SIMBA (Sun-earth IMBAlance)
EO
ESA
Atmosphere
Radiation budget
Quick facts
Overview
Mission type | EO |
Agency | ESA, RMI |
Mission status | Operational (nominal) |
Launch date | 03 Sep 2020 |
Measurement domain | Atmosphere |
Measurement category | Radiation budget |
Measurement detailed | Downward long-wave irradiance at Earth surface, Downwelling (Incoming) solar radiation at TOA, Downward short-wave irradiance at Earth surface, Solar Spectral Irradiance |
Instruments | SIMBA Instrument |
Instrument type | Earth radiation budget radiometers |
CEOS EO Handbook | See SIMBA (Sun-earth IMBAlance) summary |
SIMBA (Sun-earth IMBAlance)
Spacecraft Launch Sensor Complement References
Overview
ESA’s SIMBA CubeSat is a tiny mission with a big ambition: to measure one of the fundamental drivers of climate change in a new way. The 3U CubeSat will turn from Earth to space to the Sun and back again, to calculate our planet’s overall energy budget. 1) 2) 3) 4) 5)
SIMBA was developed for ESA by a consortium led by Belgium’s Royal Meteorological Institute (RMI) with the University of Leuven and ISIS (Innovative Solutions in Space) of Delft, the Netherlands.
“This is the kind of scientific instrument we’d otherwise place on a full-size satellite platform,” explains Stijn Nevens, Simba principal investigator at RMI.
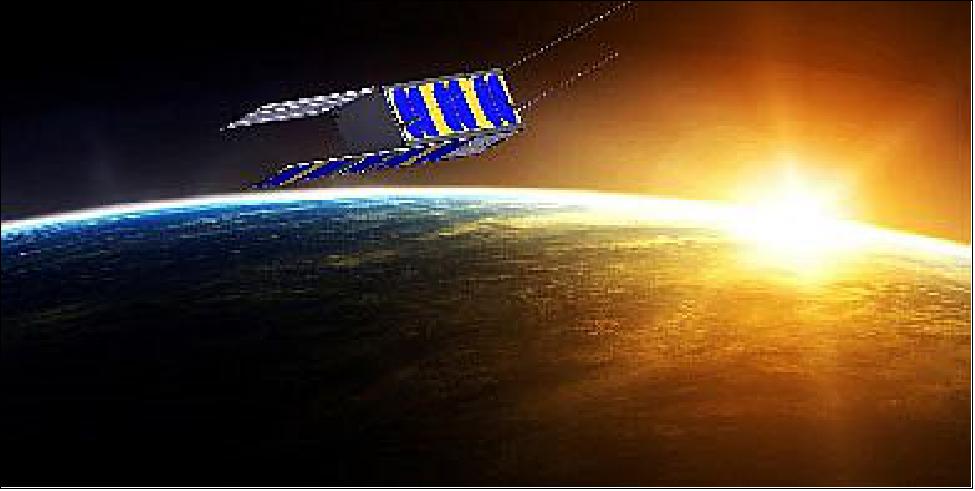
“But if we can make this work on a smaller, cheaper CubeSat, then we might be able to build and fly multiple versions of this instrument in the future, to cover the entire planet for the equivalent cost of a single traditional mission. That is important because the variable we aim to measure is crucial.
“The main origin of climate change is that an increasing amount of heat from the Sun is being retained within the atmospheric system. To quantify that directly we need to measure how much solar energy Earth is receiving – we call this the total solar irradiance – then how much of this is being reflected by the Earth’s surface and atmosphere, or being radiated out as longer-wavelength heat energy.
“Subtracting the second from the first, we end up with a figure for Earth’s radiation budget – the amount of energy our planet holds onto rather than reflects or radiates away.
“We already have a class of instruments to measure irradiated energy, called radiometers, which convert it into electrical power for measuring purposes. Downward-looking radiometers are flying for instance on Europe’s Meteosat satellites in geostationary orbit, as well as the US family of CERES instruments in lower orbits. Then there are Sunward-facing radiometers on satellites like SOHO and PROBA-2.
“But while their results have high relative accuracy, they require a lot of additional modelling to take account of factors such as diurnal differences and surface variations. They accordingly come with a large margin of error, while the instruments themselves possess inherent biases. For sharper climate change modelling we need to do better.”
