MTI (Multispectral Thermal Imager)
EO
Multiple direction/polarisation radiometers
Atmosphere
Earth radiation budget radiometers
Quick facts
Overview
Mission type | EO |
Agency | NNSA |
Mission status | - |
Launch date | 04 Mar 2000 |
End of life date | 04 Mar 2015 |
Measurement domain | Atmosphere, Gravity and Magnetic Fields |
Measurement category | Gravity, Magnetic and Geodynamic measurements, Ozone, Atmospheric Winds, Lightning Detection |
Measurement detailed | Atmospheric stability index, Electron density profile, Gravity field, Gravity gradients, Visibility, Electric Field (vector) |
Instrument type | Multiple direction/polarisation radiometers, Earth radiation budget radiometers, Space environment, Other, Scatterometers, Atmospheric chemistry, Communications, Data collection, Lightning sensors |
CEOS EO Handbook | See MTI (Multispectral Thermal Imager) summary |
MTI (Multispectral Thermal Imager)
MTI (also referred to as P97-3) is a US DOE-funded (Department of Energy) satellite mission, an R&D program and a technology demonstration mission of DOE/NNSA (National Nuclear Security Administration) with the objective to develop a broad range of new technologies, and to demonstrate the efficacy of highly accurate multispectral imaging for passive characterization of industrial facilities and related environmental impacts from space.
The MTI program is managed by SNL (Sandia National Laboratories), LANL (Los Alamos National Laboratory) and SRTC (Savannah River Technology Center), representing DOE National Laboratories. Other program participants include: USAF STP (Space Test Program) launch provision, AFRL (Air Force Research Laboratory), and universities. Major industrial partners are: Raytheon Optical Systems, Ball Aerospace, SBRS (Santa Barbara Remote Sensing), and TRW. Within STP, the MTI mission is designated as P97-3. 1) 2)
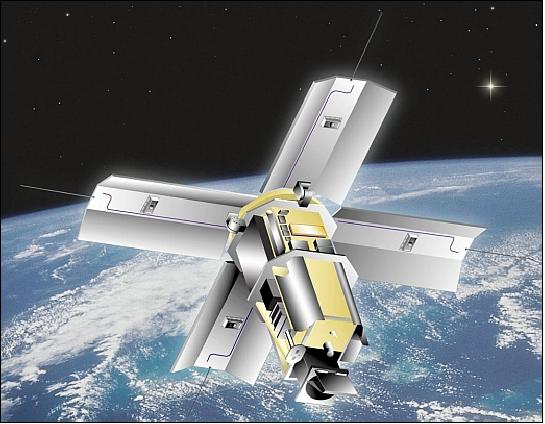
Spacecraft
The MTI satellite, with the bus built and integrated by Ball Aerospace (BCP2000 bus version), is three-axis stabilized. The spacecraft bus is an aluminum frame and shear panel design structure, the dimensions (stowed for launch) are: 1.4 m diameter and 2.6 m in height.
The S/C attitude is measured by multiple sun sensors, a horizon sensor, and gyros. Actuators are reaction wheels and torque rods. A pointing accuracy of 0.25º is provided. The S/C is agile and can be body-pointed up to ±50º in all axis. This feature provides a FOR (Field of Regard) of ±200 km for cross-track coverage (the nominal swath width of the imager is 12 km). The body-pointing feature may also be used for along-track stereo imaging support. The system does not have propulsion, so the orbit decays over time.
The S/C mass at launch is 614 kg, average total power of 575 W, with 11 battery cells (plus one spare) for ecliptic operations support. The largest single power consumption due to the Stirling cycle cryogenic cooler which cools the focal plane and adjacent structures. The S/C design life is one year with a goal of three years. 3) 4)
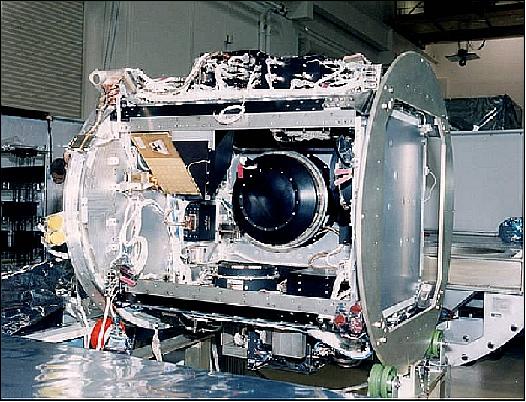
Launch
A launch of MTI on a Taurus vehicle took place on March 12, 2000 from VAFB, CA, USA. The launch was funded by the Air Forces' Space Test Program (STP).
