TacSat-2 (JWS-D1 (Joint Warfighting Space-Demonstrator 1) or RoadRunner)
EO
Ocean
Mission complete
High resolution optical imagers
Quick facts
Overview
Mission type | EO |
Agency | DoD (USA) |
Mission status | Mission complete |
Launch date | 16 Dec 2006 |
End of life date | 05 Feb 2011 |
Measurement domain | Ocean, Land |
Measurement category | Landscape topography, Ocean topography/currents |
Measurement detailed | Land surface topography, Ocean dynamic topography, Sea-ice sheet topography |
Instruments | AIS, GPS receiver |
Instrument type | High resolution optical imagers, Data collection |
CEOS EO Handbook | See TacSat-2 (JWS-D1 (Joint Warfighting Space-Demonstrator 1) or RoadRunner) summary |
TacSat-2 (also known as JWS-D1 (Joint Warfighting Space-Demonstrator 1) or RoadRunner)
TacSat-2 (Tactical Satellite-2) is a DoD technology demonstration mission of AFRL (Air Force Research Laboratory) within AFRL's (Kirtland AFB, Albuquerque, NM) Roadrunner program. Initially, the OFT (Office of Force Transformation) was the author and prime mover of the ORS (Operationally Responsive Space) initiative and the sponsor of the TacSat series; in May 2007, the program management was transferred from OFT to the newly created ORS Program Office, located at Kirtland AFB. In this setup, AFRL is the program manager for TacSat-2 and -3 while NRL (Naval Research Laboratory) is the program manager for TacSat-1 and - 4.
The TacSat-2 objective is to demonstrate techniques and methodologies to dramatically shorten the development time required for small satellites. TacSat-2 represents in fact a new way of doing business in spacecraft implementation. Specific mission objectives are: 1) 2) 3) 4) 5) 6)
• To develop a spacecraft from concept baseline to launch readiness in under 14 months
• To deploy a spacecraft from storage to full on-orbit functionality in 7 days, including autonomous on-orbit commissioning within a day
• To demonstrate in-field tactical communication, command and control of the S/C
• Image targets in the visible spectrum at a “tactically significant” resolution of < 1 m GSD
• To geolocate RF (Radio Frequency) targets and image them in the same pass
• To demonstrate several additional advanced technology experiments.
Plans are to provide the Joint Task Force (JTF) Commander with a direct operational capability by 2010.

Spacecraft
The spacecraft bus was developed by MSI (MicroSat System's Inc.) of Littleton, CO, using the spare bus and parts of the TechSat-21 program (which was cancelled in early 2003) with minor modifications. The bus structure consists of composite facesheets over an aluminum honeycomb core, comprising only 13% of the spacecraft mass. The basic structure is an irregular octagon with a “payload” and a “separation” deck.
Note: MSI is a wholly-owned subsidiary of SNC (Sierra Nevada Corporation).
The platform is 3-axis stabilized capable of providing a pointing accuracy of ≤ 0.15º. The ADCS (Attitude Determination and Control Subsystem) employs 3 reaction wheels (Dynacon MW-1000) and 3 torque rods as actuators. A star camera, referred to as ISC (Inertial Stellar Compass), provides high-precision attitude measurements. An IMU (Inertial Measurement Unit), analog sun sensors, and a magnetometer complete the ADCS sensor suite. 7) 8)
Electric power of up to 550 W is provided by the solar array using thin-film technology [referred to as FITS (Fold Integrated Thin-film Stiffener)], a low mass, low stowage volume solution for small satellite missions. The Li-ion battery has a capacity of 30 Ah. The bus power system is based on a direct energy transfer topology (tied directly to the battery via a set of FETs) delivering unregulated 28 V switched power to the loads. The C&DH (Command and Data Handling) subsystem employs a Power PC. Bus communication is provided by a Mini-SGLS (Miniature Space-to-Ground Link Subsystem), using 2 pairs of patch antennas for full spherical coverage and an encryption device. The spacecraft has a mass of about 370 kg. The mission life is 1 year (goal).
Note: ISC was funded by NASA and developed by CSDL (Charles Stark Draper Laboratory Inc.) of Cambridge, MA. The ISC attitude system employs a STAR¿250 APS imager of FillFactory NV with a pixel pitch of 25 µm. The ISC has the following key performance features: 0.1º (1σ) pointing accuracy in each axis; high¿rate maneuver capability; self¿initializing capability; mass of 2.9 kg; power of 3.5 W. ISC is composed of a wide FOV APS camera (21º FOV) and a MEMS gyro assembly with associated processing and power electronics. 9) 10)
Since 2004, ISC was planned to fly on several missions from a HitchHiker payload on the Space Shuttle to a commercial solar sail mission (Team Encounter Flight One), as well as on the ST-6 (Space Technology-6) mission (all were somehow cancelled). The experiment finally found its way onto the TacSat-2 spacecraft for space qualification. 11)



Orbit
Near-circular orbit, altitude = 413 km x 424 km, inclination = 40º, period =92.9 min.
A patented and enhanced OCK (Orbit Control Kit) of Microcosm was used onboard TacSat-2., an autonomous stationkeeping software package, which negates the influence of unwanted orbital perturbations. The OCK uses GPS data to compute the current orbit and generate ΔV requests required for precise stationkeeping. A ΔV request consists of the following information: time of the burn maneuver, magnitude of ΔV, and a flag indicating an in-track burn or a cross-track burn. OCK must be tuned to a specific orbit, based on altitude, eccentricity, and argument of perigee. 12)
RF Communications
CDL (Common Data Link) technology is being implemented (X-band downlink and uplink) using the CCSDS protocols. AFRL is providing the first year of on-orbit operations.