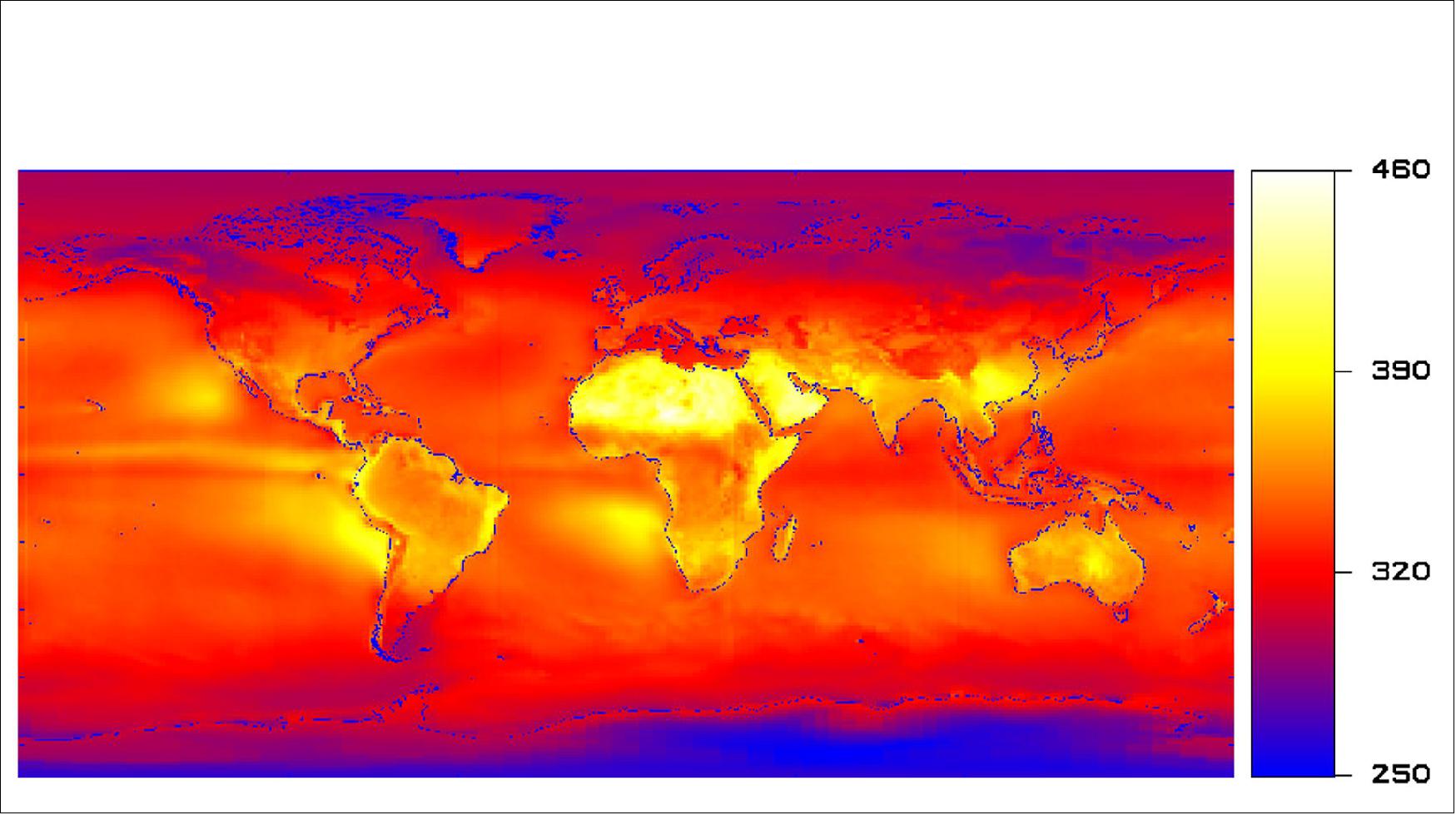
Spacecraft
SIMBA is a 3U CubeSat with dimensions of 30 x 10 x 10 cm and with a mass of 4 kg. The satellite was to be originally part of the QB50 constellation, but eventually dropped out.
