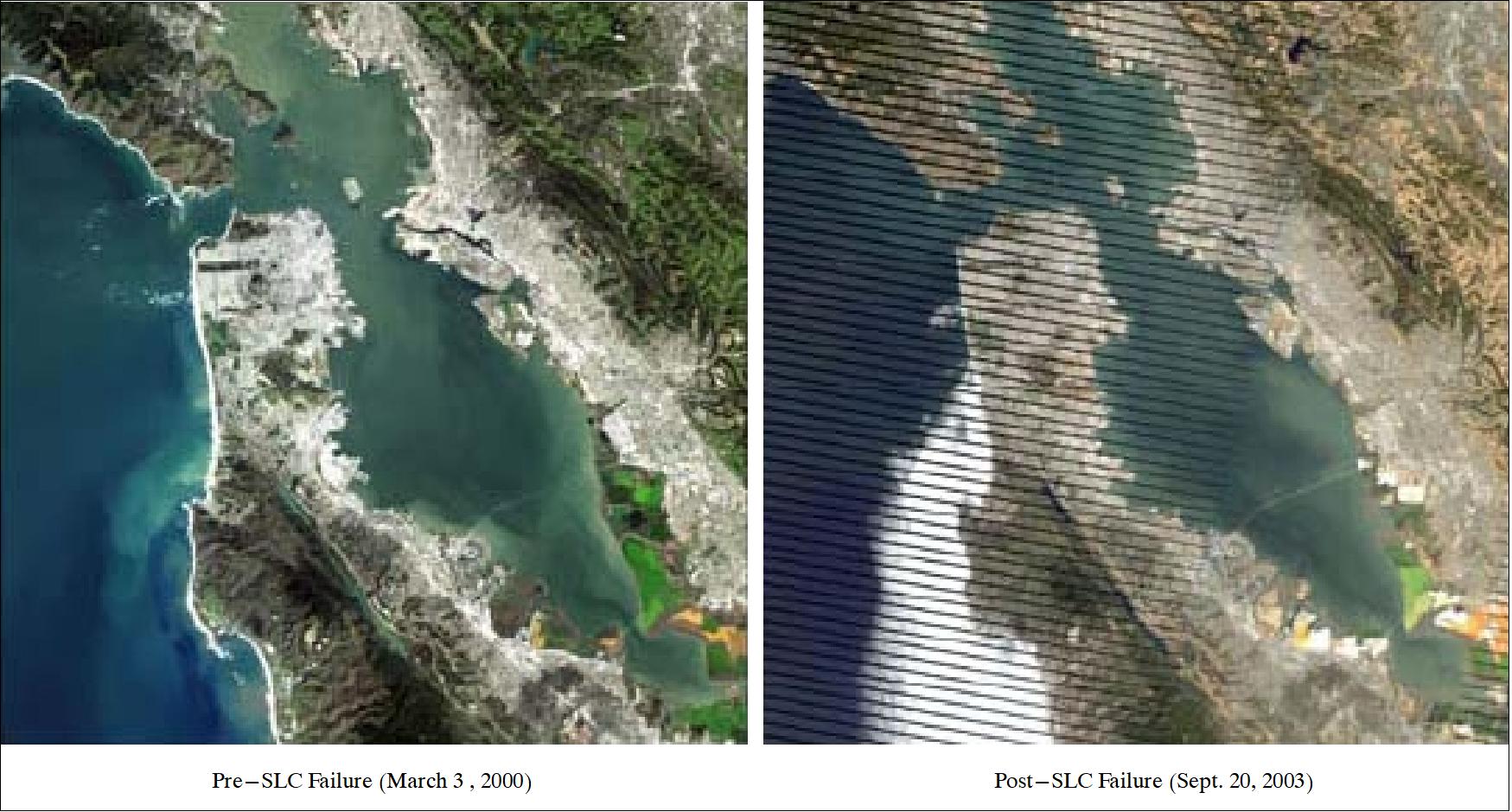

Legend to Figure 12: The Lena river of 4,400 km in length, is one of the largest rivers in the world. The Lena Delta Reserve is the most extensive protected wilderness area in Russia. It is an important refuge and breeding grounds for many species of Siberian wildlife. 20)
Mission Status
• May 5, 2022: Landsat-7 operations continued in an experimental capacity. Imaging resumed at a lower orbit of 697 km. Data from the L7 extended science mission was made publically available by August 2022. 68)
• April 6, 2022: Nominal science mission operations ended. In order to reduce the satellite's orbit by eight kilometres throughout the month of April, a series of spacecraft burns were carried out, putting the ETM+ sensor in "stand-by" mode. 69)
• January 26, 2021: Landsat 7 was used to analyse movement of the Sverdrup Glacier, in north Greenland between 1998 and 2007. Sverdrup represents a large class of Greenland glaciers that are undergoing rapid retreat in response to warm ocean water. 21)

- The position in 2000 was similar to the mid-1980s, indicating that there had been a period of stability when ocean temperatures were cool. Then, between 1998 and 2007, waters around Greenland warmed rapidly—almost 2 degrees Celsius—and the glacier started to thin, flow faster, and retreat. To assess how warming ocean water affects coastal glaciers, scientists with the Oceans Melting Greenland (OMG) mission have been studying these marine-terminating glaciers from the air and by ship. In a recent study, scientists used these data to show that when it comes to glacier melting, the depth of the fjord matters. Glaciers in deep fjords come into contact with more warm ocean water than glaciers in shallow fjords. In contrast, the 51 glaciers that extend into shallow fjords or onto shallow ridges experienced the least undercutting and contributed only 15 percent of the total ice loss. These findings suggest that climate models may underestimate glacial ice loss by at least a factor of two if they don’t account for undercutting by a warm ocean. The study also lends insight into why many of Greenland’s glaciers never recovered after the abrupt warming of ocean water between 1998 and 2007.
• December 7, 2020: Trees play a vital role in Earth’s carbon and water cycles. They also contribute food, firewood, and other resources important to human activity. But while forests are easy to spot from above, smaller stands of trees have often gone undercounted because they have been harder to detect with the satellite imagers usually available to scientists. 22) Now an international team of scientists has used artificial intelligence and commercial satellites to identify an unexpectedly large number of trees spread across arid and semi-arid areas of western Africa. These drylands were previously classified as having little to no tree cover, but new analysis techniques proved otherwise. The findings could help scientists better understand and quantify the role drylands have in the storage and cycling of carbon. 23) The research team used the Blue Waters supercomputer at the University of Illinois and an artificial intelligence technique called “deep learning” to map the trees. Once the dataset was tested, Blue Waters—one of the fastest supercomputers in the world—took just a few days to identify nearly 2 billion trees across 1.3 million km2 (500,000 square miles) of sub-Saharan Africa. Previous land cover mapping studies of the region relied on satellite imagery with 10– or 30–meter resolution (the distance visible within each pixel). Landsat-7 data contributed to the research.
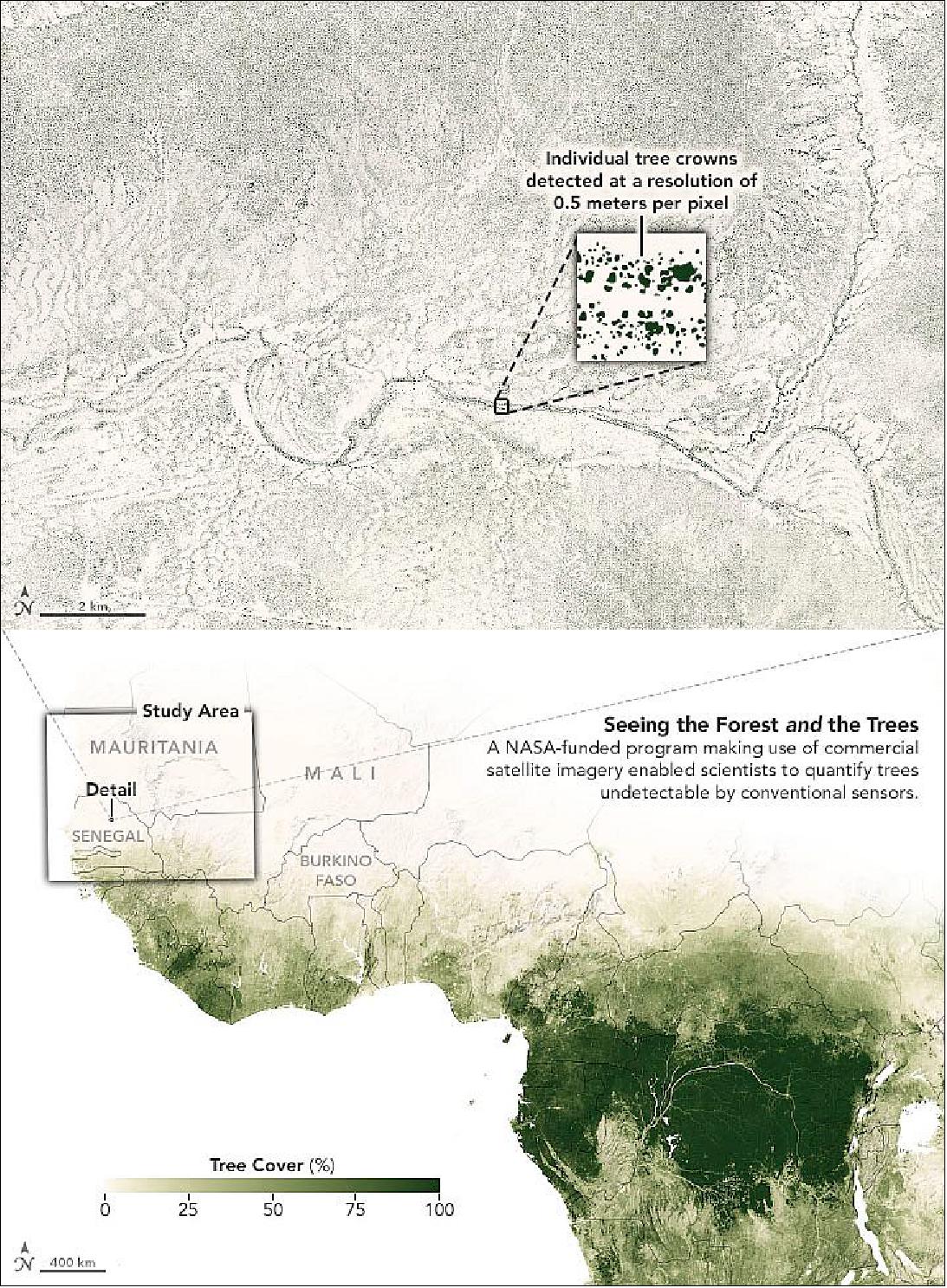
- This machine-learning method identified 1.8 billion individual trees standing outside of areas classified as forests. It also measured the crown diameter of each tree. Tree cover was, predictably, higher in areas with more rainfall. The published dataset—which includes the location and crown diameter of trees in western Africa—through the Oak Ridge National Laboratory Distributed Active Archive Center (ORNL DAAC). Users can also find it through a NASA Earthdata search.