The most important contribution of TacSat-2 is the use of a space-based CDL. Flying a CDL transponder requires ground-breaking work in frequency allocations and communications security (COMSEC) approaches. Direct tasking and data dissemination are being implemented using the SIPRNet (Secret Internet Protocol Routing Network) capabilities, a classified network of DoD. The SIPRNet system objectives are to integrate all space assets into the forces so that the Joint Task Force (JTF) Commander can call up assets by deciding the payload capability required, i.e., the area of interest, the area for direct downlink, and the date the assets must be operational. 13)
Spacecraft launch mass, bus dry mass | 367 kg, 153 kg |
Spacecraft stowed dimension | 2.0 m x 1.1 m x 1.0 m |
Mission design life | 1 year with a goal of 5 years |
Spacecraft stabilization | Three axis stabilized, zero momentum biased |
S/C pointing | 0.10º (control), 0.05º (knowledge) |
S/C slew rate | 0.34-0.64º/s (depending on payload configuration) |
Spacecraft power | 452 W (average), 700 W (peak), fixed array, triple junction GaAs |
Battery | Li-ion, 30 Ah capacity |
C&DH (Command and Data Handling) | 165 MIPS, 640 MB of RAM, 16 MB of NVRAM |
RF communications (TT&C) | 32 kbit/s (uplink) in L-band, 5 Mbit/s (downlink) in S-band |
Propulsion | 154 m/s (ΔV), use of a HET (Hall Effect Thruster) |
Launch
A launch of TacSat-2 took place on Dec. 16, 2006 on a Minotaur-1 vehicle of OSC (Orbital Sciences Corporation) from the commercial MARS (Mid-Atlantic Regional Spaceport) facility at Wallops Island, VA (representing the inaugural flight from MARS). The GeneSat-1 CubeSat (3 kg mass, triple cube) of NASA/ARC and various universities is a secondary payload on this flight. 14)
Note: Initially, TacSat-2 was planned to be launched by a Falcon-1 vehicle of Space-X (Space Exploration Technologies) from VAFB, CA - into a sun-synchronous orbit. However, the long-delayed maiden flight of Falcon-1 experienced a launch failure on March 24, 2006 [the payload on this flight was FalconSat-2 a microsatellite of the USAFA (United States Air Force Academy)]. This event caused AFRL to award a new launch contract for TacSat-2 (and also for TacSat-3) to OSC in May 2006.
The launch of TacSat-2 represents the first responsive space demonstrator operated by the US military, and the initial demonstration of a deployable thin-film solar array in space. The TacSat-2 launch was also the first Minotaur vehicle to fly a RocketCam onboard video camera, which recorded the spectacular early morning liftoff and ascent.
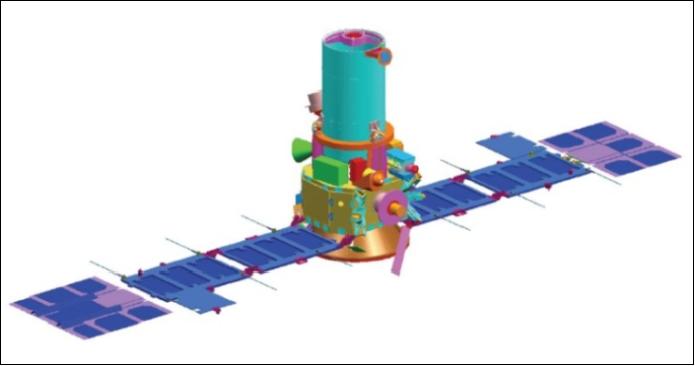
Technology Demonstration
The Minotaur launch vehicle of OSC introduced for the first time an innovative, lightweight space cargo accommodation technology, comprised of carbon fibers and epoxy forming beams and an outer skin structure, known as “Advanced Grid-Stiffened Composite Payload Fairing.” The AGS Composite Payload Fairing serves as a more efficient way to use the strongest materials to work with. Because of the structure's lightness, more mass can be devoted to the payload.
The BMDO/AFRL funded project, which started in 1994, was jointly developed by an AFRL/Boeing team at the Boeing Phantom Works. The nearly autonomous manufacturing method utilizes a combination of advanced fiber placement technology and innovative tooling to allow large composite structures to be fabricated at a lower cost than previously possible. This technique also provides a great deal of flexibility in structural design, allowing last-minute structural modifications to be made during fabrication. Extensive qualification tests were conducted prior to space flight demonstration. 15) 16)
Space operations via internet: TacSat-2 provided an extensive use of web-based tools for operations support in the following areas: 17)
1) The primary telemetry display system, RIMS (Remote Intelligent Monitoring System), was designed by ICS (Interface Control Systems) to be web-accessible. A central server decommutates the incoming telemetry and distributes it to any number of clients which can be either connected on the LAN within MOC (Mission Operations Center), or via a secure website. This system was successfully employed during the I&T (Integration and Test) phase. The system went “live” over the web interface about a month prior to launch in time for the final operation rehearsals. All the telemetry screen in the MOC are viewable via the web interface from anywhere in the world.
2) In a similar capacity, the ICS command generation tools and pass planner are also available on a separate branch of the TacSat-2 operations web page. These allow the user to generate pass plans for future contacts and populate them with the exact commands they want, including pull down menus to select any valid parameters. - Execution of these pass plans is accomplished through the mission controller's command screen. Commanding can also be accomplished via remote connection with appropriate security measures in place.
3) The third web-based feature of the mission is the ability to access and trend historical telemetry data using a variety of tools. RIMS includes a trending tool that pools from one version to the telemetry base.
4) Finally, MSI (MicroSat System's Inc.) also created an e-mail/text paging service that constantly monitors the command history telemetry to look for either indications of trouble or events of specific interest to the user. When a certain key word or text string is detected, an alert is sent via e-mail and text message to the user's phone. This allows engineers and scientists to respond to emergencies quickly.
Mission Status
• TacSat-2 (international designation of 2006 058A) reentered Earth's atmosphere on Feb. 5, 2011. 18)
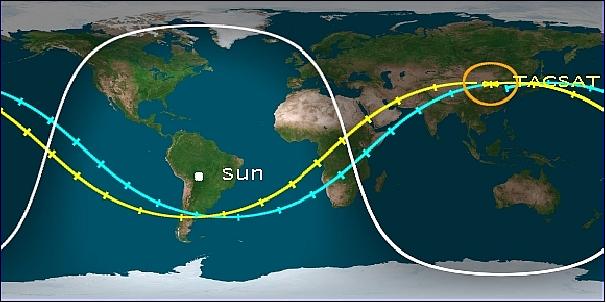
• After completing its goal of a one year experimental mission, the TacSat-2 microsatellite ceased operations on Dec. 21, 2007. The TacSat-2 milestones included: 19) 20)
- Accomplishing web-based, time critical payload tasking and information distribution from in-theater systems employed for aircraft and unmanned aerial vehicle communications. Ground operators were able of successfully tasking and receiving processed data within 90 minutes.
- Collecting tactically relevant imagery and signals intelligence data
- Performing real-time signal geo-location and identification of emitters using satellite and aircraft-based collection platforms
- Supporting 10 science and technology payloads
- The high interest of the USCG (US Cost Guard), the US Navy, and other organizations in the TIE AIS payload warranted additional experimentation. These conditions resulted in the approval of an EUE (Extended User Evaluation). One of the objectives of this EUE was to assess the potential utility of AIS capabilities in coordination with the Maritime Domain Awareness (MDA) community. The EUE ended on 27 November, 2007 when contact with the spacecraft was lost, and the operations team was unable to recover the vehicle. Not all of the EUE objectives were accomplished at least in part due to the unexpected loss of the spacecraft.