Orbit
Sun-synchronous near-circular polar orbit, altitude = 575 km x 609 km, inclination = 97.52º, period = 96.6 min, with a local equatorial crossing time of 13:00 on ascending node. The S/C has no orbit maintenance capability, resulting in an orbit decay over time.
RF communications
Downlink data transmission in X-band at 8 Mbit/s. The TT&C communication is in S-band with an uplink rate of 2048 bit/s. The CCSDS protocol is used for uplink and downlink communication. S/C operations are performed at SNL (ground station). The DPAC (Data Processing and Analysis Center) function is provided by LANL. 5) 6)
Status of Mission
• In 2011, the MTI spacecraft and its payload are operating nominally.
• On March 12, 2010, the MTI spacecraft marked its 10th anniversary of service as it completed its 55,000th orbit — far exceeding both its intended maximum life and its potential applications. 7) 8)
Originally only intended for a one- to three-year mission, the MTI satellite was designed to provide highly accurate radiometry with good spatial resolution in 15 spectral bands and measure ground temperatures from space with accuracies in the realm of one kelvin. Analysts armed with advanced computer simulation codes and expertise can then employ these precision radiometric and thermal imaging capabilities to research phenomenology associated with nuclear proliferation. The temperature data collected by the MTI satellite is important because heat is a characteristic that is difficult to hide and serves as a strong indicator of a facility’s function and throughput — producing power or nuclear weapons material.
In addition to numerous defense-related applications, the more than 100 members of the MTI users group have employed the satellite’s imagery for research in volcanology, glaciology, entomology, climatology, and the study of the moon. With sponsorship secured for the foreseeable future of the MTI satellite, the project team looks forward to years of continued work supporting research and development interests.
• In 2007, the MTI spacecraft and its payload (MTI) are operational. In particular, MTI was used to support experiments (campaign style) for the last 7 years. Due to funding constraints MTI has currently very limited usage. 9)
• In June 2003, the MTI mission completed its nominal mission research goal of 3 years of operations. The satellite continues to collect imagery for US government agencies. The pulse-tube cryocooler operated continuously for 30 months keeping the FPA of the MTI instrument cooled to 75 K. This is one of the longest continuously running mechanical on-orbit coolers ever fielded. 10) 11)
• The IRU (Inertial Reference Unit) failed after three years of operation.
• The HXRS instrument stopped working in February 2003, i.e. after almost 3 years of successful operation. The reason of its technical failure is not known, its expected lifetime was 2 years. 12)
• Loss and recovery of the MTI telescope controller: The MTI spacecraft suffered a nearly fatal malfunction in part due to subtle ground command handling issues. The ground crew sent commands in two parts: “clear register” followed by “execution.” However, the command checking process would not automatically preclude “execution” in case the “clear” command was not carried out.
Late in 2001, a command was issued when the satellite was near the horizon. The “clear” instruction did not go through, but the “execution” did. Unfortunately, this particular “execution” command, when combined with the register contents left over from a previous command, caused the flight computer to freeze. Several stored command sequences backed up.
Fortunately, MTI's fail-safe management system automatically sprang the aperture door open and moved the calibration wheel clear. Observations could still be made, and the program subsequently developed a different calibration approach so the mission could be continued. 13)
• During the total lunar eclipse of 9 January 2001 (mid-eclipse 20:21 UT), MTI imaged the moon while it was in partial and full eclipse. 14)
• The checkout of the spacecraft and its payload was completed on June 14, 2000 and commencement of the operational period. 15) 16)
• The first image was obtained on March 28, 2000.
• Shortly after launch a glitch in the satellite's on-board power system resulted in unexpected battery discharges that prevented data from being collected and transmitted to the ground station. The flight team soon learned to manage its power operations in a way that mitigated the problem.