• July 2, 2019: The border between land and sea is not a fixed line because of the Moon, Sun, and gravity. What we see on a map is just a representation of where the mean sea level is found, and the coast of any landmass is a moving target between low and high tides. This ever-shifting region is known to scientists as the intertidal zone. 29)
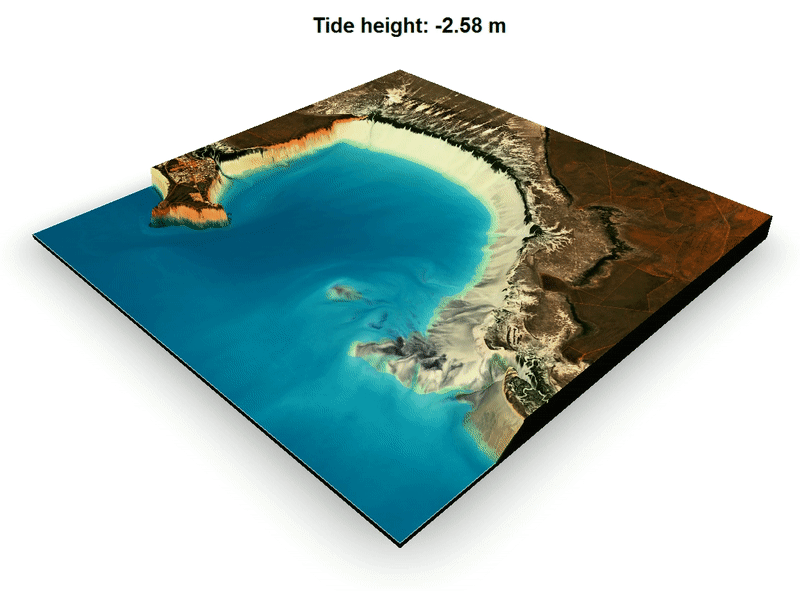
- The world’s intertidal zones include an array of ecosystems: sandy beaches, rocky shores, tidal pools, mudflats, seagrass beds, mangrove forests, and fringing coral reefs. For instance, the intertidal zone is an indispensable feeding ground for shorebirds, particularly during migration. For humans, the zone provides recreational spaces and the first line of defense against the sea. Knowing the width and height of the intertidal zone aids in tsunami preparedness, storm surge risk management, current modeling, and ecological habitat mapping. Yet this region has largely been missed in most mapping efforts, falling between well-mapped dry land and bathymetry (depth) measurements of the ocean. The team created the first automated, open-source method for deriving intertidal zone elevation. The work was published in the journal Estuarine, Coast and Shelf Science.
- Building on earlier work that used Landsat to map the horizontal extent—the x and y directions—of the intertidal zone, the Geoscience Australia team applied knowledge of the tidal range and of the presence or absence of seawater during the tidal cycle to model the elevation relative to mean sea level. The accuracy of this approach for tidal flats and sandy shores is high, approaching that of lidar-derived elevations. For rocky shores and places where the tidal model does not capture the complex or extreme tidal patterns, NIDEM is a bit less accurate. For instance, researchers at James Cook University have already incorporated the full NIDEM data set into the elevation model for the Great Barrier Reef and Queensland coast.
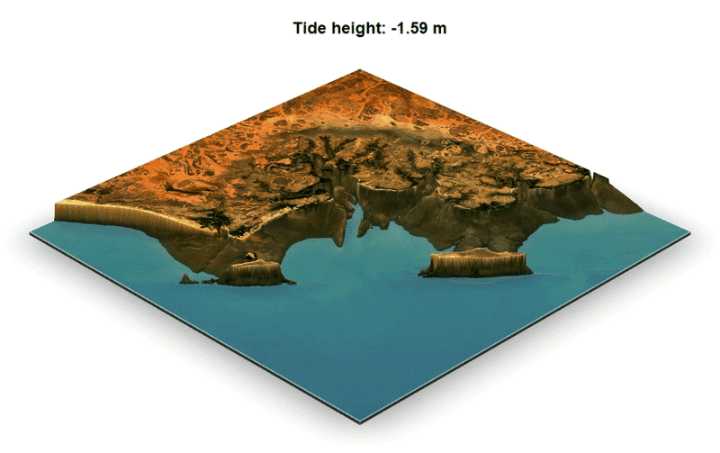
• June 4, 2019: Mongolia’s capital, Ulaanbaatar, sits near the confluence of the Selbe and Tuul rivers on a plateau at the foot of a large, forested mountain. This was not always the case. When the town was initially established in the 1600s, it was a migratory settlement built as a center for Buddhist priests. It changed locations more than two dozen times. 30) Ulaanbaatar settled into a permanent location in the 1700s, but it remains a place of rapid change. These days, the growth is mostly outward into low-density neighborhoods that have emerged on the fringes of the city, particularly in river valleys and hillsides north of the city center. Migrants typically set up gers, the circular, herder tents that nomadic people have traditionally used in the Mongolian steppe. In contrast, the core of the city features Soviet style architecture—grand neoclassical government buildings and grids of rectangular, multi-story apartments that were mostly built in the 1960s. Several teams of scientists have used Landsat images to monitor how the city—particularly the ger districts—have changed over several decades. According to some estimates, ger districts now hold more than 60 percent of Ulaanbaatar’s population.

• February 21, 2019: KBR, Inc. (formerly Kellogg Brown & Root) of Houston, TX, announced today that its global government services business, KBRwyle, has been awarded a $19 million contract by the U.S. Geological Survey (USGS) to support flight operations for its Landsat-7 satellite. 31) The Landsat-7 satellite is part of the Landsat program, a joint initiative of the USGS and NASA. Landsat satellites provide space-based images of the Earth's land surface, collecting valuable data that helps reduce hunger on Earth, enhances how quickly emergency response teams stabilize fires, and aids scientists in understanding humanity's impact on the planet.
- Under this contract, KBRwyle will perform flight operations, system maintenance, testing, and sustainment engineering activities to support the on-orbit Landsat 7 spacecraft and payload. KBRwyle will also operate and maintain the Mission Operations Center (MOC) ground systems and backup Mission Operations Center (bMOC). Additionally, the company will continue to participate in a NASA/USGS initiative to robotically refuel the Landsat 7 spacecraft.
- KBRwyle primarily performed this work at NASA's Goddard Space Flight Center in Greenbelt, Maryland, as well as at its office in Columbia, Maryland. KBRwyle provides technical support services to the USGS EROS Center. At this center, KBRwyle maintains and operates the Landsat-7 and -8 ground processing systems while helping to develop the next generation Landsat-9 ground system in support of its planned launch. The EROS Center processes and distributes data from the Landsat archive and other Earth observing satellites, as well as other forms of geographic science products and information.
• April 27, 2018: The Kerguelen Islands rise from the southern Indian Ocean, not far from the “Furious Fifties.” As a result, the Kerguelens are often shrouded by clouds, making it hard for satellites to get a good look at the shrinking ice cap on the archipelago’s main island, Grand Terre. 33) Lamarack Glacier retreated by 1100 meters, its terminus also trading water for land, while Descartes Glacier retreated by 1000 meters through a narrow arm of a lake. Notice the appearance of nunataks—peaks of rock that now protrude from the ice surface. A study published in 2015 confirmed that the retreat of this ice cap is due primarily to changes in snowfall.

• April 15, 2018: Landsat-7 had spent 19 years in orbit.
• March 22, 2018: In a part of the Amazon rainforest that reaches into northeastern Peru, there are no roads cutting through the vegetation and no visible patches of deforestation. “When you see it from the air, it appears to stretch to the horizon,” said Corine Vriesendorp, a conservation ecologist at the Field Museum. 34) Formally established in January 2018, Yaguas National Park helped ensure that more than 2 million acres (809,370 ha) of Peruvian Amazon forest stay intact. The park’s boundaries are shown here, atop a map of aboveground forest biomass. Satellite data and models were used to estimate the density of woody material (Figure 22); dark green areas are denser than light green areas. These intact forests are important for supporting biodiversity. They give plants and animals adequate space to maintain healthy populations and to continue to evolve as they have over millions of years. Uninterrupted or “connected” forests can even help species respond to climate change by allowing them to migrate to areas with more moisture or higher, cooler elevations.