The AIS (Automated Identification System) hardware, designed to capture position information from ocean-going ships, demonstrated that AIS signals could be successfully collected from space over extensive areas of open water. The project also provided insights into how to design AIS hardware and software to handle dense signal environments associated with crowded sea lanes, a topic of keen interest to the U.S. Coast Guard participants in the experiment. TacSat-2 was in fact the first small satellite to successfully collect AIS signals from space.
• The testing of the OCK (Orbit Control Kit)did not start before the summer of 2007. A series of three short validation tests, lasting up to several days, were performed. These short duration tests were followed by a 4th extended test that lasted two weeks. Results: Autonomous onboard orbit control has been further validated on TacSat-2 (2007) after its initial validation on UoSat-12 (1999). Similar to the experiment on UoSat-12, OCK managed to maintain very accurate in-track position over an extended period of time on TacSat-2, in spite of a variety of off-nominal events. - The greatest return on investment when using OCK will be on constellations, where constellation maintenance is a significant cost and performance component, and on small, low cost missions that need at least some orbit control. In summary, autonomous, on-board orbit control can fundamentally change the way space missions operate. It is a key component in increasing spacecraft utility and in decreasing operational complexity (Ref. 12).
• The TacSat-2 minisatellite was operating nominally as of 2007 with all subsystems checked out (see reference 40). As of the summer 2007, TacSat-2's imagery has been assessed to be “useful to the tactical operator,” but continues to get better as the calibration process works to obtain the highest National Image Interpretability Rating Scale (NIIRS) rating possible. The primary remaining challenges are:
5) Improve interaction with flight software and the ADCS to reliably take images of the intended target
6) Use the focus mechanism to achieve an optimal focus setting to maximize resolution
7) Reduce noise in the focal plane electronics or categorize it sufficiently to filter it out with automated ground processing
8) Compile a library of three-color images for assessment by an imagery analyst.
• As of late April 2007, the USAF has started to gather imagery from TacSat-2 despite the conflicts as to who is in charge. 21)
• As of March 2007, a policy dispute has held up activation of the spacecraft's main instruments. A policy problem in the form of concerns that the spacecraft could be perceived as an instrument for spying, possibly on US citizens. Due of these concerns, the Office of the Secretary of Defense has withheld its approval for activation of TacSat-2's imaging and signals-intelligence payloads, i.e. the CDL (Common Data Link) downlink. CDL was designed for air-to-ground communications. The system has never been used before for space-to-ground links. 22)
• Immediately after launch, a ground system problem left controllers unable to contact the satellite for several days (this involved an incompatible radio configuration with the ground station). Another setback that has been corrected, was an error in the attitude determination and control system (controllers noticed at times a pointing error of about 2º).
Sensor Complement
ECI (Enhanced Commercial Imager)
ECI is the prime instrument of TacSat-2 (a pushbroom imager), it was developed by AFRL, Nova Sensors, Solvang, CA, and SAIC (Science Applications International Corporation), San Diego, CA. The objective is to provide high-resolution imagery at < 1 m GSD (Ground Sample Distance). The instrument features a Pan band and 3 multispectral bands [red (0.95 µm), green (0.75 µm), and blue (0.55 µm)] in the visible range across a swath of 6144 pixels (5 km swath width).

The optics subsystem employs a COTS telescope of 50 cm aperture of RC Optical Systems. The imager uses a Fairchild Imaging CCD 583 TDI line scan array, sampling at approximately 9600 lines/s. The TDI (Time Delay Integration) process is essentially noise-free, it allows charge accumulation to take place over the number of TDI stages, and preserves the ground resolution capability of the very high rates that the TDI lines are “scanned” off the array. 23)
ROPE (Roadrunner On-orbit Processing Experiment)
ROPE was developed by PSI (Physical Sciences Inc.). This payload consists of an array of FPGAs (Field Programmable Gate Array) designed to process onboard imagery of ECI into standard military imaging formats. ROPE supports also event processing to identify likely targets, and JPEG data compression for real-time data transmission to the ground segment. 24)
ROPE is a reconfigurable on-board, real-time multispectral image processing system with a throughput of 360 MB/s. It consists of of 4 major components: a wide-field multispectral imager, with red, green, blue, and panchromatic bands; the MSP (Malleable Signal Processor), the FP (Fusion Processor), and an 8 GB solid state buffer-a large array of SDRAMs. The MSP is built around five radiation-tolerant Xilinx Virtex-II FPGAs. The MSP has 4 processing pipelines, one for each imagery band. A Xilinx MicroBlaze 32-bit embedded RISC processor in Service FPGA acts as the master MSP controller.
The first processing step is a multiplicative gain correction and a subtractive offset correction to compensate for non-uniformities in the focal plane array. The MSP then performs lossy JPEG image compression. The compressed data, as well as pixels flagged by the RX algorithm, are transferred to the FP via a 64-bit parallel interface. Alternatively, data can be packetized and serialized and directly downlinked to the CDL (Common Data Link) RF modem. Raw data can be buffered in SDRAM on the MSP and transferred to the FP-buffering is necessary because the clock provided to the MSP from the FP across the 64-bit interface is not fast enough to keep up with the MSP raw data flow.

TIE/AIS (Target Indicator Experiment/Automated Identification System)
TIE was provided by NRL/NCST (Naval Center for Space Technology). The Linux-powered TIE payload consists of a wideband sensor suite capable of gathering a wide variety of RF signals (radar, radio and handheld communication signals). The objective is to locate targets based on RF signatures and in conjunction with the P3 aircraft. TIE is an improved version of the implementation on the TacSat-1 satellite. TIE uses a total of 11 antennas.
The TIE payload performs real-time signal geolocation and SEI (Specific Emitter Identification) services of radio frequency (RF) signals using space- and air-based collection platforms. The RF signals are in the range from 0.5 to 18.0 GHz using an adaptation of an UAV (Unmanned Air Vehicle) heritage payload design. Real time commanding of the payload and data down-linking can be performed using an UHF software-defined radio transponder system in concert with a similar radio on the ground that is connected to a modem and laptop computer. The software-defined radio is capable of collecting the AIS (Automated Identification System) signal now required on large ships for port safety and homeland defense.
The TIE payload is also reprogrammable on orbit for acquiring new targets. The TIE package features a commercial telescope of 50 cm aperture to take high-resolution imagery in the same pass.