Sensor Complement
The overall instrument design objective is to support and evaluate advanced multispectral and thermal imaging, image processing and associated technologies. The instrument consists of the following elements: a telescope assembly (36 cm unobscured clear aperture, built by Raytheon Optical Systems, Danbury, CT), a cryogenically cooled focal plane array (FPA) with 15 linear spectral-sensitive pushbroom detector arrays, a complex built-in calibration hardware designed to collect radiometrically calibrated images with accuracies currently achievable only in the laboratory, and associated readout and control electronics. Optical filters define 15 spectral bands, ranging from the visible to the thermal infrared (TIR). The nominal FOV = 12 km x 12 km. 17) 18) 19) 20) 21)
The project combines and advances five technologies:
1) Multispectral imaging
2) Thermal imaging
3) Advanced radiometric calibration
4) Atmospheric characterization
5) Modeling and analysis.
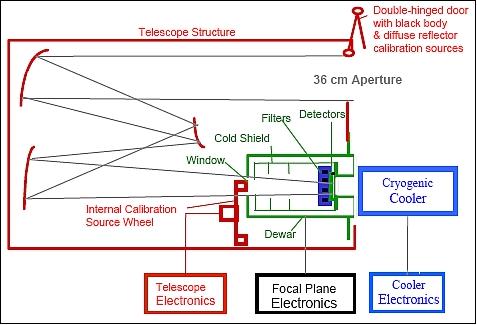
The Optical Assembly (OA), built by Raytheon Optical Systems, is shown in the cutaway view of Figure 5. This assembly includes the telescope structure, optics, calibration sources built into the double hinged aperture door and internal wheel assembly, focus mechanism (not shown), various actuators and other mechanisms, and numerous thermistors and heaters for temperature control.
The OA design features a 36 cm, 3-mirror, off-axis, anastigmatic, f/3.5 telescope design, housed in a composite structure for dimensional stability. The unobscured design offers near diffraction limited performance as shown in Figure 4. It also eliminates scattering and thermal emissions from structures that would be within the field of view of the focal plane arrays in an on-axis design. The design also provides an accessible exit pupil (Lyot stop) that permits effective cold shielding to thermally isolate the focal plane arrays from the telescope structure and internal components. The entire OA, including its internal components, is therefore uncooled, but thermally isolated from the rest of the satellite and temperature-controlled at 275 K. 22)

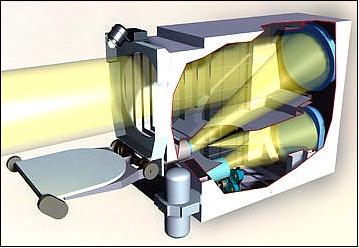
Legend to Figure 5: This cutaway reveals the optics. The primary, secondary and tertiary mirrors can be seen inside. The muzzle of the focal plane assembly dewar and the internal calibration wheel can also be seen. Outside the the double-hinged door and solar ratioing radiometer (opposite the door hinges) are also shown. The cryogenic cooler is also shown, although it is not part of Optical Assembly.
Figure 6 is a cutaway line drawing of the FPA (Focal Plane Assembly), built by Santa Barbara Research Center. At its heart are six SCAs (Sensor Chip Assemblies) mounted on three motherboards as shown in Figure 7. Each SCA consists of photosensitive detector material and a silicon ROIC (Readout Integrated Circuit). For bands A-D, the detectors and ROIC are implemented as a monolithic structure. For bands E-O, the photosensitive material is bump bonded to the ROIC. The ROIC provides preamplifiers for each pixel and circuitry for serializing pixel outputs onto analog output lines.
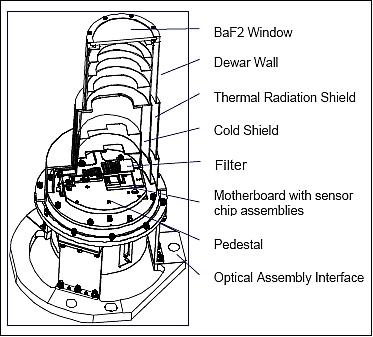

Imaging of a nominal nadir ground sample time in the along-track direction takes 715 µs for bands A-D, and 2.86 ms for bands E-O. The integration times for all bands are variable, up to 12 µs less than the pixel readout rate. A single detector array scans a 12 km image in about 1.7 seconds. The detectors are staggered in the along-track direction, so the system images a given ground point at slightly different times for each spectral band. A single 12 km x 12 km image requires about 4.5 s. - An average revisit time of one week for all target areas considered is provided with the body-pointing feature of the spacecraft.