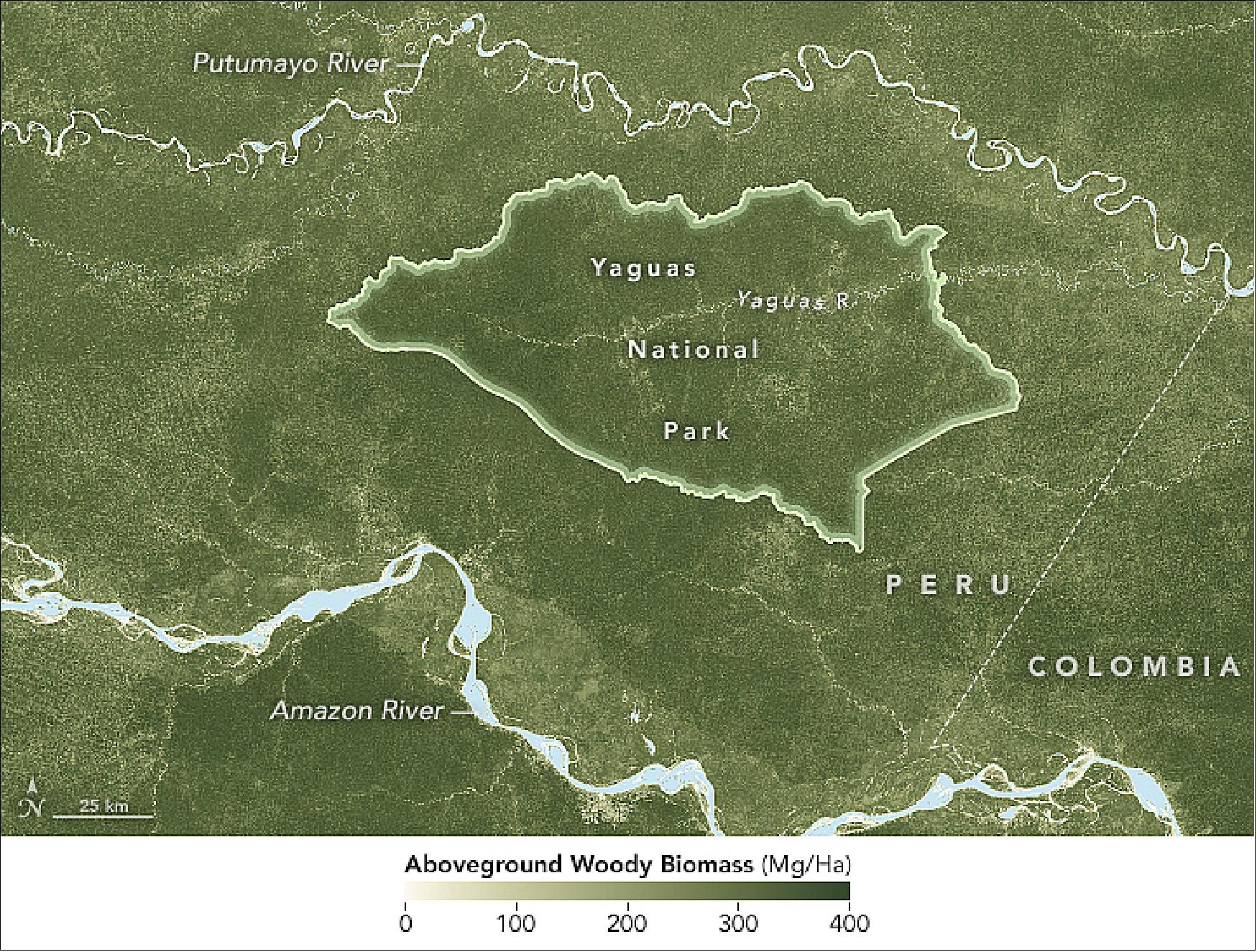
• March 21, 2018: Many of Senegal’s largest rivers are flanked by corridors of green and interlaced with networks of sinuous creeks and streams. These lush green areas are havens for mangroves—short, shrubby trees with waxy leaves and tangles of finger-like roots that rise from shallow tidal areas. 35) The blend of land, water, and wood that define Senegal’s mangrove forests provides breeding grounds and nurseries for fish, prevents erosion during tropical cyclones, and helps cleanse waters of pollutants. While scientists estimate that Earth lost at least 35 percent of global mangrove habitat between 1980 and 2000 (often due to aquaculture and other coastal development), there are signs that these ecosystems are surviving and even retaking lost ground in some areas. For instance, Senegal’s mangroves have experienced significant regeneration and expansion since 2000, according to a satellite-based analysis by NASA scientists Lola Fatoyinbo and David Lagomasino.
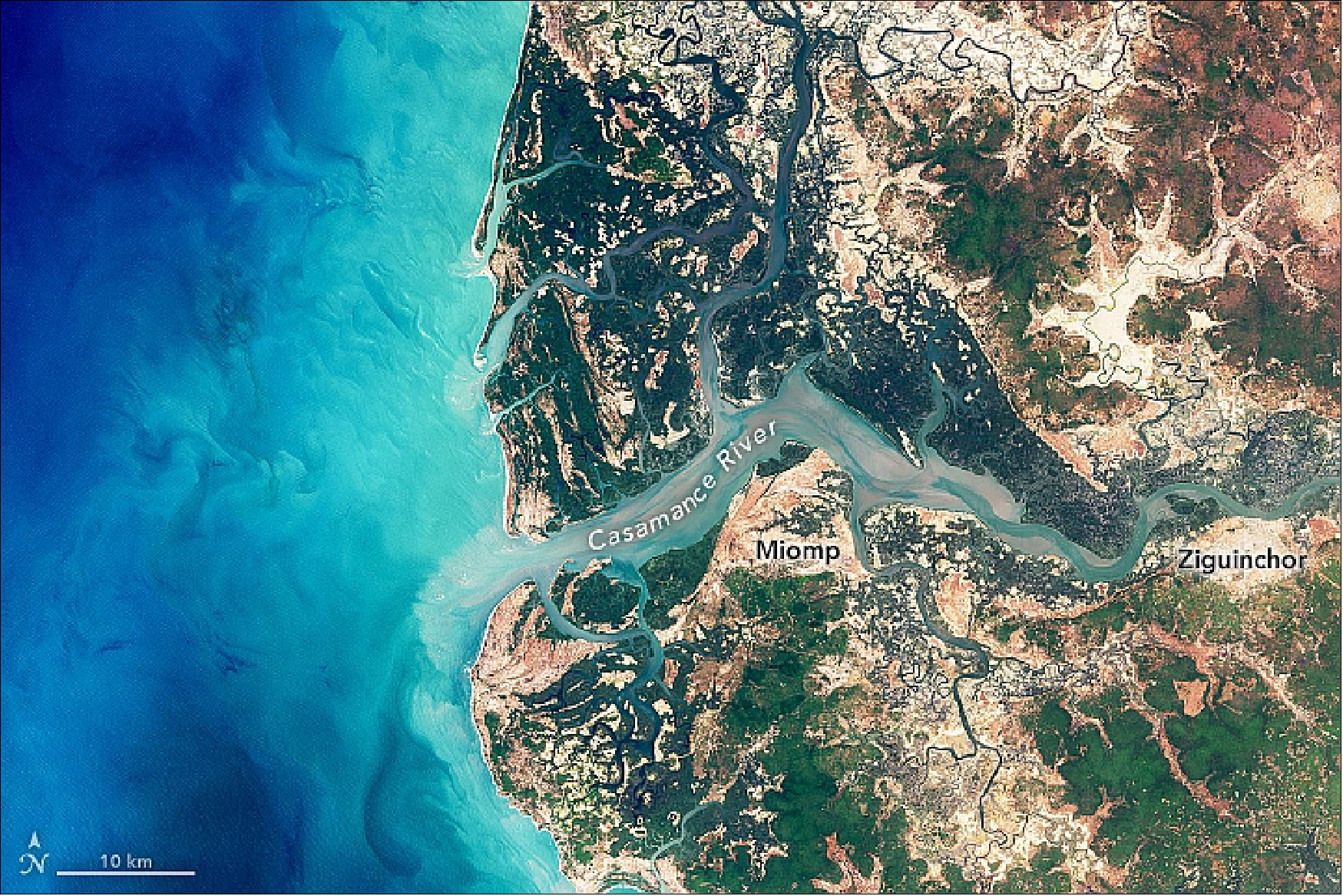
• September 14, 2017: Engineers successfully completed recovery efforts of the Landsat-7 SSR (Solid State Recorder) anomaly that occurred on September 13, 2017. The satellite resumed nominal imaging operation as of September 13th and data are available to download from EarthExplorer, GloVis, or the LandsatLook Viewer. 36)
• May 11, 2017: More than a month after being ignited by lightning, the West Mims fire continues to burn along the Florida-Georgia border. On May 8, 2017, the ETM+ (Enhanced Thematic Mapper Plus) on the Landsat 7 satellite captured this image of the wildfire, most of which has burned within the Okefenokee National Wildlife Refuge. The Okefenokee National Wildlife Refuge has a size 1,627 km2 located in Charlton, Ware, and Clinch Counties of Georgia, and Baker County in Florida, United States. 37) According to InciWeb, the burned area grew from 100,500 acres (401 km2) on May 2 to more than 133,700 acres (541km2) on May 8. A closer view of the burn scar is visible in the first photograph below, acquired from aircraft on April 25, 2017.

• January 10, 2017: Cambodia has one of the fastest rates of forest loss in the world. In broad swaths of the country, densely forested landscapes—even those in protected areas—have been clear-cut over the past decade. Much of the forest has been cleared for rubber plantations and timber. 38) Scientists from the University of Maryland and the World Resources Institute’s Global Forest Watch have been using Landsat satellite data to track the rate of forest loss on a global scale. Though other countries have lost more acres in recent years, Cambodia stands out for how rapidly its forests are being cleared. Between 2001 and 2014, the annual forest loss rate in Cambodia increased by 14.4 %. The transformation of Cambodia’s landscape has been profound, as revealed by this pair of Landsat images. The image of Figure 25, captured by Landsat 7’s ETM+ on December 31, 2000, shows an intact forest near the border of the Kampong Thom and Kampong Cham provinces. Researchers working with Landsat data and other economic datasets have demonstrated that changes in global rubber prices and a surge of land-concession deals have played key roles in accelerating Cambodia’s rate of deforestation. Researchers found that the rate of forest loss within concession lands was anywhere from 29 to 105% higher than in comparable lands outside the concessions.