In essence, this Linux implementation (developed by the PTR Group Inc., Herndon, VA) is the successor to the Copperfield system for TacSat-1. The main differences being that there are now two CPU cards instead of one, and the support for AIS and the SEI capability has been merged into a single cPCI (Compact Peripheral Component Interface) card. In fact, one CPU card consists of a Motorola PowerPC MPC8265, a Xilinx Virtex-II FPGA, and an RF frontend daughter card. 25) 26) 27) 28) 29)
The TIE payload is made up of several main components. A simplified block diagram of the TIE payload is shown in Figure 9.
- TCU (TIE Controller Unit)
- PIU (Payload Interface Unit)
- PPU (Payload Power Unit)
- UHF transponder
- Rubidium clock
- GPS receiver
- RFFE (RF Front End)
- Antenna suite.
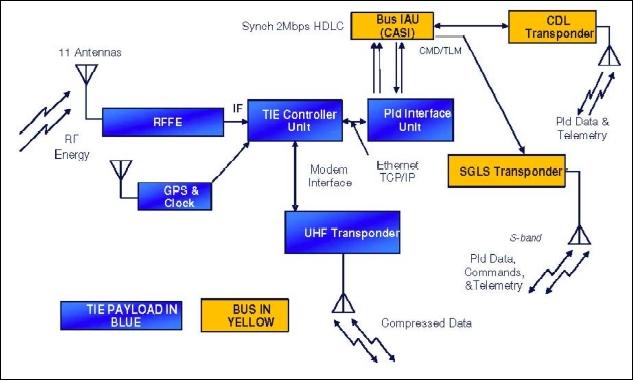

The Target Indicator Experiment provided an interesting opportunity to test various concepts in the area of ORS (Operational Responsive Space) not only from the hardware and software development perspective, but also from the perspective of test and integration.
On-orbit operations have not only validated the use of the TIE hardware, but have also validated the use of the innovative payload control software mechanisms. Built in an unusual way through the use of an operating system scripting language (BASH), the Payload Control (PLC) software leverages the existing developments of a scripting language, and using standard UNIX-style command-line tools to isolate the specific components that need to interface to hardware a lower level. This methodology has proven useful from a spaceflight flexibility standpoint, but also as means to leverage software developments through the development process, to include the integration and testing process.
TIE has proved that with careful engineering and experience in space systems, innovation and radical thinking can result in capable design and fairly smooth integration with high-reuse of components and tools across the life-cycle of a payload.
AIS (Automated Identification System) System Design
The TIE/AIS receiver system was implemented as a software reconfigurable radio to optimize its performance and flexibility. New radio software could be uploaded to the spacecraft to change and improve its performance. Unfortunately, the TIE team did not have the resources to engage in a significant level of reprogramming and upgrading prior to the end of the mission. The modifications that were completed were limited to capturing raw, undemodulated, sampled RF data. These changes, and the sequence of experiments being conducted at the end of the mission were leading to some possible improvements in the software radio algorithm. 30)
The AIS antennas were deployable whips that used a legacy tapered hinge-lock mechanism, and were mounted on and restrained by the solar array (Figure 11). These antenna elements were electrically combined into a directional phased array. Creating a phased array with significant gain or directivity at VHF frequencies requires significant physical separation of the elements. Taking advantage of the size of the TacSat-2 solar arrays facilitated operating at the VHF marine frequencies, and allowed the beam forming electronics to create a beam with some amount of directivity and about 10dB of gain.
The phased array, as implemented, represented a significant compromise from the original design due to mechanical interferences between some of the elements of the array and the spacecraft bus. Two elements were completely removed and one was shortened to a “T” configuration. - Detailed on-orbit antenna pattern measurements were not taken, and would have been challenging at these frequencies with the resources available to the TIE team. However, plotting the AIS data that was collected demonstrated that the antenna pattern was generally consistent with the pattern predicted by the analysis software.
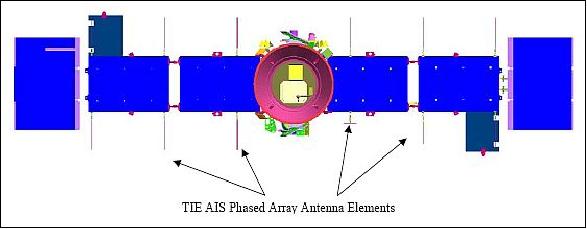
The AIS receiver system was designed to receive and digitize VHF RF signals, and to demodulate the messages on-board the spacecraft. The resulting binary messages were then encapsulated as standard NMEA-0183 message strings. These messages were functionally equivalent to the messages that a shipbased AIS receiver would have produced. The onboard demodulator process was instrumented to provide additional information about the demodulation process to include how many messages were not decoded due to a variety of issues such as frame errors, missing start flags, etc. On-board demodulation also provided a significant degree of data compression. The TIE payload software was modified on orbit to capture snapshots of analog-to-digital converter samples for use in detailed analysis on the ground. However, the resulting data files were large, and given the complexities of the host spacecraft, only a few of these snapshots were successfully captured and brought to the ground. The TIE team was in the process of working on updated software that would have made this process more efficient and result in smaller data files when the mission ended.
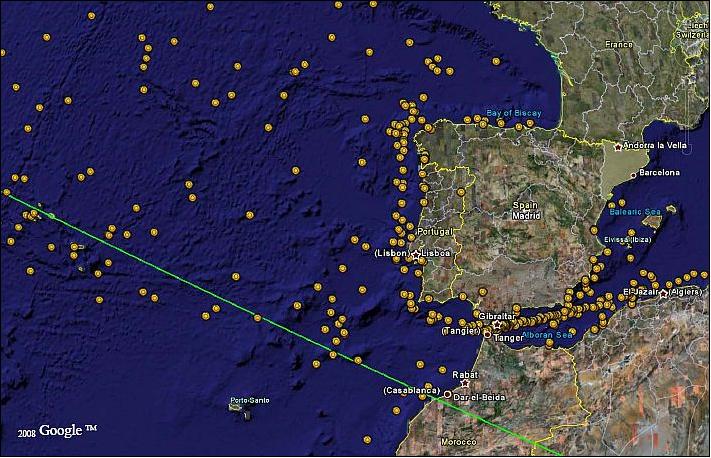

CDL (Common Data Link)
CDL is an AFRL experimental communication system (first spaceborne CDL demonstration). The objective is to use it in combination with a ground-based CDL terminals. This high-performance system permits downlink data rates of up to 274 Mbit/s (6 x 42.8 Mbit/s). The low broadcast speed is 10 Mbit/s. The CDL provides communication and imagery to the MIST (Modular Interoperable Surface Terminal), located at the Navy's China Lake, CA, facility. In addition, program personnel will assess the satellite's ability to accept commands from the MIST. By demonstrating that it can communicate directly with the China Lake facility, TacSat-2 can directly talk to any common data link compatible ground station across the globe. Flying a CDL transponder requires ground-breaking work in frequency allocations and communications security (COMSEC) approaches.