The SIMBA nanosatellite consists of 3 main building blocks:
• Satellite generic functions (avionics), specified by RMI, and assembled by ISIS (Innovative Solutions In Space BV, Delft, The Netherlands.
• ADCS (Attitude Determination and Control System) of the Catholic University of Leuven.
• Payload, developed by RMI.
Simba is equipped with a specially-developed CubeSat-optimized ADCS, contributed by the University of Leuven. This includes an experimental star tracker camera to fix its position against the star constellations in the sky and ‘reaction wheels’ whose shifting rate of spin cause the nanosatellite to shift its attitude in reaction.
Dr. Nevens adds: “This ADCS will give Simba a pointing accuracy of 0.1 degrees, which also enhances the overall accuracy of our data. We will achieve traceability, being able to know precisely where and at what we are looking at any one time.”
Simba has been supported by BELSPO (Belgian Science Policy Office) through the ‘Fly’ element of ESA’s GSTP (General Support Technology Program), readying promising technologies for space.
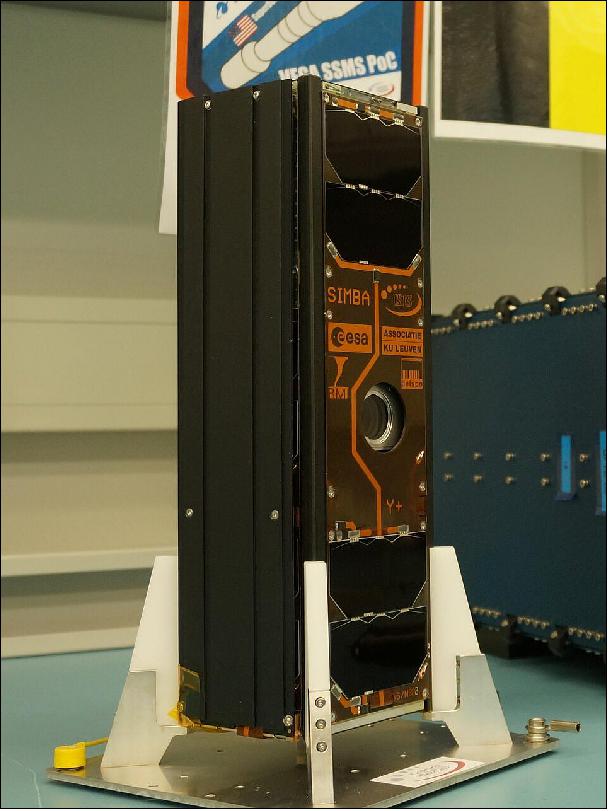
Passenger payloads (53) of the Vega rideshare mission
Arianespace has realized the first European “rideshare” mission for small satellites, with 53 satellites onboard the Vega launcher for 21 customers from 13 different countries. With this new SSMS (Small Spacecraft Mission Service) shared launch concept, Arianespace demonstrates its ability to respond – in an innovative and competitive manner – to institutional and commercial requirements of the growing market for small satellites. The total satellite launch mass was 1,327 kg. 7)
With the demonstration of its new SSMS service, Arianespace is strengthening its position in the growing market for small satellites. This service will soon be supplemented by the MLS (Multi Launch Service) – a similar offer available on Ariane 6, allowing Arianespace to increase the number of affordable launch opportunities for small satellites and constellations.
• ESAIL is a maritime microsatellite with a mass of 112 kg for AIS (Automatic Identification System) ship tracking operated by exactEarth. It was built by a European manufacturing team led by the satellite prime contractor Luxspace. ESAIL features an enhanced multiple antenna-receiver configuration for global detection of AIS messages and high-resolution spectrum capture, which will enable the demonstration of advanced future services such as VDES (VHF Data Exchange System) message reception. 8)
• Lemur-2, eight 3U CubeSats built by Spire Global Inc., San Francisco, CA . These satellites carry two payloads for meteorology and ship traffic tracking. The payloads are: STRATOS GPS radio occultation payload and the SENSE AIS payload.
• TriSat is a 3U CubeSat (5 kg) imaging mission led by the University of Maribor, Slovenia. The mission is focused on remote sensing by incorporating a miniaturized multispectral optical payload as the primary instrument, providing affordable multispectral Earth observation in up to 20 non-overlapping bands in NIR-SWIR (Near to Short Wave Infrared) spectrum.