MTI's nominal pixel GSD (Ground Sampling Distance) is 5 m at visible wavelengths and 20 m in the infrared. This fine spatial resolution and a special cirrus detection channel at 1.38 µm eliminate most of the problems connected to unresolved sub-pixel cloudiness. The MTI instrument is well suited for measurement of local and regional scale environmental variables with a swath width of 12 km and capability to image up to 48 km long strips. 23)
The pulse-tube cryocooler is a demonstration device of Northrop Grumman (formerly TRW) manufacture (flight qualification on MTI). It provides FPA cooling of > 3 W. 24)
Spectral band | Spectral range (µm) | GSD, (m) | Radiometric | NER | Detector |
A | 0.45 - 0.52 VIS, Blue | 5 | 3 | 4.4 10-5 | Si-PIN |
E | 0.86 - 0.90 NIR, Water vapor ref. | 20 | 3 | 3.0 10-5 | InSb |

MTI's spectral bands are carefully selected to collect data needed to derive a broad range of information on facilities and activities, including surface temperatures, materials, water quality, and vegetation health. To enhance accuracy, additional bands provide simultaneous information on atmospheric water vapor, aerosol content, and sub-visual cloud presence. Imaging the Earth's surface through the atmosphere presents many challenges.
The spectral coverage of MTI permits daytime and nighttime observations. The MWIR and TIR bands are in particular suited for nighttime measurements. During its three-year demonstration mission, MTI records periodically images of participating government, industrial, and natural sites, mostly within the continental US. These sites are instrumented to record ground-truth data by a team of scientists and technicians from SRTC (Savannah River Technology Center) and by site owners and operators.
Optical telescope assembly built by Hughes Danbury Optical Systems, Danbury, CT | 36 cm aperture, off-axis TMA (Three Mirror Anastigmatic) design, f/3.5, |
FPA (Focal Plane Assembly) built by SBRS | Consists of SCAs (Sensor Chip Assemblies), optical filters, and a vacuum enclosure; six SCAs are mounted on 3 motherboards; 3 SCA pairs are needed to provide cross-track coverage in all bands |
Detectors at 75 K | Monolithic silicon PIN diodes for VNIR bands |
Cryogenic cooler | Pulse-tube cryogenic cooler (>3 W of cooling at 65 K) |
Calibration sources | Blackbody, QLCS (Quick Look Calibration Source), QTH (Quartz Tungsten Halogen) |
Readout/control electronics | 12 bit data quantization, 266 Mbit/s of source data rate (all bands), |
Mass storage unit | 4.1 Gbit capacity |
Instrument mass, power | 240 kg, 350 W |
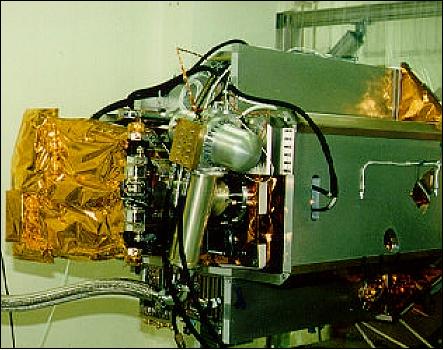
MTI Instrument Calibration
The strategy is based on accurate pre-launch calibration in the Los Alamos Radiometric Calibration Facility, plus on-orbit maintenance using sources built into the payload plus vicarious calibrations. The built-in calibration system uses instrumented ground sites, the sun, the moon and cold space looks to maintain long-term calibration. A more limited calibration sequence is performed before and after each imaging sequence. The TIR bands of MTI can be used to retrieve SST (Sea Surface Temperature). 25) 26) 27) 28) 29)
Calibration and testing of the MTI imager has frequently made use of instrumented ground sites such as DOE's ARM (Atmospheric Radiation Monitoring) locations in the Southern Great Plains, the TWP (Tropical Western Pacific) site, and the ACRF (ARM Climate Research Facility) North Slope of Alaska and Adjacent Arctic Ocean (NSA/AAO) site and other nearby areas of interest at the convenience of the MTI project. In addition to Earth-based observations, MTI routinely images the moon.

The program's considerable research effort consists of simultaneous data gathering from instrumented cooperative ground facilities and the satellite which serves to improve and validate algorithms for characterizing facility processes from remotely measurable data.