Note: DoD designated CDL as its standard for use in imagery and signals intelligence in 1991. This link consists of a secure, jam-resistant uplink operating at 200 kbit/s and a down link that can operate at various data rates. CDL is currently also being used on UAVs (Unmanned Aerial Vehicle) including Global Hawk and Predator.
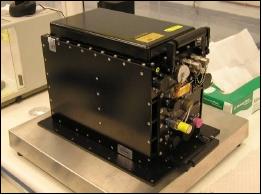
The TacSat-2 CDL is a derivative of an existing airborne CDL transceiver, based on the F-16 TARS (Tactical Aerial Reconnaissance System) radio. As such, it is mature technology; however, this is the first application of CDL in space. To accommodate a space application, modifications were made to the MMA (Microwave Modem Assembly) – mostly to the physical envelope and removing parts not required for space application. 31) 32)
There was also a software update to account for the higher Doppler Shift associated with spacecraft versus aircraft. The Doppler range for aircraft application is 0-40 kHz, where a LEO spacecraft Doppler can be as high as 180 kHz with a 5 kHz/s rate of change when passing directly over the ground station. Due to insufficient time and money, fully space qualifying a CDL transceiver was not feasible. With a minimal degree of shielding the risk was acceptable for the 6-month to 1-year mission life.
The TacSat-2 modified CDL payload provides in-theater tasking and data downlink via the MMA, uplink patch antenna and downlink ESA (Electronically Scanned Array). There are 3 primary capabilities of the TacSat-2 CDL link:
- Uplink (200 kbit/s)
- Low rate downlink (10.71 Mbit/s)
- High rate downlink (274 Mbit/s).
The ESA was sized to provide sufficient power to close the link 5 – 10º above the horizon (Figure 15 for an illustration of the CDL architecture).
• Uplink: The CDL uplink is used to command tactical downlinks. However, it is also extremely useful for uploading large software files to the spacecraft due to the higher uplink rate. Furthermore, this uplink can be used for any spacecraft commanding required, tactical or otherwise.
• Low Rate Downlink: The low rate downlink channel is used to downlink any spacecraft status, experiment, or sensor data. There is no real-time telemetry display, however.
• High Rate Downlink: The high rate downlink has an effective throughput of about 274 Mbit/s. This throughput is used exclusively by the ROPE (Roadrunner Onboard Processing Experiment). The ROPE compresses and stores all 4 bands of imagery data. Therefore, only imagery data can be passed on the high rate downlink.
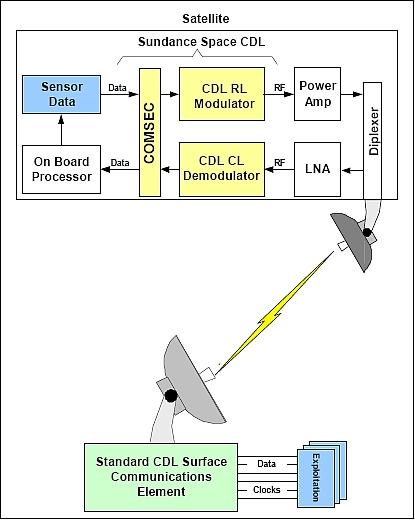
The China Lake MIST has the capability to track the expected satellite path on an input ephemeris file, or by slaving to the satellite downlink signal. Few modifications were made to the China Lake MIST for operation with a space-based CDL: software was updated to provide ephemeris tracking and physical fiber optic interface was reconfigured. The modifications were modular such that a switch between airborne and space-based CDL could be accomplished in very little time.
The RTT (Remote Tasking Terminal) is the piece of the puzzle making it easy for tactical users with little training to task the satellite. The intelligence of the systems on the RTT and the simplicity of the interface make tasking a satellite as easy as purchasing an airline ticket online.
The RTT is equipped with the RIMS (Remote Information Monitoring System) – the primary satellite command and control interface. To accommodate situations so dynamic that the tactical user does not have time to verify feasibility of execution, RIMS utilizes/interfaces with both mission planning software and ATE (Autonomous Onboard Tasking) software.
The tactical ground system provides connectivity to the Tactical Exploitation System, TES-Lite. Both imagery and signals data can be ingested by the TES-Lite for exploitation. It is then available on the SIPRNet for dissemination.
The connectivity to SIPRNet also allows remote tasking/data collection capability. This connection provided a further reach for tactical operation. If an operator was not in direct contact with a MIST ground station but had access to SIPRNet, they could remotely task and receive data from the in-theater MIST. Considering network access, even for secure networks, is more prevalent than ever, users could task the satellite from practically anywhere across the globe. Remote tasking was successfully executed during the ACTD (Advanced Concept Technology Demonstration).
The validation of TacSat-2 in-theater commanding and downlink was conducted in 2 stages: ACTD Validation and EUE (End-User Evaluation). ACTD validation occurred between December 17, 2006 and September 31, 2007; EUE between October 1, 2007 and December 21, 2007. The purpose of the EUE was to further investigate TacSat-2’s militarily useful applications, as recommended by USSTRATCOM (US Strategic Command).
In summary, TacSat-2 could be tasked virtually anywhere on the globe and deliver intelligence products utilizing resources and infrastructure already available to the warfighter in theater, including the web and the MIST. A CDL ground station like MIST is a primary tool to communicate with UAVs (Unmanned Aerial Vehicles) and now might fulfill the dual role of air and spacecraft commanding and data collection.