• The launch integrator company Spaceflight Inc. of Seattle WA is providing its services for four different customers with a total of 28 satellites. These are:
a) NewSat-6 (also written as ÑuSat-6), is a low Earth orbit commercial remote sensing microsatellite (43.5 kg) designed and manufactured by Satellogic S.A. with HQs in Argentina, a vertically integrated geospatial analytics company that is building the first Earth observation platform with the ability to remap the entire planet at both high-frequency and high-resolution. This is Satellogic’s 11th spacecraft in orbit, equipped with multispectral and hyperspectral imaging capabilities and it will be added to the company’s growing satellite constellation.
b) 14 Flock-4v, 3U CubeSats, next-generation SuperDove satellites of Planet Inc., San Francisco, they will join its constellation of 150 Earth-imaging spacecraft.
c) SpaceBEE, 12 (.25U) picosatellites of Swarm Technology which provide affordable global connectivity.
d) Tyvak-0171, an undisclosed minisatellite of Tyvak, developed by Maxar with a mass of 138 kg.
e) Planet Inc. of San Francisco launches a total of 26 Flock 4v SuperDoves on this mission. They will be split into two batches on the same launch: 14 of them will be housed inside and deployed from ISL’s QuadPack deployers and the remaining 12 will be deployed from D-Orbit’s InOrbit Now (ION) freeflying deployment platform. 9)
f) Athena, a communications minisatellite mission (138 kg) of PointView Tech LLC, a subsidiary of Facebook. The objective is to provide broadband access (internet connectivity) to unserved and underserved areas throughout the world.
g) AMICalSat, a 2U CubeSat, an educational mission, developed by CSUG (University of Grenoble Alpes, France) and MSU-SINP (Lomonosov Moscow State University-Skobeltsyn Institute of Nuclear Physics, Russia). The objective is to take pictures of the Northern light in order to reconstruct the particle precipitation into the polar atmosphere. The payload is a very compact, ultra-sensitive wide filed imager (f=23mm, aperture f/1.4). Firstly, AMICal Sat will observe auroras using nadir pointing, i.e. by determining the center of the Earth to map and link the geographical position of the auroral oval and its internal structures with solar activity. Secondly, the CubeSat will perform image capture ‘in limbo’ through tangential orientation with the Earth to capture the vertical profile of the auroras and match an altitude to their various emissions.
h) PICASSO, a 3U CubeSat mission (mass of 3.8 kg) developed for ESA ( European Space Agency) led by BISA (Belgian Institute for Space Aeronomy), in collaboration with VTT Technical Research Center of Finland Ltd, Clyde Space Ltd. (UK) and the CSL (Centre Spatial de Liège), Belgium. The goal is to develop and operate a scientific 3U CubeSat.
i) GHGSat-C1 of GHGSat Inc., Montreal, Canada, is the first of two nanosatellites (~16 kg) as the commercial follow-on to the GHGSat-D (CLAIRE) demonstration satellite developed and launched by UTIAS/SFL of Toronto in 2016. GHGSat monitors industries greenhouse gas (GHG) and air quality gas (AQG) emissions, including: oil & gas, power generation, mining, pulp & paper, pipelines (natural gas), landfill, chemicals, metals & aluminum, cement, agriculture, and transportation.
j) NEMO-HD of SPACE-SI (Slovenian Center of Excellence for Space Sciences and Technologies) is a microsatellite (65 kg) developed at UTIAS/SFL of Toronto, Canada in cooperation with SPACE-SI. The NEMO-HD (Next-generation Earth Monitoring and Observation-High Definition) satellite is a high precision interactive remote sensing mission for acquiring multispectral images and real time HD video.
k) FSSCat (Federated Satellite Systems on Cat) is the winner of the 2017 Copernicus Master “ESA Sentinel Small Satellite Challenge (S3)”. Proposed by the Universitat Politèctica de Catalunya (UPC) and developed by a consortium composed of UPC (ES), Deimos Engenharia (PT), Golbriak Space (EE), COSINE (NL) and Tyvak International (IT).