Water vapor is an important variable constituent of the atmosphere. The MTI has three NIR (Near Infrared) bands, E, F, and G, within the 850-1050 nm spectral range, that are used for the CWV (Columnar Water Vapor) retrieval using the CIBR (Continuum Interpolated Band Ratio) and the APDA (Atmospheric Precorrected Differential Absorption) methods. The basis of the NIR algorithms used for CWV retrieval is to measure the top of the atmosphere outgoing radiances within a chosen water vapor absorption band, and just outside this absorption. The ratios of out-of absorption and in absorption radiances are then related to the amount of water vapor along the path. A complication arises due to atmospheric aerosols and due to non-linear spectral variability of the ground reflectance. 30) 31)
Note: The annual average of CWV varies between 0.25 g/cm2 in the polar regions to over 5 g/cm2 in the tropics.
There are restrictions on the dissemination of MTI raw data. Users of MTI raw data must be US citizens with approved MTI projects, who have signed appropriate non-distribution agreements. DOE has established an MTI Users Group, comprising many researchers from 50 national defense and civilian agencies.

HXRS (Hard X-Ray Spectrometer)
The instrument is a joint endeavour by the Astronomical Institute of the Academy of Sciences of the Czech Republic (ASU) and NOAA/SEC (Space Environment Center) in Boulder, CO. The instrument was built in Prague by Space Devices, Ltd. HXRS has the following objectives: a) to record with a high time-resolution a rare species of solar flare associated with high-energy proton storms known to damage satellites and potentially endanger astronauts, b) to evaluate the efficiency of this type of instrument to predict interplanetary proton events, and c) to test the effectiveness of new shielding methods. NOAA hopes to obtain information needed to design a system capable of forecasting such storms. 32) 33) 34) 35) 36)
HXRS measures hard X-ray emissions of the sun in eight energy bands with time resolutions of up to 200 ms. The instrument consists of two identical scintillation detectors, one with shielding and one without, mounted side by side. Shielding is provided by a strong permanent magnet and a thick layer of plastic material. Each detector consists of a NaI crystal (25 mm diameter, 4.5 mm thick) coupled with a photomultiplier (Hamamatsu). Note: NaI (Tl) is a crystal of sodium-iodide activated by thallium. Such crystals are commonly used as X-ray detectors (coupled with photomultipliers).
Each detector is automatically calibrated in-flight by means of a radioactive source (AM241) on a movable arm. A 16-bit microprocessor controls all instrument functions and operations. The instrument data rate depends very much on the solar activity, i.e. on the number of hard X-ray events. During an event when all energy bands register with 200 ms time resolution, there are 10 channels that are read at 5 times/s (or 50 words/s). During quiet periods the data rate is about 1/10. The HXRS instrument mass is 7.695 kg, the power consumption is 5 - 6.5 W.
Incident X-ray photons create optical pulses in the crystals, they are converted into electrical signals and amplified by the photomultipliers. Pulse height (amplitude) discriminators measure the signal which is proportional to the incident photon energy. The number of pulses in each energy band counted per observation time interval is registered and send to the telemetry processor.

The MTI S/C spends a major portion of its time in standby mode (i.e., when the MTI instrument is not observing); during standby the S/C is always sun-pointing within 1º to collect solar energy. HXRS observations coincide with this standby mode.
Band Number | Energy Range (keV) | Max. Counts | Band Number | Energy Range (keV) | Max. Counts |
S0 | 12.6 - 19.0 | 65535 | S5 | 100.2 - 147.2 | 2047 |
S1 | 19.0 - 29.0 | 32767 | S6 | 147.2 - 219.5 | 1023 |
S2 | 29.0 - 44.0 | 16383 | S7 | 219.5 | 511 |
S3 | 44.0 - 67.2 | 8191 | S8 | 249.5 | 255 |
S4 | 67.2 - 100.2 | 4095 | SA | 12.6 | 65535 |
The “S” in “Band Number” of Table 3 signifies a “shielded detector.” In parallel, the same energy bands are also part of the other (non-shielded) detector of the instrument.
Operational modes of HXRS:
• Observing mode: BDN (Band Division Normal)
• Observing mode BDS (Band Division Shifted)
• Technical mode: Provision of instrument status
• Calibration mode: Each detector is calibrated independently but not simultaneously with the others.