• November-December 2016: Landsat-7’s duty cycle was raised to 105%, resulting in ~15 more scene acquisitions per day. On average, Landsat-7 started acquiring around 470 scenes per day. The USGS committed to continuity by extending Landsat-7’s operational life until the launch of Landsat-9. Once retired, plans were prepared to use Landsat-7 to test satellite-refueling technology via the NASA Restore-L mission. 39)
• May 2016, NASA officially moved forward with plans to execute the ambitious, technology-rich Restore-L mission, an endeavor to launch a robotic spacecraft in 2020 to refuel a live satellite. The mission – the first of its kind in LEO (Low Earth Orbit), will demonstrate that a carefully curated suite of satellite-servicing technologies is fully operational. The key candidate client for this venture was Landsat-7 40)
• July 2016: Soil salinity is one of the most important problems affecting many areas of the world. Saline soils present in agricultural areas reduce the annual yields of most crops. This research deals with the soil salinity mapping of the Seyhan plate of Adana district in Turkey from the years 2009 to 2010, using remote sensing technology. In the analysis, multitemporal data acquired from Landsat-7 ETM+ satellite in four different dates (19 April 2009, 12 October 2009, 21 March 2010, 31 October 2010) are used. 42) The main objectives of this study are: (i) to understand the spectral reflectance characteristics of saline soil in the Seyhan plate, (ii) to explore the potential of Landsat imagery to detect and map the soil salinity over the study area, (iii) to analyze the correlation between field measurements and Landsat imagery, and (iv) to produce the soil salinity map according to high, moderate and low saline content.
- Çukurova is a district in south central of Turkey covering the provinces of Adana and Mersin. The total area of the Çukurova is about 38,000 km2, Turkey’s biggest delta plain with a large stretch of flat and fertile land, which is among the most agriculturally productive areas of the world. The Landsat-7 ETM+ satellite images with 30 m resolution were used.The most compatible and close dates were selected according to the dates of field work not to have any problems like seasonal changes. The dates of satellite images used are given in the Table 6. As a first step, preprocessing of Landsat images is applied. Several salinity indices such as NDSI (Normalized Difference Salinity Index), BI (Brightness Index) and SI (Salinity Index) are used besides some vegetation indices such as NDVI (Normalized Difference Vegetation Index), RVI (Ratio Vegetation Index), SAVI (Soil Adjusted Vegetation Index) and EVI (Enhanced Vegetation Index) for the soil salinity mapping of the study area.
Date of field measurements | Date of satellite pass |
02-05-2009 | 19-04-2009 |
04-10-2009 | 12-10-2009 |
04-10-2010 | 31-10-2010 |
24-03-2010 | 21-03-2010 |

- Among all different band combinations tested, the correlation of 18 sampled points of March 21, 2010 with EC measurements, showed the highest correlation (78.40%) due to near simultaneous acquisition of the satellite data (3 days). After the correlation analysis, the satellite data showing the highest correlation (March 21, 2010) were chosen to map the soil salinity in the area As observed, the highest saline soils in the study area are taking place in the region covering reeds due to the presence of high amount of salt in the lake. Although the scan line error of Landsat-7 ETM+ can be corrected using the available remote sensing software, the original values are modified.
• November 2015: Landsat-7 continued to aquire geometrically and radiometrically accurate data worldwide, and methods were established that allowed users to fill the data gaps. 43) Landsat data support a vast range of applications in areas such as global change research, agriculture, forestry, geology, land cover mapping, resource management, water, and coastal studies. Specific environmental monitoring activities such as deforestation research, volcanic flow studies, and understanding the effects of natural disasters all benefit from the availability of Landsat data. In recent years, Landsat data have also been used to track oil spills and to monitor mine waste pollution. Table 7 lists Landsat bands and describes the use of each band to help users determine the best bands to use in data analysis.
Band name | LS8 | LS7 (ETM+) | LS4-5 (TM) | LS4-5 (MSS) | LS1-3 (MSS) | Description of use |
Coastal/Aerosol | Band 1 | - | - | - | - | Coastal areas and shallow water observations; aerosol, dust, smoke detection studies |
B (blue) | Band 2 | Band 1 | Band 1 | - | - | Bathymetric mapping; soil/vegetation discrimination, forest type mapping, and identifying manmade features |
G (green) | Band 3 | Band 2 | Band 2 | Band 1 | Band 4 | Peak vegetation; plant vigor assessments |
R (Red) | Band 4 | Band 3 | Band 3 | Band 2 | Band 5 | Vegetation type identification; soils and urban features |
NIR (Near Infrared) | Band 5 | Band 4 | Band 4 | Band 3 | Band 6 | Vegetation detection and analysis; shoreline mapping and biomass content |
SWIR1 (Shortwave Infrared-1) | Band 6 | Band 5 | Band 5 | - | - | Vegetation moisture content/drought analysis; burned and fire-affected areas; detection of active fires |
SWIR2 (Shortwave Infrared-2) | Band 7 | Band 7 | Band 7 | - | - | Additional detection of active fires (especially at night); plant moisture/drought analysis |
PAN (Panchromatic) | Band 8 | Band 8 | - | - | - | Sharpening multispectral imagery to higher resolution |
Cirrus | Band 9 | - | - | - | - | Cirrus cloud detection |
TIR (Thermal Infrared) | Band 10 | Band 6 | Band 6 | - | - | Ground temperature mapping and soil moisture estimations |
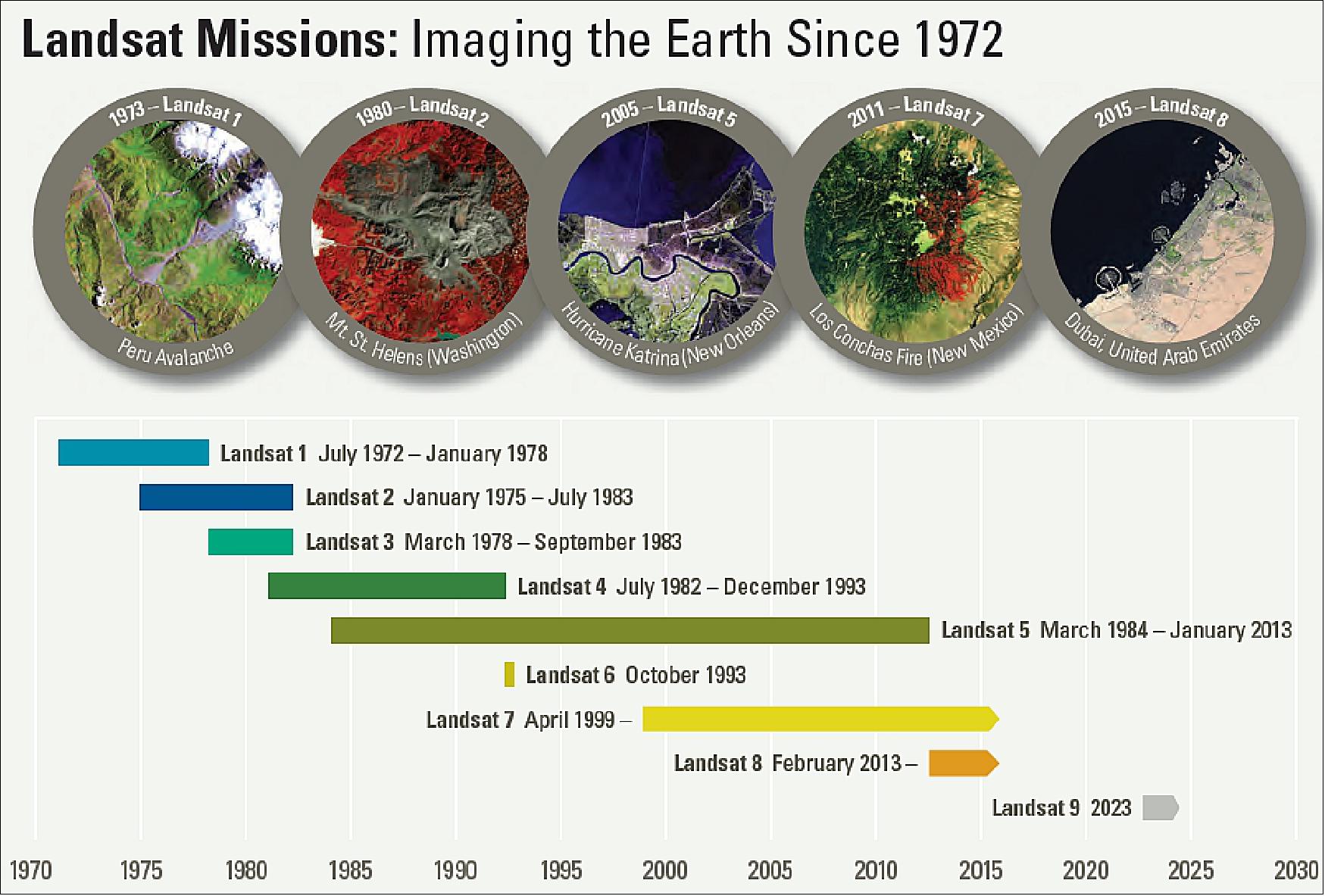
• October 12, 2015: The delta plain of the Mississippi River is disappearing. The lobe-shaped arc of coastal land from the Chandeleur Islands in eastern Louisiana to the Sabine River loses a football field’s worth of land every hour. Put another way, the delta has shrunk by nearly 5,000 km2 over the past 80 years. That’s as if most of Delaware had sunk into the sea. 44) Though land losses are widely distributed across the 300 km wide coastal plain of Louisiana, Atchafalaya Bay stands as a notable exception. In a swampy area south of Morgan City, new land is forming at the mouths of the Wax Lake Outlet and the Atchafalaya River. Wax Lake Outlet is an artificial channel that diverts some of the river’s flow into the bay about 16 km west of where the main river empties. This series of false-color satellite images chronicles the growth of the two deltas between 1984 and 2015. All of the images were acquired by instruments on Landsat satellites: the Thematic Mapper on Landsat 5, the Enhanced Thematic Mapper Plus on Landsat 7, and the Operational Land Imager on Landsat 8. A combination of shortwave infrared, near infrared, and green light was used to accentuate differences between land and water. Water appears dark blue; vegetation is green; bare ground is pink. All of the images were acquired in autumn, when river discharge tends to be low.