l) Phi-Sat-1 (Φ-Sat-1) is the first on-board ESA initiative (6U CubeSat) on Artificial Intelligence (AI) promoted by the Φ Department of the Earth Observation Directorate and implemented as an enhancement of the FSSCat mission. Among mission objectives, scientific goals are Polar Ice and Snow monitoring, soil moisture monitoring, terrain classification and terrain change detection (i.e. hazard detection and monitoring, water quality), while technological goals are optical Inter-Satellite Link (OISL) demonstration.
m) The RTAFSAT-1 (Royal Thai Air Force Satellite-1) mission, also referred to as NAPA-1, is a 6U CubeSat, the first remote sensing CubeSat mission for Thailand. The satellite will carry out an Earth Observation Demonstration mission with SCS Gecko Camera and Simera TriScape-100 payloads; the designed lifetime is 3 years.
n) DIDO-3, a commercial 3U CubeSat mission of SpacePharma. The objective is to gather data by researching the effects of a microgravity environment on biological materials. SpacePharma from Israel will be on board of SSMS POC with DIDO-3 Nanosatellite to perform biological experiments under Microgravity for several customers involved in pharmaceutical business, supported by the Italian Space Agency (ASI) and Israeli Space Agency (ISA). Dido-3 will be monitored from the Ground Station developed by SpacePharma in Switzerland.
o) SIMBA (Sun-Earth Imbalance), a 3U CubeSat mission led by the Royal Meteorological Institute Belgium, The objective is to measure the TSI (Total Solar Irradiance) and Earth Radiation Budget climate variables with a miniaturized radiometer instrument. This mission will help in the study of global warming. This science mission will have a design lifetime of 3 years and the satellite performances will be monitored from a ground station located in The Netherlands.
p) TARS-1, a 6U CubeSat of Kepler Communications, developed at ÅAC Clyde Space for IoT (Internet of Things) applications. TARS-1 features deployable solar arrays, software defined radios (SDR), a narrowband communications payload and high gain antennas.
q) OSM-1 Cicero, the first nanosatellite developed in Monaco by OSM (Orbital Solutions Monaco engineers, a 6U CubeSat with a mass of ~10 kg) based on the Tyvak Nano-Satellite Systems design. OSM plans to build nanosatellites to gather environment and climate data.
r) TTU100, a 1U CubeSat developed at the Tallin University of Technology, Estonia. The objective is to test earth observation cameras and high-speed X-band communications. It will perform remote sensing in the visible and IR electromagnetic spectrum.
• UPMSat-2 (Universidad Politecnica de Madrid Satellite-2), a demonstration microsatellite (45 kg) of IRD-UPM.
Launch
The SIMBA 3U CubeSat was launched as a passenger payload at 01:51 UTC, 03:51 CEST on 3 September, 2020 (22:51 local time on 2 September in Kourou).The launch vehicle was the VEGA SSMS (Small Spacecraft Mission Service) Proof Of Concept flight. 6)
Orbit
Sun-synchronous orbit; target orbit for the 7 microsatellites: altitude of 515 km, inclination of 97.45º; target orbit for the 46 nanosatellites: altitude of 530 km, inclination = 97.51º. The nominal mission duration (from liftoff to separation of the 53 satellites) is: 1 hour, 44 minutes and 56 seconds.
Sensor Complement
Radiometer
The idea with SIMBA is to achieve a high absolute accuracy by employing the same instrument for the very first time to measure irradiance from both the Sun and Earth. The CubeSat will turn from our planet to deep space – for calibration purposes – then to our parent star.
“We’re using a broadband, wild field of view instrument, meaning we’re measuring the total outgoing flux from the whole Earth,” adds Dr. Nevens. “Simba is based on a cavity radiometer, which is basically an internal space on the other side of a very small hole, totally painted black. We are measuring how that cavity warms up.
“Imagine a house with central heating that you want to keep warm. On a summer day you don’t have to do any heating, but on a winter’s day you’ll lose a lot of heat and need to actively warm it. So we’ll be measuring how much extra energy we need to put in to maintain a fixed temperature.