MTI Ground Segment
DPAC (Data Processing and Analysis Center) is the central repository for algorithms, data, metadata, and general analysis tools of MTI at LANL (Los Alamos National Laboratory). The DPAC processes all raw MTI data to produce several levels of data products. 37) 38)

The DPAC automated pipeline is designed to produce all Level 0 products and most of Level 1. The major features of the automated pipeline include:
1) Automatic processing of data through Level 0 by simply placing a raw data file in the appropriate location. No user intervention is necessary for this processing to occur.
2) Automatic generation of Level 1 and higher processing requests when Level 0 is complete.
3) Input from the database to guide the processing, as well as output to the database to monitor progress and post results.
4) All the facilities needed to run the data multiple times (e.g., when new versions of processing algorithms are developed).
References
1) P. G. Weber, B. C. Brock, A. J. Garrett, B. W. Smith, C. C. Borel, W. B. Clodius, S. C. Bender, R. R. Kay, M. L. Decker, “Multispectral Thermal Imager Mission Overview,” Proceedings of SPIE, Imaging Spectroscopy V, Vol 3753-39, Denver , CO, July 19-21, 1999, pp. 340-346, URL: http://cborel.net/weber_spie799a.pdf
2) R. Rex Kay, Brian C. Brock, Tammy D. Henson, Jeffrey L. Rienstra, Max L. Decker, N. Glenn Rackley, Paul G. Weber, Steven C. Bender, Donald A. Byrd, “An Introduction to the Department of Energy’s Multispectral Thermal Imager (MTI) Project Emphasizing the Imaging and Calibration Subsystems,” 12th AIAA/USU Conference on Small Satellites, Logan, Utah, USA, Aug. 31 to Sept. 3, 1998, URL: http://www.smallsat.org/proceedings/12/ssc98/11/sscxi6.pdf
3) http://www.ballaerospace.com/page.jsp?page=90
4) W. Randy Bell, Paul G. Weber, “Multispectral Thermal Imager — Overview,” Invited Paper for SPIE?s 15th Aerosense Symposium, Orlando, FL, USA, April 16-20, 2001, URL: http://library.lanl.gov/cgi-bin/getfile?00357140.pdf
5) , R. Rex Kay, N. G. Rackley, “Multispectral Thermal Imager (MTI) Satellite Imaging Operations and Performance,” AIAA/USU Conference on Small Satellites, Aug. 13-16, 2001, Logan, UT, SSC01-V-2
6) J. J. Szymanski, W. Atkins, L. Balick, et al., “Multispectral Thermal Imager Science, Data Product and Ground Data Processing Overview,” IGARSS'2001, Sydney, Australia, July 9-13, 2001
7) Darrick Hurst, “MTI satellite continues to serve after 10 years in orbit.” SNL, April 19, 2010, URL: https://share.sandia.gov/news/resources/news_releases/mti_10yrs/
8) “MTI satellite celebrates 10 years in orbit, continues to serve,” SNL, March 29, 2010, URL: http://www.ornl.gov/info/news/pulse/no308/feature.shtml
9) J. J. Szymanski, P. G. Weber, “Multispectral Thermal Imager: Mission and Applications Overview,” IEEE Transactions on Geoscience and Remote Sensing, Vol. 43, Issue 9, Sept. 2005, pp.1943-1949
10) John German, “Sandia's MTI satellite completes its three-year mission,” June 2003, URL: http://www.sandia.gov/LabNews/LN05-30-03/key05-30-03_stories.html#mti
11) J. D. German, “Around the world 17,300 times: Sandia's MTI satellite completes its three-year orbital research assignment,” May 30, 2003, URL: http://www.sandia.gov/LabNews/LN05-30-03/labnews05-30-03.pdf
12) Information was provided by Frantisek Farnik of the Astronomical Institute, Ondrejov, Czech Republic.