- Both deltas are being built by sediment carried by the Atchafalaya River. About 40 percent of the Atchafalaya’s discharge gets channeled through the Wax Lake Outlet. Even with the reduced flow, the Atchafalaya carries enough sediment to build land. According to one estimate by scientists from Louisiana State University (LSU), the Atchafalaya and Wax Lake Outlet deltas have combined to grow by 2.8 km2 per year. The rate of growth has varied considerably, mainly due to the timing of major floods and hurricanes. The land loss coincided with a period when hurricanes Allison, Isidore, and Lili battered Atchafalaya Bay and there were no major floods to replenish sediments. As is typical of deltas in southern Louisiana, the Atchafalaya and Wax Lake Outlet deltas have grown southward into the Gulf of Mexico. Vegetation is critical to maintaining new land because roots stabilize sediment and prevent erosion. The Atchafalaya delta has grown at a faster rate than its neighbor—about 1.6 km2 per year, versus 1.2 km2 per year for the Wax Lake delta. Due to the lack of dredging, Wax Lake delta is more natural in character, with a more symmetric, lobate shape. Instead of building new land along the mouth of the Mississippi, the controlled river sends jets of sediment-rich water directly into the relatively deep waters of the Gulf of Mexico and toward the edge of the continental shelf. With sea level rising rapidly due to natural geological processes, climate change, and human activities, predictions for the future of the Mississippi Delta are not optimal.
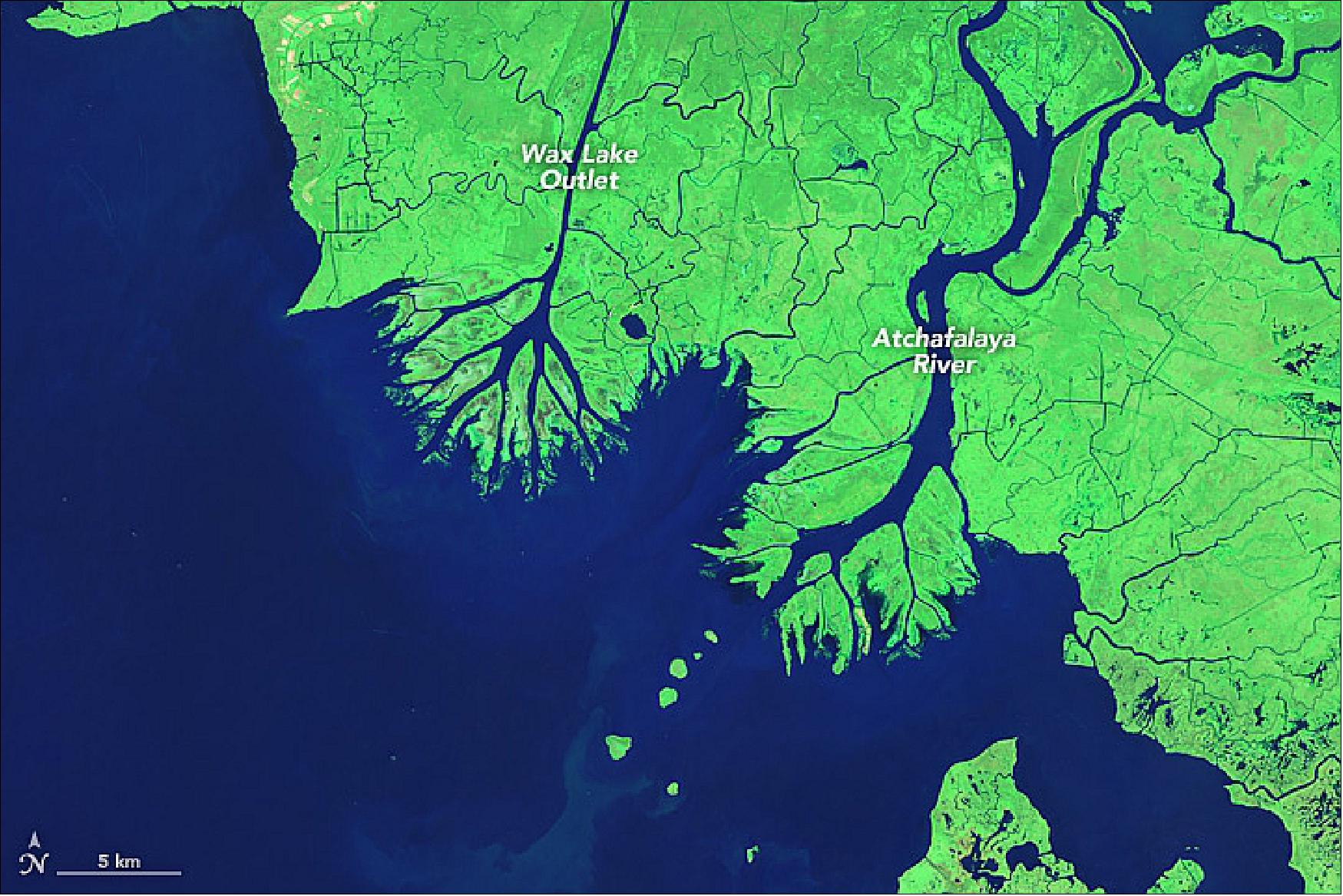
• April 15, 2015: Landsat-7 was on orbit for 16 years, well past its 5-year design life. A number of spacecraft components are being monitored (e.g., attitude control system, remote telemetry command box, power control unit) but overall, Landsat-7 is acquiring more imagery than at any point in its history (collecting about 475 scenes/day, ~22% of pixels are missing per scene (faulty scan-line corrector). 45) 46) Preliminary analysis shows that if Landsat-7 is authorized to continue imaging outside of its nominal 10:00 AM equator crossing time window, the mission could be extended until early 2020. As Landsat 5 orbit decayed to an approximate 9:15 AM orbit in the late 1990s, there is precedent for imaging outside the nominal crossing time.
• 2015: The LS-7 spacecraft was operational in 2015 with known degradations to the ETM+ instrument, collecting global data.