HET (Hall Effect Thruster)
HET was provided by AFRL at Edwards AFB, CA. HET is part of the MPI (Microsatellite Propulsion Integration) experiment. This Demonstration ion engine is based on the Busek Tandem Hall Thruster BHT-200-X3 (Busek Co. Inc., Natick, MA). HET features an Isp of up to 1600 s, variable thrust levels, and variable power usage. The nominal 200 W thruster has an operating envelope ranging from 50 to 300 W. The available thrust range is 4-17 mN with nominally 12.4 mN (43% efficiency). The instrument has a mass of < 1 kg and a size of 100 mm diameter and a length of 105 mm. HET is an experiment, it's main use is to maintain orbit against drag. Note: The BHT-200-X3 represents the first U.S.-designed and built HET to fly in space. 33) 34)

Discharge input power | 200 W |
Discharge voltage | 250 V |
Discharge current | 800 mA |
Propellant mass flow rate | 0.94 mg/s |
Thrust | 12.8 mN |
Specific impulse | 1390 s |
Propulsive efficiency | 43.5% |
IGOR (Integrated GPS On-orbit Receiver)
IGOR was developed by Broad Reach Engineering (BRE), Tempe, AZ. IGOR is of BlackJack heritage of JPL. The objective is to conduct ionospheric reflection and transmission experiments. In addition, IGOR provides the spacecraft with a precise navigation solution in support of high-precision imaging observations. The instrument has dimensions of 200 mm x 240 mm x 105 mm, a mass of 4.6 kg, and a power consumption of 16 W nominal and 23 W (peak). First flights of the IGOR device were conducted on the FormoSat-3/COSMIC mission (launch April 15, 2006) 35) 36)
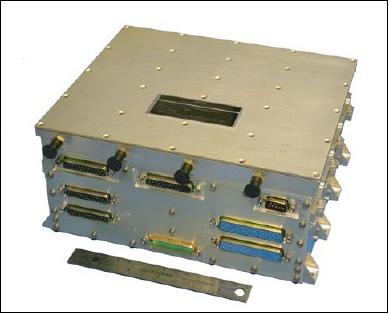
ADS (Atmospheric Density Specification)
ADS consists of two complementary experiments to characterize the neutral wind of the upper atmosphere.
• ACME (Anemometer Cross-track Measurement Experiment). ACME measures the cross-track component of the very rarified atmosphere at the orbital altitude of 350 km; it requires precise pointing knowledge to remove the spacecraft in-track velocity component from its measurement. ACME is being developed by the University of Texas at Dallas, TX.
• ADMS (Absolute Density Mass Spectrometer). ADMS measures the atomic mass of the prevailing species in the wind in the range from 1 to 50. ADMS is being developed at AFRL, Hanscom AFB, MA.
The coordinated ADS package measurements permit a better understanding of the dynamic processes that affect the variability of the upper atmosphere.
MVIS (Miniaturized Vibration Isolation System)
The objective of MVIS is to demonstrate damping of spacecraft jitter - in support of higher-quality observation measurements. MVIS consists of a series of actuators placed at the imager telescope assembly center of gravity.
ESA (Experimental Solar Array)
ESA is an innovative, low-cost, and lightweight solar array system developed by MSI. The objective of ESA is to provide a protoflight-level testing environment of a two-wing TFPV (Thin Film Photovoltaic) solar array. The experiment consists of a flexible TFPV array designed to demonstrate two different cell technologies and two different deployment mechanisms. ESA is supported by the patented FITS (Folded Integrated Thin film Stiffeners) of MSI, providing improved stiffness. FITS uses TFPV material to create a high specific power and a highly efficient stowage/deployment system. These arrays offer the potential to drastically reduce the storage volume needed for high-power microsatellites. MSI has completed a test program in validating the dynamic models of this deployment that can also be used to validate larger-scale FITS arrays. 37) 38) 39)
The baseline design for the TacSat-2 experimental solar array consists of two FITS solar array wings using TFPV on a 1 mm stainless steel substrate. Each of these wings is integrated to the end of the main rigid solar array wings of the spacecraft using a restraint system (Figure 18). One wing uses a traditional torsion spring hinge, while the other wing uses EMC (Elastic Memory Composite) hinges developed by CTD (Composite Technology Development). A further objective is to collect vital flight data to validate the predicted performance of the TFPV. This flight data is collected using the IV Electronics Box of LMA (Lockheed Martin Astronautics), Denver, CO.
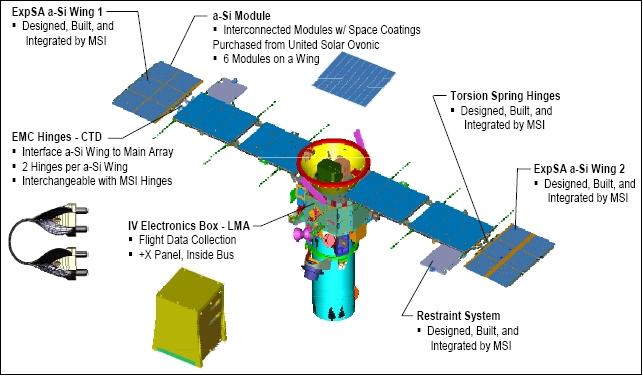
The design of the FITS experimental solar array is unique because it is attached to the outer ends of the main solar arrays of the spacecraft. It consists of the TFPV blanket assembly, composite base structure assembly, FITS stiffener assembly, tri-fold hinge assembly, electrical assembly, and the restraint system assembly. The base structure assembly is made of multiple thin composite laminates that are designed to provide stiffness to the array when deployed but also protect its vital critical components by providing a load path to the root deployment hinges. The FITS stiffeners interface with the base structure, provide the z-fold deployment force to the array and provide deployed-depth to create the required stiffness once deployed.
During the IV acquisition mode, the FITS solar array string switches are set to open, removing the experimental solar array wings from the bus (Figure 19). The IV electronics will characterize each wing separately, acquiring five sets of curve data for each.
The FITS experimental solar array will provide 120 W of additional power to the spacecraft (60 W per wing) on top of the primary solar arrays while providing valuable on-orbit performance data of the TFPV to the community for future mission planning.

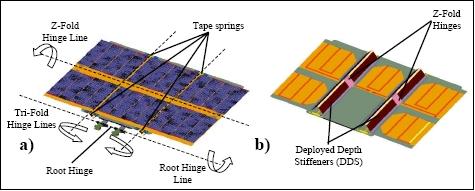
Autonomy
Autonomy is an AFRL device which was developed by ICS (Interface & Control Systems, Chapel Hill, NC). Automony consists of two subsystems: OOCE (On-Orbit Checkout Experiment), and ATE (Autonomous Tasking Experiment). The OOCE features an enabling technology for autonomously commissioning the spacecraft (checkout) during its first day of orbital life. ATE permits non-expert users in the battlefield to send data requests to the spacecraft (example: “image a particular region in latitude and longitude”) and to receive the response directly, if possible with regard to the current spacecraft location in orbit. ATE also serves as long-term and short-term experiment scheduler for all the payloads.
Major Changes of TacSat-2 During its Development Phase
Background: In 2003, the project Roadrunner was born at AFRL. The TechSat-21 contractor (MSI) received a sole-source contract to build the bus, and the program leveraged flight hardware purchased for TechSat-21 as well as a significant amount of the flight software that had been developed. While Roadrunner was in the design phase, the Office of Force Transformation (OFT) was hatching plans to leverage low-cost spacecraft as tactical theater assets. Together with the Naval Research Laboratory (NRL), they developed the concept for TacSat-1, a low-cost SIGINT platform that would provide intelligence collecting capability directly to the theater commander. 40)
The TacSat-1 effort grew into the concept of Operationally Responsive Space (ORS). This program would develop a series of TacSats and lessons learned from these experiments would give rise to a series of operational tactical spacecraft that could be called up and deployed on short notice.