“To get our baseline we’ll begin the mission by looking down at Earth for a long time, to see what temperature it stabilizes at. Then we’ll swivel out to deep space, just a few degrees from absolute zero, to learn the maximum level of heat we need to apply to keep it there. Then we will turn to the Sun in turn, measuring the amount of radiation coming in.”
SIMBA Measurement Concept
The SIMBA Sun-Earth measurement concept is illustrated in Figure 4. In order to be able to measure the small imbalance which is believed to be driving climate change – of the order of 0.5 W/m2 – the difference between the incoming solar radiation – of the order of 340.5 W/m2 – and the outgoing terrestrial radiation – of the order of 340 W/m2 has to be measured. When the incoming and outgoing radiation are measured by separate instruments – as is the traditional approach – the small imbalance to be measured will be overwhelmed by the sum of the calibration errors of the separate sun and earth measurements. The new approach proposed by Simba is to measure with the same instrument the Sun and the Earth radiation, such that in principle the instrument calibration error is the same for the Sun and for the Earth measurement and cancels in the differential Sun-Earth measurement.
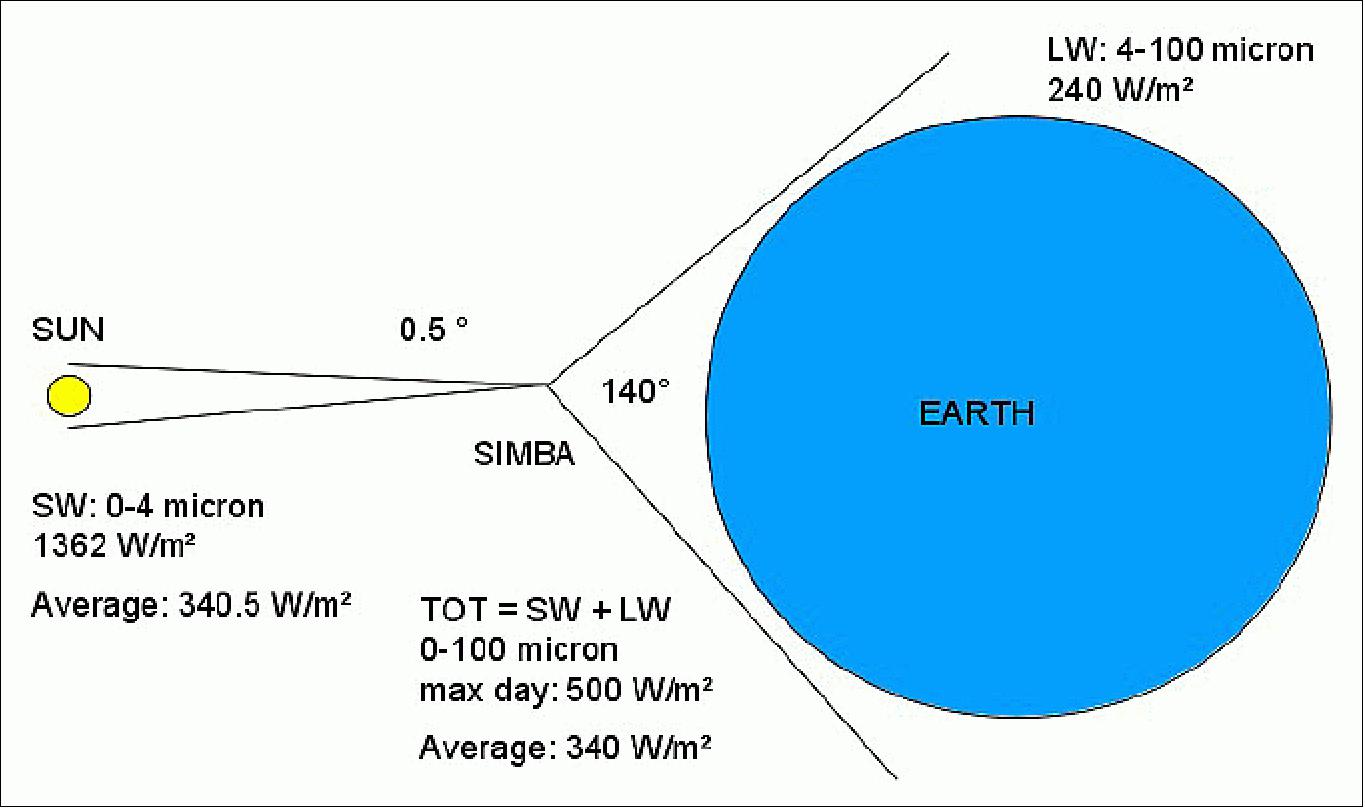
Even with this differential sun-earth measurement concept, several challenges remain in order to have an accurate intercalibration of the sun-earth measurements:
1) The Sun is almost a point source covering an angle of 0.5°, compared to the earth which covers a large field of view of 140° when viewed from horizon to horizon from a low earth orbit. Therefore Simba will need a wide field of view with a uniform angular sensitivity. As main SIMBA sensor we choose a wide field of view cavity radiometer, as has been used on the Nimbus-6, and -7 and ERBE satellites.