• April 2014: The Landsat-7 satellite had been observing Earth from outer space for 15 years. 47) At this point the Landsat-7 archive provided 1,708,353 scenes of ETM+ data which corresponded to ~ 1,586 TB Raw and L0Ra Data (average scene size of 487 MB). 49)
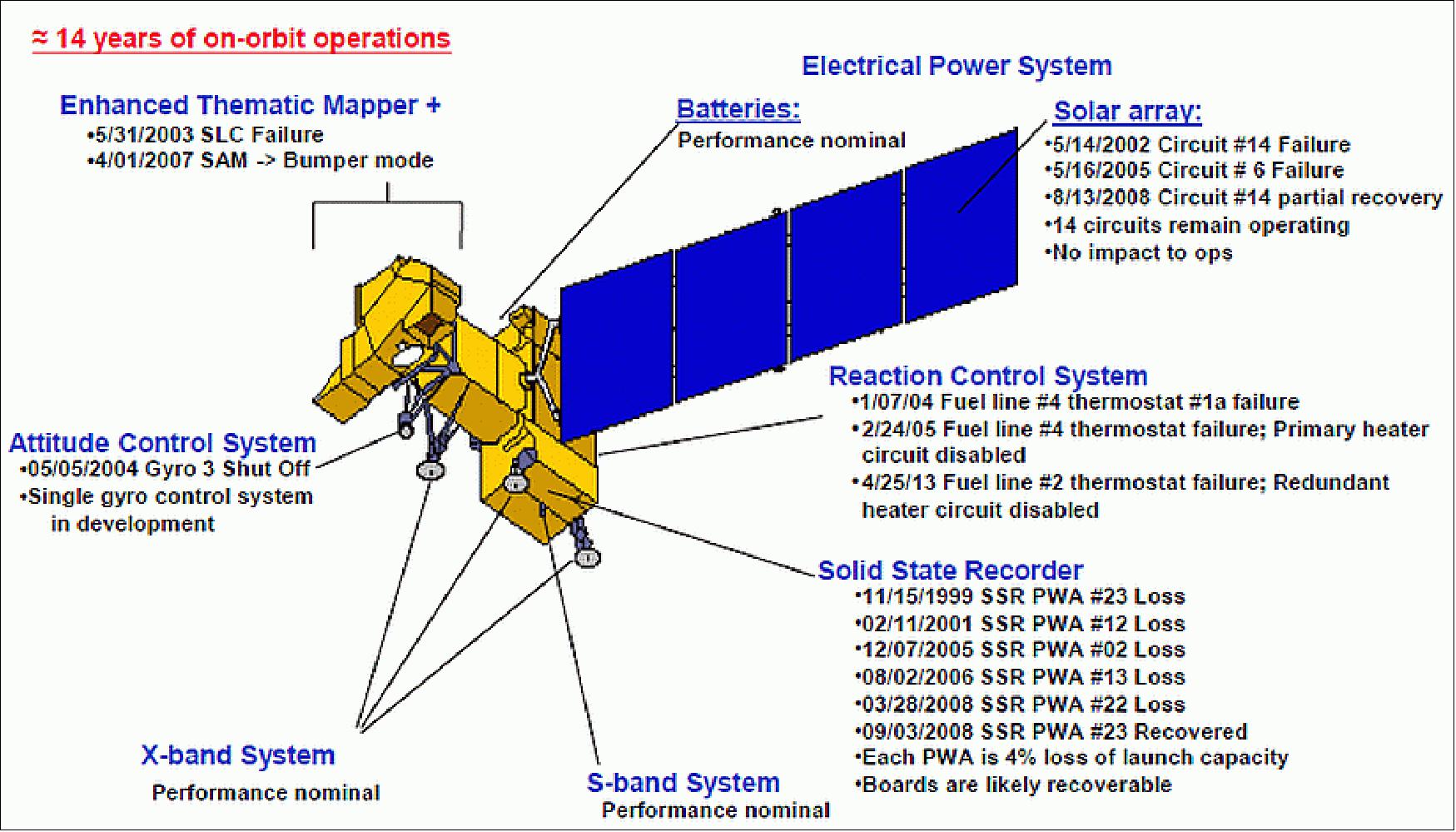
• January 2014: Landsat-7 continued its science mission and based on fuel estimates will continue to do so until at least mid-2016. Landsat-7, while its imagery is slightly degraded due to the scan-line corrector failure, continues to provide global coverage and an 8-repeat cycle for the Landsat Mission when combined with Landsat-8. 50)
• 2013: The LS-7 spacecraft was operational in 2013 with known degradations to the ETM+ instrument, collecting global data. 51)

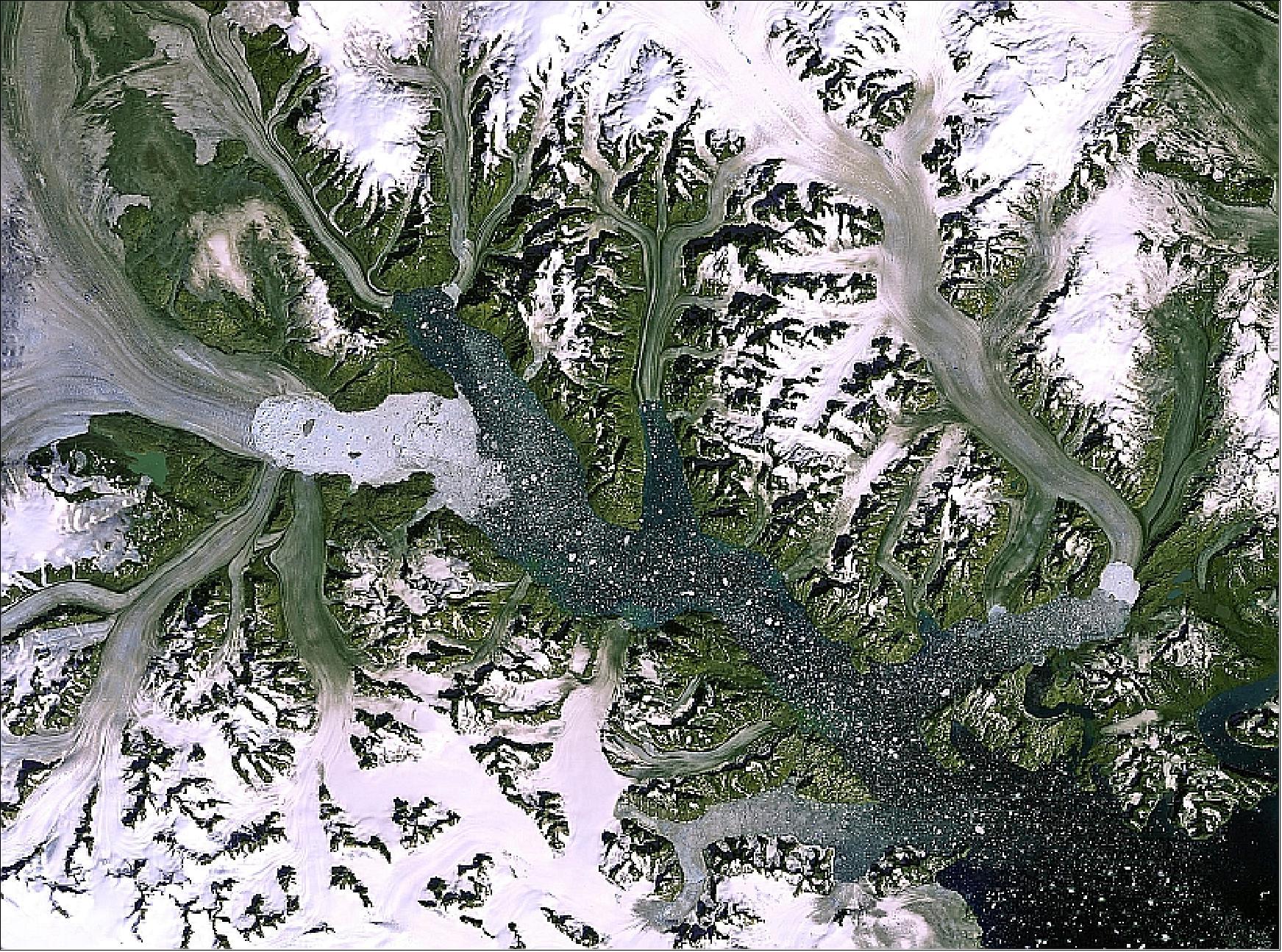
• July 23, 2012: The Landsat program marked its 40th anniversary, representing the longest continuous global record of Earth observations from space. Through four decades, Landsat satellites have taken specialized measurements of Earth's continents and surrounding coastal regions, enabling people to study many aspects of the planet and to evaluate the dynamic changes caused by both natural processes and human practices. The long record of Landsat spectral information is a historical archive unmatched in quality, detail, coverage, and length. 54) The data-rich USGS archive built from the Landsat satellites since 1972, with more than three million images, represents the surface of Earth over a 40-year period, a story of our physical world unparalleled in the history of science.
• 2012: The Landsat-7 spacecraft was operational with known degradations to the ETM+ instrument. 55)

Legend to Figure 34: The 3 Landsat images were captured by the Landsat-1, -4 and -7 satellites. Visible is the Lisan Peninsula (bottom center) that forms a land bridge through the Dead Sea. Deep waters are dark blue, while pale blue shows salt ponds and shallow waters to the south. The pale pink and sand-colored regions are desert lands. Denser vegetation appears bright red. - The expansion of massive salt evaporation projects on the Dead Sea are clearly visible in this time series of images taken by Landsat satellites operated by NASA and the USGS (U.S. Geological Survey). The USGS preserves a 40-year archive of Landsat images that is freely available data over the Internet.
• 2011: The Landsat-7 spacecraft was operational (11 years of on-orbit operations). 57)
• 2010: Landsat-7 was operational providing image data, however with the known degradations of the ETM+ instrument. Expendable fuel, necessary for stabilizing the orbit and angle of the satellite, will run out in 2012. 58) 59) 60)

• March 3rd/19th, 2006: In preparation for this event, the Landsat-7 flight operations team successfully tested bumper mode operation over several geometric calibration sites. Analysis of the preliminary data show the movement of one of the antennas impacts image acquisition, but this error can be corrected by the ground processing system. Additional in-depth research is underway, but this successful bumper mode test is a positive indicator for the continuation of the Landsat-7 mission.
• February 1, 2006: The Landsat-7 team developed and uploaded flight software that can act like a ”virtual” gyro -- ready to use if another gyro fails. The enhanced capability was designed, developed, tested, and implemented with no interference to ongoing Landsat-7 operations.
• May 5, 2004: The LS-7 project de-powered one of its gyros , due to indications of anomalous behavior. The spacecraft has three two-degrees-of-freedom gyros and needs two at any time to maintain attitude control. A risk assessment reported a 40 percent likelihood of another gyro failure by July 2005. A team was assembled to modify the software on board the spacecraft to operate in what is being termed Virtual Gyro (V-Gyro) mode. In this mode, if another gyro fails, the attitude control system would use the remaining gyro, along with existing onboard instrumentation and new control logic, to maintain attitude control.
• April 15, 2004: The Landsat-7 spacecraft and its payload reached the end of its five-year design life.
Ground Segment
Landsat-7 data is received and distributed to the user community by USGS (capturing and processing 250 Landsat scenes per day and delivering at least 100 of the scenes to users each day).NASA/GSFC performed on-orbit mission operations until Oct. 1, 2000; after that responsibility for flight operations and LS-7 management was transferred to the USGS/EDC (flight operations, maintenance, and management of all ground data reception, processing, archiving, product generation, and distribution). The operating philosophy changed to the effect that ETM+ data covering the global continental surfaces are being archived in the USA. The ETM+ archive is continually being updated as data become available. This data policy differs from the past (Landsat-4 and -5), where data was only acquired from the S/C on the basis of customer requests. The new archiving policy will substantially increase the amount of data available to the user community. 62) 63) 64)
The Landsat-7 ground system design includes an Image Assessment System (IAS) to provide users with ancillary information needed to generate useful, radiometrically calibrated and geometrically corrected ETM+ digital imagery. Another aspect of the new data handling policy is that ETM+ data will be distributed from the archive in an essentially raw form. Users are responsible for the task of preprocessing their imagery (i.e. radiometric and geometric corrections). The price tag for Landsat-7 imagery is substantially lower than for the commercial products of Landsat-4 and -5.