The Roadrunner program team worked closely with OFT and NRL because of the similar goals they were pursuing. In 2004 Roadrunner was dubbed “TacSat-2”. This association with OFT and the ORS enterprise provided valuable advocacy for the TacSat-2 program. But at the same time, TacSat-2 was also expected to maintain consistency with the overall goals of ORS. Evolution and compromise were inevitable.
At the spacecraft manufacturer MSI (MicroSat System's Inc.), the TacSat-2 program experienced significant changes on the spacecraft bus and its payload as can be seen in comparing Figures 21 and 22 (initial design) with Figures 4 and 5 (final design), respectively. 41)
The spare parts from TechSat-21 are still being used, that's the majority of the attitude control system, and that hasn't changed since the initial design at MSI.
The spacecraft mass has changed as the flight configuration became more refined (from 415 kg initially to 370 kg as of 2006). The ACME payload was removed from the flight manifest as a weight savings measure.

Initially, the launch of TacSat-2 was planned to be on the newly developed launch vehicle Falcon-1 of SpaceX. But finally, in the spring of 2006, a launch contract was awarded to OSC (Orbital Space Corporation) to fly on a Minotaur-1 launch vehicle. The decision to abandon the unproven Falcon launch vehicle for the proven Minotaur-1 and to abandon the proven Vandenberg launch base for the unproven Wallops Island site proved to be excellent. The Minotaur continued its record of reliability and was able to set a new speed record for accommodating the TacSat-2 payload. Even more beneficial, Wallops proved to be up to the challenge of hosting an ORS launch on short notice and will likely be the chosen site of future ORS missions.
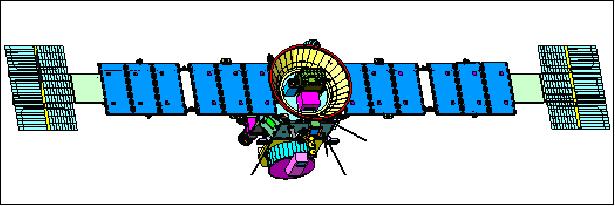
References
) T. Yee, “Roadrunner, a High-Performance Responsive Space Mission,” Proceedings of the AIAA/USU Conference on Small Satellites, Logan, UT, Aug. 9-12, 2004, SSC04-I-5
2) T. Yee, “MSI TacSat-II Lessons Learned,” Proceedings of the 19th AIAA/USU Conference on Small Satellites, Logan, UT, USA, Aug. 8-11, 2005, SSC05-IV-7
3) T. Doyne, P. Wegner, R. Riddle, M. Hurley, M. Johnson, T. Duffey, “TacSat and ORS Activity,” Proceedings of the 4S Symposium: `Small Satellite Systems and Services,' Chia Laguna Sardinia, Italy, Sept. 25-29, 2006, ESA SP-625
4) J. Raymond, P. Stadter, C. Reed, E. Finnegan, M. Hurley, C. Merk, T. Kawechki, C. Garner, P. Jaffe, G. Sandhoo, “A TacSat Experimentation Update and the Phase 3 Standardized Bus,” AIAA Space Conference, Aug 30 - Sept 1, 2005, Long Beach, CA, USA, AIAA-2005-6827
5) http://www.nasa.gov/mission_pages/tacsat-2/main/index.html
6) S. Schoneman, L. Amorosi, M. Laidley, K. Wilder, B. Hunley, “Minotaur I Demonstration of Responsive Launch for the TacSat-2 Mission,” AIAA 5th Responsive Space Conference, April 23-26, 2007, Los Angeles, CA, USA, RS5-2007-5002, URL: https://web.archive.org/web/20150607005647/http://www.responsivespace.com/Papers/RS5/SESSION%20PAPERS/SESSION%205/5002_SCHONEMAN/5002P.pdf
7) Timothy J. Rood, Jason A. Wynn, “Pioneering Technologies of the TacSat-2 Attitude Determination and Control Design,” Proceedings of the AAS Guidance and Control Conference, Breckenridge, CO, USA, Feb. 1-6, 2008, AAS 08-075
8) Terrance Yee, “Key Elements of Rapid Integration and Test,” 3rd Responsive Space Conference, April 25-28, 2005, Loa Angeles, CA, USA, URL: https://web.archive.org/web/20150607213314/http://www.responsivespace.com/Papers/RS3/SESSION%20PAPERS/SESSION%204/4001-YEE/4001P.pdf
9) T. Brady, C. Tillier, R. Brown, A. Jimenez, A. Kourepenis, “The Inertial Stellar Compass:¿ A New Direction in Spacecraft Attitude Determination,” AIAA/USU Conference on Small Satellites, Logan, UT, Aug. 12¿15, 2002, SSC02¿II¿1
10) T. Brady, S. Buckley, C. J. Dennehy, J. Gambino, A. Maynard, “The Inertial Stellar Compass:A Multifunction, Low Power, Attitude Determination Technology Breakthrough,” 26th Guidance and Control Conference, Breckenridge, CO; AAS (American Astronomical Society), Vol. 113, Feb. 5¿9, 2003, ASS¿03¿003, pp. 39¿56
11) T. Brady, “Technology Validation of the Inertial Stellar Compass (ISC),” Proceedings of the Sixth Annual NASA Earth Science Technology Conference (ESTC 2006), College Park, MD, USA, June 27-29, 2006
12) Yegor Plam, Richard Van Allen, James Wertz, Thomas Bauer, “Autonomous Orbit Control Experience on TacSat-2 using Microcosm's Orbit Control Kit (OCK),” Proceedings of the AAS Guidance and Control Conference, Breckenridge, CO, USA, Feb. 1-6, 2008, AAS 08-008, URL: http://www.smad.com/analysis/88-OCK%20AAS%2008-008.pdf
13) “TacSat / Joint Warfighting Space (JWS), April 4, 2009,” URL: http://www.globalsecurity.org/space/systems/tacsat-jws.htm
14) “Minotaur SLV Mission #6 – TacSat-2,” OSC, Dec. 16, 2006, URL: http://www.orbital.com/NewsInfo/MissionUpdates/PrinterFriendly_tacsat2.shtml
15) “Light Carbon-Fiber Structure Protects Heavy Space Cargo,” Spacedaily, Feb. 22, 2007, URL: http://www.spacedaily.com/reports...