13) http://www.aero.org/capabilities/cords/pdfs/SOPSO-V3-4.pdf
14) S. L. Lawson, A. P. Rodger, B. G. Henderson, S. C. Bender, P. G. Lucey, “Multispectral Thermal Imager Observations of the Moon during Total Eclipse,” 34th Lunar and Planetary Science Conference, March 17-21, 2003, Houston, TX, USA, URL: http://www.lpi.usra.edu/meetings/lpsc2003/pdf/1761.pdf
15) “DOE satellite begins research mission,” June 14, 2000, URL: http://www.sandia.gov/media/NewsRel/NR2000/mti.htm
16) T. Hanson, “MTI satellite begins scientific work, maps Cerro Grande Fire damage,” URL: http://www.lanl.gov/news/index.php/fuseaction/home.story/story_id/1037
17) R. Rex Kay, S. C. Bender, T. D. Henson, D. A. Byrd, J. L. Rienstra, M. L, Decker, N. G. Rackley, P. J. Claassen, R. E. Kidner, R. B. Taplin, D. M. Burlington, K. D. Marbach, C. E. Lanes, C. Little, B. W. Smith, B. C. Brock, P. G. Weber, “Multispectral Thermal Imager (MTI) Payload Overview,” Proceedings of SPIE, Imaging Spectroscopy V, Vol 3753, Denver , CO, July 19-21, 1999, pp. 347-358, URL: http://www.osti.gov/bridge/servlets/purl/8953-ELzDWI/webviewable/8953.pdf
18) T. D. Henson, S. Bender, W. Rappoport, et al., “Multispectral Thermal Imager Optical Performance and Integration of the Flight Focal Plane Assembly,” SPIE Vol. 3753, Denver , CO, July 19-21, 1999, pp. 359-368
19) J. L. Rienstra, M. Ballard, “MTI focal plane assembly design and performance,” 1999, URL: http://www.osti.gov/bridge/servlets/purl/7872-J6eg9x/webviewable/7872.pdf
20) R. R. Kay, B. C. Brock, T. D. Henson, J. L. Rienstra, M. L. Decker, N. G. Rackley, “An Introduction to the Department of Energy's Multispectral Thermal Imager (MTI) Project,” Proceedings of the 12th AIAA/USU Conference on Small Satellites, Logan, UT, USA, Aug. 31 to Sept. 3,1998
21) Paul G. Weber, Christoph C. Borel, William B. Clodius, Bradly J. Cooke, Barham W. Smith: "Design considerations, modeling and analysis for the Multispectral Thermal Imager", Proceedings of. SPIE Conference on 'Infrared Imaging Systems: Design, Analysis, Modeling, and Testing,' SPIE Vol 3701, paper 3701-13, 1999.
22) Tammy Henson, Les Krumel, Dick Blake, Don Byrd, Wynn Christensen, William Rappoport, Gon-Yen Shen, “Multispectral Thermal Imager Optical Assembly Performance and Integration of the Flight Focal Plane Assembly,” URL: http://cborel.net/Henson_SPIE99.pdf
23) P. Nandy, J. L. Smith, M. L. Decker, “Subpixel resolution with the multispectral thermal imager (MTI),” Proceedings of the SPIE, Vol. 5159, `Imaging Spectrometry,' edited by Sylvia S. Shen, and Paul E. Lewis, SPIE Annual Meeting, Aug. 6-7, 2003, pp. 147-157
24) B. J. Tomlinson, D. Davidson, C. Lanes, W. Burt, A. Gilbert, “ Multispectral Thermal Imager (MTI) Space Cryocooler Development, Integration, and Test,” Springer book “Cryocoolers,” DOI: 10.1007/b113939
25) W. B. Clodius, C. Borel, L. Balick, S. J. Hook, “Validation of the MTI Water Surface Temperature Retrieval Algorithms,” Proceedings of IGARSS 2002, Toronto, Canada, June 24-28, 2002
26) W. B. Clodius, et al., “MTI On-Orbit Calibration,” SPIE Vol. 3753, Denver , CO, July 19-21, 1999, pp. 380-391
27) E. Villa-Aleman, R. J. Kurzeja, M. M. Pendergast, “MTI Thermal Bands Calibration at Ivanpah Playa with a Fourier Transform Infrared Spectrometer,” URL: http://sti.srs.gov/fulltext/ms2001550/ms2001550.html
28) B. D. Zak, P. Nandy, J. Smith, “The Multispectral Thermal Imager (MTI) North Slope of Alaska Project,” Fourteenth ARM Science Team Meeting Proceedings, Albuquerque, New Mexico, March 22-26, 2004, URL: http://www.arm.gov/publications/proceedings/conf14/extended_abs/zak-bd.pdf