2) The Sun's surface is approximately a blackbody of 5800 K, emitting SW (ShortWave) radiation with wavelengths up to 4 µm. The Earth is approximately a blackbody of 300 K, emitting LW (LongWave) radiation above 4 µm. At night the Earth leaving radiation is only LW radiation, during the day it is composed of both SW reflected solar radiation and LW emitted thermal radiation. The SIMBA cavity needs to have a flat spectral response. As auxiliary sensors for the separation of Earth's SW and LW radiation, black and white flat spectral sensors are foreseen. The black sensor will absorb SW and LW radiation, the white sensor will mainly absorb LW radiation.
3) The Earth-leaving radiation is highly variable in space and in time due to the daily weather variation. Therefore the SIMBA MSF (Main Sensor Face), containing the cavity radiometer, will be pointed nearly continuously towards Earth, in order to maximize the Earth sampling. Only sporadically, e.g. once every three months, the MSF will be pointed towards the sun for a solar calibration. As the solar variation is well known (Figure 5), such a sporadic sampling of the sun is in principle sufficient. The SIMBA pointing modes are illustrated in Figure 6. During nadir pointing, the MSF is pointed towards Earth. During zenith pointing, the satellite is flipped over by 180°, and the MSF is seeing deep space for calibration at night, and sees the sun sweeping trough its field of view during the day, allowing a basic solar measurement. In a dedicated solar pointing mode, the angle of the MSF relative to the sun is kept fixed, for an improved solar calibration. As auxiliary sensors, the project foresees a second set of black and white sensors on the face opposite the MSF, and a wide angle visible camera on the MSF. The former allows solar and deep space calibration measurements during nadir pointing, the latter allows a high resolution characterization of the reflected solar radiation.
![Figure 5: RMIB TSI composite measurements (red: daily measurements, green: 121 day running mean) and regression model (blue: 121 day running mean model) based on Mount Wilson magnetograms [image credit: RMIB (Royal Meteorological Institute Belgium)]](/api/cms/documents/163813/5862427/SIMBA_Auto1.jpeg)
4) The diurnal cycle of the radiation, which is particularly strong in the tropics, is only sampled twice per day from LEO (Low Earth Orbit). The full sampling of the diurnal cycle combined with global measurements requires sampling with a constellation of satellites. While such a constellation would be expensive and slow to put in place with conventional satellite programs – e.g. the NASA ERBE program - it becomes feasible thanks to the relatively low cost and fast CubeSat approach. To measure the Earth radiation imbalance with a constellation of satellites is the long-term goal of the freshly started RMIB SIMBA program, to be compared with the measurement of the Solar Constant that was achieved in a 30 year program starting with the first RMIB space flight in 1983. The first SIMBA satellite to fly with QB50 in 2016 is intended as the technology demonstrator satellite of the SIMBA program.