16) P. M. Wegner, J. E. Higgins, B. P. VanWest, “Application of Advanced Grid-Stiffened Structures Technology to the Minotaur Payload Fairing,” 43rd AIAA/ASME/ASCE/AHS/ASC Structures, Structural Dynamics, and Materials Conference, April 22-25, 2002, Denver, CO, USA, AIAA 2002-1336
17) T. Yee, “How TacSat-2 is Proving the Military Utility of Web Enabled Space Operations,” AIAA 5th Responsive Space Conference, April 23-26, 2007, Los Angeles, CA, USA, RS5-2007-4002 [web source no longer available]
18) http://reentrynews.aero.org/2006058a.html
19) “TacSat-2's Milestone Mission Advanced Responsive Space Concept,” Spacedaily, Jan. 22, 2008, URL: http://www.spacewar.com/.../TacSat_2_Milestone_Mission_Advanced_Responsive_Space_Concept
20) “TacSat-2's milestone mission advanced responsive space concept,” SpaceRef, January, 17, 2008, URL: http://www.spaceref.com/news/viewpr.html?pid=24531
21) “TacSat-2 satellite gathers imagery despite conflicts as to who is in charge,” April 25, 2007, URL: http://rfdesign.com/military_defense_electronics/news/satellite_gathers_imagery_0425/
22) J. Singer, “Despite Snags, TacSat-2 Gives USAF Plenty to Chew On,” Space News, March 19, 2007, p. 12
23) R. Morris, “Responsive Space Technology: The NDIA 8th Annual Science & Engineering, Technology Conference/DoD Tech Expo, April 17, 2007, URL: http://www.dtic.mil/ndia/2007science/DAY01/robertMorris.pdf
24) R. L. Coxe, G. H. Romero, A. Pakyari, M. Leary, J. Lyke, D. Fronterhouse, “Development of the Malleable Signal Processor (MSP) for the Roadrunner On-Board Processing Experiment (ROPE) on the TacSat-2 Spacecraft; Proceedings of MAPLD 2005 (Military and Aerospace Applications of Programmable Devices and Technologies), Washington DC, Sept. 7-9, 2005, paper: A162, URL: http://klabs.org/mapld05/papers/162_coxe_paper.doc
25) “PTR Group Developed Payload Now in Orbit Onboard TacSat-2,” URL: https://web.archive.org/web/20100703185049/http://www.linux-mag.com:80/id/2822/
26) http://www.linuxdevices.com/news/NS8137978310.html
27) C. Huffine, T. Duffey, “TACSAT-2/TIE Payload Development: Enabling Rapid Development and Testing of Space Payload Hardware and Software,” Proceedings of the 22nd Annual AIAA/USU Conference on Small Satellites, Logan, UT, USA, Aug. 11-14, 2008, SSC08-II-6
28) C. J. Finley, N. Peck, “TacSat-2: A Story of Survival,” Proceedings of the 21st Annual AIAA/USU Conference on Small Satellites, Logan, UT, USA, Aug. 13-16, 2007, SSC07-VII-2
29) Christopher Huffine, “TACSAT-2 Target Indicator Experiment (TIE) AIS Payload Overview,” 2007 Maritime Domain Awareness Forum, October, 29, 2007, URL: http://www.dtic.mil/ndia/2007USCG/Huffine.pdf
30) Timothy Duffey, Christopher Huffine, Stuart Nicholson, “On-orbit results from the TacSat-2 ACTD Target Indicator Experiment AIS Payload,” Proceedings of the IAA Symposium on Small Satellite Systems and Services (4S), Rhodes, Greece, May 26-30, 2008, ESA SP-660, August 2008
31) Lisa Baghal, Randon Wright, Richard Galindez, “In a Tactical Minute: Lessons Learned From the First-Ever In-Theater Satellite Command and Downlink,” 7th Responsive Space Conference, Los Angeles, CA, USA, April 27-30, 2009, paper: RS7-2009-6002 [web source no longer available]
32) Richard Galindez, Darren Morrow, Tom Kamlowsky, Todd Wilstead, Brent Ovard, Alex Hailu, Ron Reeves, Myron E. Moore, John S. Fairbanks, “A Common Data Link (CDL) for Space-Based Communications: Migration of Airborne Hardware to Space,” Proceedings of the 19th Annual AIAA/USU Conference on Small Satellites, Logan, UT, USA, Aug. 8-11, 2005, SSC05-VIII-2
33) M. R. Nakles, W. A. Hargus Jr., D. B. VanGilder, “Comparison of Numerical and Experimental Near-Field Plasma Properties of the BHT-200-X3 Hall Thruster,” 42nd AIAA/ASME/SAE/ASEE Joint Propulsion Conference & Exhibit, July 9-12, 2006, Sacramento, CA, USA, AIAA 2006-4479
34) http://www.busek.com/index_htm_files/70008504.pdf
35) http://www.cosmic.ucar.edu/systems/IGOR_Flyer.pdf
36) BRE brochure, 2009, URL: http://www.broadreachengineering.com...
37) J W. Zuckermandel, S. Enger, N. Gupta, “Design, Build, and Testing of TacSat Thin Film Solar Arrays,” Proceedings of the 20th Annual AIAA/USU Conference on Small Satellites, Logan, UT, Aug. 14-17, 2006, paper: SSC06-VIII-4
38) J. R. Jorgensen, E. L. Louis, J. D. Hinkle, M. J. Silver, J. Zuckermandel, S. Enger, “Dynamics of an elastically deployable solar array: ground test model validation,” Proceedings of the 46th AIAA/ASME/ASCE/AHS/ASC Structures, Structural Dynamics & Materials Conference, Austin, Texas, April, 2005
39) J W. Zuckermandel, S. Enger, N. Gupta, J. Summers, “Modular, Thin Film Solar Arrays for Operationally Responsive Spacecraft,” Proceedings of the 2007 IEEE Aerospace Conference, Big Sky, MT, March 3-10, 2007
40) C. J. Finley, N. Peck, “TacSat-2: A Story of Survival,” Proceedings of the 21st Annual AIAA/USU Conference on Small Satellites, Logan, UT, USA, Aug. 13-16, 2007, SSC07-VII-2
41) T. Yee, “Adapting to Change in Small Satellites, Lessons from the TacSat Program,” Proceedings of the 20th Annual AIAA/USU Conference on Small Satellites, Logan, UT, Aug. 14-17, 2006, paper: SSC06-IV-9
The information compiled and edited in this article was provided by Herbert J. Kramer from his documentation of: ”Observation of the Earth and Its Environment: Survey of Missions and Sensors” (Springer Verlag) as well as many other sources after the publication of the 4th edition in 2002. - Comments and corrections to this article are always welcome for further updates (eoportal@symbios.space).