29) S. J. Hook, W. B. Clodius, L. Balick, R. E. Alley, A. Abtahi, R. C. Richards, S. G. Schladow, “In-Flight Validation of Mid and Thermal Infrared Data from the Multispectral Thermal Imager (MTI) Using an Automated High Altitude Validation Site at Lake Tahoe CA/NV, USA,” IEEE Transactions on Geoscience and Remote Sensing, Vol. 43, No 9, 2005, URL: http://laketahoe.jpl.nasa.gov/publications/2005_Hook_et_al_In-Flight_Val_Mid_Thermal_Infrared
_Data_Multispectral_Thermal_Imgr.pdf
30) P. Chylek, C. C. Borel, W. Clodius, P. A. Pope, A. P. Rodger, “Satellite Based Columnar Water Vapor Retrieval with the Multi-Spectral Thermal Imager (MTI),” IEEE Transactions on Geoscience and Remote Sensing, accepted, 2003
31) R. J. Kurzeja, B. L. O'Steen, M. M. Pendergast, “Determination of the Spatial Variability of Temperature and Moisture Near a Tropical Pacific Island with MTI Satellite Images,” WSRC-MS-2001-00561
32) F. Fárnik, H. Garcia, A. Kiplinger, “Solar Broad-band Hard X-Ray Spectrometer Onboard the MTI Satellite,” Proceedings of `A Crossroads for European Solar & Heliospheric Physics Conference,' Tenerife, March 23-27, 1998, pp. 305-308, ESA SP-417
33) H. A. Garcia, F. Fárnik, A. L. Kiplinger, “Hard x-ray spectroscopy for proton flare prediction,” Proceedings of the SPIE Conference on Missions to the Sun II, San Diego, CA, July 1998, Vol. 3442, pp. 210-216
34) F. FárnÍk, H. Garcia, M. Karlický, “ New Solar Broad-Band Hard X-Ray Spectrometer: First Results,” Solar Physics, Vol. 201, No 2, Juli 2001, pp.357-372
35) F. Fárník, H. S. Hudson, M. Karlický, T. Kosugi, “X-ray and radio observations of the activation stages of an X-class solar flare,” Astronomy & Astrophysics, Vol. 399, 2003, pp. 1159-1166, URL: http://www.aanda.org/index.php?option=article&access=standard&Itemid=129&url=/articles/aa/pdf/2003/09/aa2883.pdf
36) Howard Garcia, Alan Kiplinger, Frantisek Farnik, “Proton Storms from Hard X-ray Spectroscopy,” URL: http://www.swpc.noaa.gov/AboutUs/Review2000/Garcia_Poster.ppt
37) John J. Szymanski,* Christoph C. Borel, William B. Clodius, Anthony B. Davis, Christopher E. Heil, James B. Krone, Keri Ramsey, Barham W. Smith, Kathy Smith, James Theiler, Pierre V. Villeneuve, Paul G. Weber, “ Proceedings of SPIE, 'International Symposium on Optical Science, Engineering, and Instrumentation,' Denver, CO, USA, July 18-23, 1999, URL: http://cborel.net/Szymanski_SPIE99.pdf
38) John J. Szymanski,* William Atkins, Lee Balick, Christoph C. Borel, William B. Clodius, Wynn Christensen, Anthony B. Davis, J. Chris Echohawk, Amy Galbraith, Karen Hirsch, James B. Krone, Cynthia Little, Peter McLachlan, Aaron Morrison, Kim Pollock, Paul Pope, Curtis Novak, Keri Ramsey, Emily Riddle, Charles Rohde, Diane Roussel-Dupre, Barham W. Smith, Kathy Smith, Kim Starkovich, James Theiler, Paul Weber, “Multispectral Thermal Imager Science, Data Product and Ground Data Processing Overview,” Proceedings of IGARSS 2001, Sydney, Australia, July 9-13, 2001
The information compiled and edited in this article was provided by Herbert J. Kramer from his documentation of: ”Observation of the Earth and Its Environment: Survey of Missions and Sensors” (Springer Verlag) as well as many other sources after the publication of the 4th edition in 2002. - Comments and corrections to this article are always welcome for further updates (eoportal@symbios.space).