In April 2008, the USGS announced to open the Landsat-7 archive providing free access to the entire user community. Previously acquired imagery from Landsat 1 through Landsat 5, is also now available for download at no charge using the same standard processing format. The release schedule is shown in Table 8. 65)
As of early January 2009, over 225,000 scences were downloaded since Oct. 1, 2008. Newly acquired Landsat 7 ETM+ SLC-off and Landsat 5 TM images with less than 40 percent cloud cover are automatically processed and made available for immediate download. Imagery with greater than 40 percent cloud cover can be processed upon request. Once the requested scenes are processed, an email notification is sent to the customer with instructions for downloading. These scenes will then become accessible to all users. 66)
Data | Available over the Internet |
Landsat 7 – all new global acquisitions | July 2008 |
Landsat 7 – all data | September 2008 |
Landsat 5 – all TM data | December 2008 |
Landsat 4 – all TM data | January 2009 |
Landsat 1-5 – all MSS data | January 2009 |

The direct downlink service to a global network of existing Landsat ground stations (in X-band via each of three directional antennas) is maintained. All real-time image data (within view of a licensed ground station) are directly downlinked via the three X-band links. TDRSS may be used for TT&C data relays in S-band (backup). The prime (US) ground receiving station for the Landsat-7 archive is located at the EROS Data Center (EDC) in Sioux Falls, South Dakota. A second (US) reception facility near Fairbanks, AK, acquires coverage of Alaska and international coverage using the onboard recorder. An additional receiving station in Svalbard/Spitzbergen (Norway) provides backup reception. All data received at either Fairbanks or Svalbard is being shipped to EDC for archiving and distribution.

References
1) Special issue on Landsat Sensor Performance Characterization, IEEE Transactions on Geoscience and Remote sensing, Vol. 42, No 12, Dec. 2004
2) “Landsat: A Global Land-Imaging Project,” USGS, URL: http://pubs.usgs.gov
/fs/2010/3026/pdf/FS2010-3026.pdf
3) “Landsat-7 Science Data Handbook,” USGS, URL: http://landsathandbook.gsfc.nasa.gov/
4) K. Dolan, P. Sabelhaus, D. Williams, “Landsat-7 Extending 25 Years of Global Coverage,” Proceedings of Information for Sustainability, 27th International Symposium on Remote Sensing of Environment, Tromsoe, Norway, June 8-12, 1998, pp. 622-625
5) “Landsat-7 Science Data Users Handbook,” URL: http://landsathandbook.gsfc.nasa.gov/payload/
6) B. L. Markham, et al., “Radiometric Calibration of the Landsat-7 Enhanced Thematic Mapper Plus,” Proceedings of IGARSS '94, Volume IV, pp. 2004-2006
7) K. Thome, B. Markham, J. Barker, P. Slater, S. Biggar, “Radiometric Calibration of Landsat,” PE&RS, July 1997, pp. 853-858
8) J. R. Irons, D. L. Williams, B. L. Markham, “Landsat-7 ETM+ on-orbit calibration and data quality assessment,” Proceedings of IGARSS'95, Florence, Italy, July 10-14, 1995, pp. 1573-1575
9) B. L. Markham, D. L. Williams, J. R. Irons, “Landsat Sensor Performance: History and Current Status,” IEEE Transactions on Geoscience and Remote sensing, Vol. 42, No 12, Dec. 2004, pp. 2691-2694
10) D. S. Lee, J. C. Storey, M. J. Choate, R. W. Hayes, “Four Years of Landsat-7 On-Orbit Geometric Calibration and Performance,” IEEE Transactions on Geoscience and Remote sensing, Vol. 42, No 12, Dec. 2004, pp. 2786-2795
11) P. L. Scaramuzza, B. L. Markham, J. A. Basi, E. Kaita, “Landsat-7 ETM+ On-Orbit Reflective-Band Radiometric Correction,” IEEE Transactions on Geoscience and Remote sensing, Vol. 42, No 12, Dec. 2004, pp. 2766-2809
12) J. C. Storey, “Landsat 7 on-orbit modulation transfer function estimation,” Proceedings of SPIE, 'Sensors, Systems, and Next-Generation Satellites,' V, Hiroyuki Fujisada, Joan B. Lurie, Konradin Weber, Editors, Vol. 4540, 2001, URL: http://landsathandbook.gsfc.nasa.gov/pdfs/L7_MTF_Storey_SPIE2001.pdf
13) G. Chander, D. J. Meyer, D. L. Helder, “Cross Calibration of the Landsat-7 ETM+ and EO-1 ALI Sensor;” IEEE Transactions on Geoscience and Remote sensing, Vol. 42, No 12, Dec. 2004, pp. 2821-2831
14) J. G. Masek, M. Honzak, S. N. Goward, P. Liu, E. Pak, “Landsat-7 ETM+ as an observatory for land cover :Initial radiometric and geometric comparisons with Landsat-5 Thematic Mapper,” Remote Sensing of Environment Vol. 78, 2001, pp. 118-130
15) B. L. Markham, J. L. Barker, J. A. Barsi, E. Kaita, K. J. Thome, D. L. Helder, F. D. Palluconi, J. R. Schott, P. Scaramuzza, “Landsat-7 ETM+ Radiometric Stability and Absolute Calibration,” Proceedings of SPIE, 'Sensors, Systems, and Next-Generation Satellites VI,' Hiroyuki Fujisada, Joan B. Lurie, Michelle L. Aten, Konradin Weber, Editors, Vol. 4881, 2003
16) B. Markham, S. Goward, T. Arvidson, J. Barsi, P. Scaramuzza, “Landsat-7 Long-Term Acquisition Plan Radiometry - Evolution Over Time,” PE&RS (Photogrammetric Engineering & Remote Sensing), Vol. 72, Oct. 2006, pp. 1129-1135
17) T. Arvidson, S. Goward, J. Gasch, D. Williams, “Landsat-7 Long-Term Acquisition Plan: Development and Validation,” PE&RS, Vol. 72, Oct. 2006, pp. 1137-1146
18) S. Goward, T. Arvidson, D. Williams, J. Faundeen, J. Irons, S. Franks, “Historical Record of Landsat Global Coverage: Mission Operations, NSLRSDA, and International Cooperator Stations,” PE&RS, Vol. 72, Oct. 2006, pp. 1155-1168
19) Robert Tetrault, “Access and Availability of Resourcesat-1 AWiFS Data for Agriculture,” http://www.pecad.fas.usda.gov/pdfs/2006/RLT_Access_FAS_AgApnsWorkshop_Sept2006_REV20060927.pdf
20) Deanna Archuleta, “Department of the Interior’s Role in Earth Observation,” Proceedings of JACIE 2010 (Joint Agency Commercial Imagery Evaluation) Workshop, Fairfax VA, USA, March 16-18, 2010, URL: http://calval.cr.usgs.gov/JACIE_files/JACIE10/Presentations/TueAM/Archuleta_3-16-10_JACIE.pdf
21) ”Undercutting Sverdrup Glacier,” NASA Earth Observatory, Image of the Day for 26 January 2021, URL: https://earthobservatory.nasa.gov/images/147776/undercutting-sverdrup-glacier
22) ”Counting Trees in Africa’s Drylands,” NASA Earth Observatory, Image of the Day for 7 December 2020, URL: https://earthobservatory.nasa.gov/images/147612/counting-trees-in-africas-drylands
23) Martin Brandt, Compton J. Tucker, Ankit Kariryaa, Kjeld Rasmussen, Christin Abel, Jennifer Small, Jerome Chave, Laura Vang Rasmussen, Pierre Hiernaux, Abdoul Aziz Diouf, Laurent Kergoat, Ole Mertz, Christian Igel, Fabian Gieseke, Johannes Schöning, Sizhuo Li, Katherine Melocik, Jesse Meyer, Scott Sinno, Eric Romero, Erin Glennie, Amandine Montagu, Morgane Dendoncker & Rasmus Fensholt, ”An unexpectedly large count of trees in the West African Sahara and Sahel,” Nature Communications, Volume 587, pp. 78-82, October 2020, https://doi.org/10.1038/s41586-020-2824-5
24) Mitchell K. Hobish, Laura E. P. Rocchio, Darrel Williams, Samuel Goward, ”The Living Legacy of Landsat 7: Still Going Strong after 20 Years in Orbit,” NASA, The Earth Observer, July-August 2019, Volume 31, Issue 4, pp: 4-14, URL: https://eospso.nasa.gov/sites/
default/files/eo_pdfs/Jul_Aug_2019_final_color_508_0.pdf
25) Michael A. Wulder, Thomas R. Loveland, David P. Roy, Christopher J. Crawford,Jeffrey G. Masek, Curtis E. Woodcock, Richard G. Allen, Martha C. Anderson,Alan S. Belward, Warren B. Cohen, John Dwyer, Angela Erb, Feng Gao, Patrick Griffiths,Dennis Helder, Txomin Hermosilla, James D. Hipple, Patrick Hostert, M. Joseph Hughes,Justin Huntington, David M. Johnson, Robert Kennedy, Ayse Kilic, Zhan Li, Leo Lymburner,Joel McCorkel, Nima Pahlevan, Theodore A. Scambos, Crystal Schaaf, John R. Schott,Yongwei Sheng, James Storey, Eric Vermote, James Vogelmann, Joanne C. White,Randolph H. Wynne, Zhe Zhu, ”Current status of Landsat program, science, and applications,” Remote Sensing of Environment, Volume 225, May 2019, Pages 127-147, https://doi.org/10.1016/j.rse.2019.02.015 , URL: https://reader.elsevier.com/reader/sd/pii/S0034425719300707?