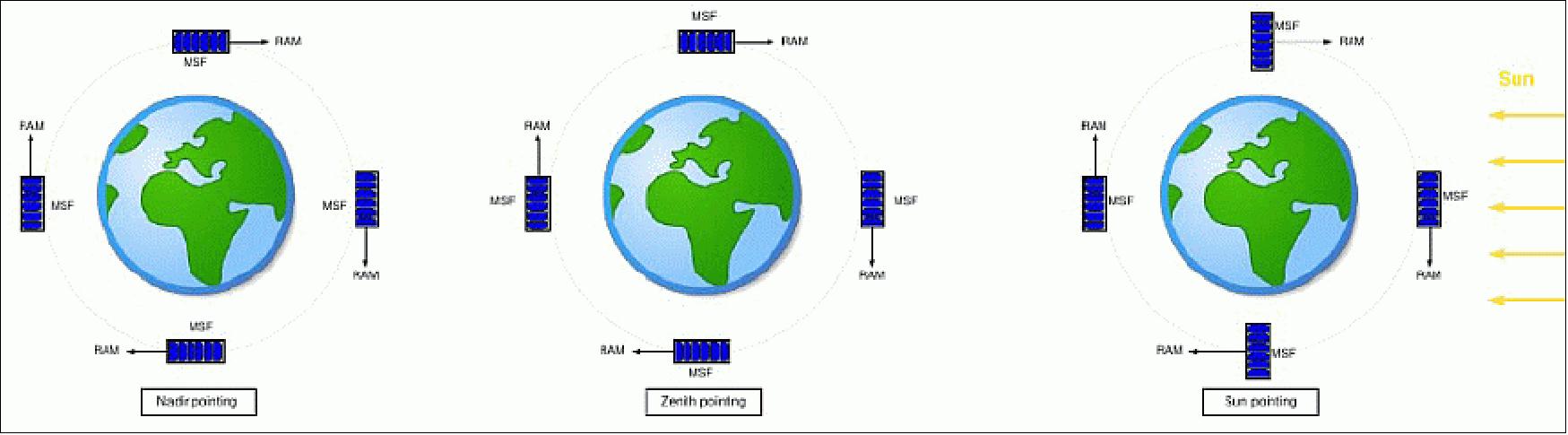
References
1) ”Simba CubeSat to swivel from Earth to Sun to help track climate change,” ESA Enabling & Support, 15 June 2020, URL: http://www.esa.int/Enabling_Support/Space_Engineering_Technology
/Simba_CubeSat_to_swivel_from_Earth_to_Sun_to_help_track_climate_change
2) Tjorven Delabie, ”SIMBA Sun Earth Imbalance mission,” KU Leuven, November 2015, URL: http://be-space.eu/wp-content/uploads/2015/11/SIMBA_Delabie.pdf
3) S. Dewitte, A. Chevalier, E. Janssen, N. Clerbaux, M. Meftah, A. Irbah,T. Delabie, O. Karatekin, “The Sun‐Earth Imbalance Radiometer for a Direct Measurement of the Net Heating of the Earth,” Proceedings of the 4S (Small Satellites Systems and Services) Symposium, Port Petro, Majorca Island, Spain, May 26-30, 2014
4) Steven Dewitte, “Long term solar changes and their effect on Earth,” STCE (Solar-Terrestrial Center of Excellence) Workshop, Brussels, Belgium, May 19, 2014
5) Steven Dewitte, “Radiation Measurements at the RMIB - Past, Present and Future,” September 27, 2013, URL: http://centenary.meteo.be/meteo/view/en/11939750-Dewitte.html
6) ”Vega return to flight proves new rideshare service,” ESA Enabling & Support, 3 September 2020, URL: https://www.esa.int/Enabling_Support/Space_Transportation
/Vega/Vega_return_to_flight_proves_new_rideshare_service
7) ”With Vega, Arianespace successfully performs the first European mission to launch multiple small satellites,” Arianespace Press Release, 3 September 2020, URL: https://www.arianespace.com
/press-release/with-vega-arianespace-successfully-performs-the-
first-european-mission-to-launch-multiple-small-satellites/
8) ”Vega flight VV16,” Wikipedia, URL: https://en.wikipedia.org/wiki/Vega_flight_VV16
9) Mike Safyan, ”Planet’s First Launch of 2020: 26 SuperDoves on a Vega,” Planet, 13 February 2020, URL: https://www.planet.com/pulse/planets-first-launch-of-2020-26-superdoves-on-a-vega/
The information compiled and edited in this article was provided by Herbert J. Kramer from his documentation of: ”Observation of the Earth and Its Environment: Survey of Missions and Sensors” (Springer Verlag) as well as many other sources after the publication of the 4th edition in 2002. - Comments and corrections to this article are always welcome for further updates (eoportal@symbios.space).