token=A77033BD9D286A27CDBD7B3B9ED6D2C296CA379CB
2C3105DEFB6C55AB7A3448BACC4594FCEF5663E0D95F416A8F2A75A
26) For more information on NAFD, visit: https://web.archive.org/web/20201022203536/https://landsat.gsfc.nasa.gov/watching-a-quarter-century-of-north-american-forest-dynamics-with-landsat/
27) https://sspd.gsfc.nasa.gov/restore-L.html
28) https://web.archive.org/web/20201030010448/https://landsat.gsfc.nasa.gov/how_landsat_helps/
29) ”Mapping the Land Between the Tides,” NASA Earth Observatory, 2 July 2019, URL: https://earthobservatory.nasa.gov/images/145237/mapping-the-land-between-the-tides?src=eoa-iotd
30) ”The Urbanization of Ulaanbaatar,” NASA Earth Observatory, 4 June 2019, URL: https://earthobservatory.nasa.gov/images/145125/the-urbanization-of-ulaanbaatar?src=eoa-iotd
31) ”KBRwyle Awarded $19M to Perform Flight Ops for USGS Satellite That Fights World Hunger, Helps Earth Flourish,” PR Newswire, 21 February 2019, URL: https://www.prnewswire.com/news-releases/
kbrwyle-awarded-19m-to-perform-flight-ops-for-usgs-satellite-
that-fights-world-hunger-helps-earth-flourish-300799280.html
32) ”Daily Operations of Landsat 7,” USGS, Published on Jul 18, 2018, URL: https://www.youtube.com
/watch?v=OGTbWK06FX4&feature=youtu.be
33) ”Glacier Retreat at Cook Ice Cap,” NASA Earth Observatory, 27 April 2018, URL: https://earthobservatory.nasa.gov/IOTD/view.php?id=92059&src=eoa-iotd
34) ”Peru’s Newest National Park,” NASA Earth Observatory, 22 March 2018, URL: https://earthobservatory.nasa.gov/IOTD/view.php?id=91871
35) ”The Spread of Mangroves in Senegal,” NASA Earth Observatory, 21 march 2018, URL: https://earthobservatory.nasa.gov/IOTD/view.php?id=91867
36) ”September 14, 2017 – Landsat 7 Anomaly Resolution,” USGS, URL: https://www.usgs.gov/landsat-missions/september-14-2017-landsat-7-anomaly-resolution
37) ”Fire Scars the Okefenokee,” NASA Earth Observatory, May 11, 2017, URL: https://earthobservatory.nasa.gov/IOTD/view.php?id=90214&src=iotdrss
38) ”Cambodia’s Forests Are Disappearing,” NASA Earth Observatory, Jan. 10, 2017, URL: http://earthobservatory.nasa.gov/IOTD/view.php?id=89413
39) Todd A. Schroeder, Thomas R. Loveland, Michael A. Wulder, James R. Irons, ”Landsat Science Team: 2016 Summer Meeting Summary,” The Earth Observer, Volume 28, Issue 6, November-December 2016, pp: 45-50, URL: https://eospso.nasa.gov/sites/default/files/eo_pdfs/Nov-Dec%202016%20color%20508.pdf
40) ”NASA’s Restore-L Mission to Refuel Landsat-7, Demonstrate Crosscutting Technologies,” NASA Technology, June 23, 2016, URL: [web source no longer available]
41) ”Celebrating 50 years of Landsat Vision,” USGS, Sept. 21, 1966, URL: http://landsat.usgs.gov
/project-eros-idea-worked.php
42) A. Azabdaftari , F. Sunar, ”Soil salinity mapping using multitemporal Landsat data,” Proceedings of the XXIII ISPRS ((International Society for Photogrammetry and Remote Sensing) Congress, Prague, Czech Republic, July 12-19, 2016, doi:10.5194/isprsarchives-XLI-B7-3-2016, URL: http://www.int-arch-photogramm-remote-sens-spatial-inf-sci.net/XLI-B7/3/2016/isprs-archives-XLI-B7-3-2016.pdf
43) Landsat—Earth Observation Satellites, USGS, Nov. 2015, URL: http://pubs.usgs.gov/fs/2015/3081/fs20153081.pdf
44) Adam Voiland, ”Growing Deltas in Atchafalaya Bay,” NASA Earth Observatory, Oct. 12, 2015, URL: http://earthobservatory.nasa.gov/Features/WorldOfChange/wax_lake.php
45) Guy Thayer, “Landsat-7 status,“ in 'Landsat Science Team Meeting: Winter 2015,' The Earth Observer, May-June 2015, Volume 27, Issue 3, p. 20, URL: http://eospso.nasa.gov/sites/default/files/eo_pdfs/May_Jun_2015_color_508.pdf
46) Tim Newman, “Land Remote Sensing Program Update,” USGS, March 18, 2015, URL: https://www.fgdc.gov/ngac/meetings/march-2015/landsat-program-update-ngac-march-2015.pdf
47) John Campbell, “Space Veteran Landsat 7 Marks 15 Years of Observing Earth,” USGS, April 15, 2014, URL: http://www.usgs.gov/blogs/features/usgs_top_story/
space-veteran-landsat-7-marks-15-years-of-observing-earth/
48) Jenn Sabers Lacey, “USGS EROS Center - 40 Years of Service to our Plnat,” Proceedings of JACIE 2014 (Joint Agency Commercial Imagery Evaluation) Workshop, Louisville, Kentucky, USA, March 26-28, 2014, URL: https://calval.cr.usgs.gov/wordpress/wp-content/
uploads/J-Lacey-ASPRS-JACIE-Landsat-March-26-2014-Final1.pdf
49) Jenn Sabers Lacey, “USGS EROS Center - 40 Years of Service to our Plnat,” Proceedings of JACIE 2014 (Joint Agency Commercial Imagery Evaluation) Workshop, Louisville, Kentucky, USA, March 26-28, 2014, URL: https://calval.cr.usgs.gov/wordpress/wp-content/
uploads/J-Lacey-ASPRS-JACIE-Landsat-March-26-2014-Final1.pdf
50) Information provided by James M. Lacasse of USGS Climate and Land Use Change, Sioux Falls, S.D., USA
51) Tom Holm, “Landsat: Building a Future on 40 Years of Success - Status: Landsat 7,” 12th Annual JACIE (Joint Agency Commercial Imagery Evaluation) Workshop , St. Louis, MO, USA, April 16-18, 2013, URL: http://calval.cr.usgs.gov/wordpress/wp-content/uploads/JACIE-LDCM-Status-and-Overview-v4.pdf
52) “LDCM Underfly with Landsat 7,” USGS, March 29, 2013, URL: http://landsat.usgs.gov/LDCM_Underfly_with_Landsat_7.php
53) http://www.esa.int/Our_Activities/Observing_the_Earth/Earth_from_Space_Water_and_ice
54) Joel N. Shurkin, “Landsat Looks and Sees,” Space Daily, July 23, 2012, URL: http://www.spacedaily.com/reports/Landsat_Looks_and_Sees_999.html
56) Aries Keck, “NASA Sees New Salt in an Ancient Sea,” Space Daily, April 11, 2012, URL: http://www.spacedaily.com/reports/NASA_Sees_New_Salt_in_an_Ancient_Sea_999.html
57) Kristi Kline, Rachel Headley, “Landsat Project StatusLandsat,” Proceedings of the Landsat Science Team Meeting, Mesa, AZ, USA, March 1-3, 2011, URL: http://landsat.usgs.gov
/documents/Kline%20-%20Landsat%20Status%20-%20LST%203-2011.pdf
58) Information provided by Ronald E. Beck of USGS
59) Thomas Kalvelage, “Landsat Project Status, Landsat Science Team Meeting,” Jan. 19-21, 2010, Mountain View, CA, USA, URL: http://landsat.usgs.gov/documents/
Jan_2010_ScienceTeamMeeting_LandsatOverview_TomK_final_Jan19.pdf
60) “Landsat Science Team Meetings,” URL: http://landsat.usgs.gov/science_LST_Team_Meetings.php
61) J. A. Barsi, B. L. Markham, D. L. Helder, G. Chander, “Radiometric calibration status of Landsat-7 and Landsat-5,” Proceedings of. SPIE, Vol. 6744, Florence, Italy, Sept. 17-20, 2007, URL: http://landsat.gsfc.nasa.gov/pdf_archive/publications/SPIE07_LandsatRadiometry.pdf
62) J. R. Irons, D. L. Williams, B. L. Markham, “Landsat-7 ETM+ On-Orbit Calibration and Data Quality Assessment,” Proceedings IGARSS '95, Vol. II, pp. 1573-1575
63) W. C. Draeger, T. M. Holm, D. T. Lauer, R. J. Thompson, “The Availability of Landsat Data: Past, Present and Future,” PE&RS, July 1997, pp. 869-875
64) R. A. Williamson, “The Landsat Legacy: Remote Sensing Policy and the Development of Commercial Remote Sensing,” PE&RS, July 1997, pp. 877-885
65) “Imagery for Everyone -- Timeline Set to Release Entire USGS Landsat Archive at No Charge,” April 21, 2008, USGS, URL: http://landsat.usgs.gov/documents/USGS_Landsat_Imagery_Release.pdf
66) http://landsat.usgs.gov/mission_headlines2009.php
67) “International Ground Station (IGS) Network,” USGS, URL: http://landsat.usgs.gov/about_ground_stations.php
68) Landsat 7 Data Acquired at a Lower Orbit Now Available | U.S. Geological Survey. (n.d.). www.usgs.gov. Retrieved October 31, 2022, URL: https://www.usgs.gov/landsat-missions/news/landsat-7-data-acquired-lower-orbit-now-available
69) Landsat 7 Nominal Science Mission Ending | U.S. Geological Survey. (n.d.). www.usgs.gov. Retrieved May 03, 2024, URL: https://www.usgs.gov/landsat-missions/news/landsat-7-nominal-science-mission-ending
Spacecraft Launch Sensor Complement Mission Status Ground Segment References Back to top