TWINS (Two Wide-Angle Imaging Neutral-Atom Spectrometers)
EO
Atmosphere
Ocean
Multi-purpose imagery (ocean)
Quick facts
Overview
Mission type | EO |
Agency | NASA |
Mission status | Operational (extended) |
Launch date | 28 Jun 2006 |
Measurement domain | Atmosphere, Ocean, Land, Gravity and Magnetic Fields |
Measurement category | Multi-purpose imagery (ocean), Multi-purpose imagery (land) |
Instrument type | Other, Data collection, Hyperspectral imagers |
CEOS EO Handbook | See TWINS (Two Wide-Angle Imaging Neutral-Atom Spectrometers) summary |
TWINS (Two Wide-Angle Imaging Neutral-Atom Spectrometers)
TWINS is a collaborative Sun-Earth connection two-satellite mission of NASA, LANL (Los Alamos National Laboratory), JHU/APL, SwRI (Southwest Research Institute), USC (University of Southern California), WVU (West Virginia University), The Aerospace Corporation, and the University of Bonn, Germany. TWINS is part of the NASA SMEX (Small Explorer) program. David J. McComas of SwRI is the PI (Principal Investigator) of the TWINS missions.
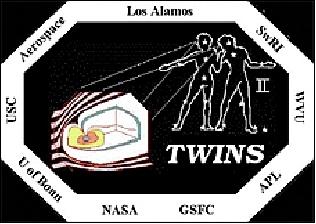
The overall mission objective is to demonstrate a new capability of stereoscopic imaging of the magnetosphere by establishing global connectivities and causal relationships between processes in different regions of the magnetosphere. By imaging the charge exchange neutral atoms over a broad energy range (about 1-100 keV) using two identical instruments on two widely spaced high-altitude and high-inclination spacecraft, TWINS will enable the 3-three-dimensional visualization and the resolution of large scale structures and dynamics within the magnetosphere for the first time. Areas to be studied by TWINS include: 1)
• Neutral atoms and charged particles
• The Earth's magnetic field
• Geomagnetic storms and substorms
• Auroras
• The 11-year solar cycle
• Space weather - the effects of the sun on human life on earth and in space.
Background
The feasibility of magnetospheric imaging using ENAs (Energetic Neutral Atoms), which arise from the charge exchange process between cold geocoronal neutral hydrogen and local energetic ion populations, was first demonstrated in the early 1990s (ENAP on ATLAS-1 (STS-45 flight, launch March 24, 1992). The POLAR mission of NASA (launch Feb 24, 1996) is flying the CEPPAD/SEPS (Comprehensive Energetic-Particle Pitch Angle Distribution / Source Loss Cone Energetic Particle Spectrometer) instrument package capable of detecting ENAs. The SAC-B mission of CONAE (launch Nov. 4, 1996) had ISENA (Imaging Spectrometer for Energetic Neutral Atoms) of CNR/IFSI on-board. Unfortunately, the third stage of the SAC-B launch vehicle failed to separate from the S/C, thus preventing any operations. The IMAGE mission of NASA (launch March 25, 2000, end of the IMAGE mission in Dec. 2005) employed three ENA imagers (LENA, MENA, HENA). It is expected that the imagery obtained by the TWINS constellation (two widely-spaced high-altitude spacecraft) may revolutionize the study of the magnetosphere.
TWINS science objectives: 2)
While TWINS has numerous scientific goals that relate to the various individual regions which will be imaged, the primary scientific goal of the TWINS investigation is to establish the global connectivity and causal relationships between processes in different regions of the magnetosphere. The IMAGE (Imager for Magnetopause-to-Aurora Global Exploration) mission of NASA (launch March 25, 2000) has taken the first important steps in imaging the magnetosphere; TWINS provides another major step forward by enabling the capability of unfolding the emission variation along the line-of-sight from the integrated ENA intensities obtained from each of the spacecraft. The stereo imaging of TWINS counters a serious difficulty with all magnetospheric imaging carried out to date: that of interpreting structures in an optically thin medium when viewed from a single vantage point; it greatly reduces the reliance on simplifying assumptions such as isotropy of the magnetospheric ions when retrieving the 3-D magnetospheric structure and dynamics from the data.
The TWINS investigation measurement goals are:
1) Ion Dynamics: To view the global dynamics, composition, and energization of ions throughout the magnetosphere with approximately 1-minute time-resolution using simultaneously obtained dual-vantage-point images of key magnetospheric components including the ring current, inner plasma sheet, near-Earth cross-tail current sheet, the high-latitude, low-altitude extensions of these regions and of the outer magnetosphere, the magnetotail, boundary layers, and cusp. 3)
2) Plasma Origins and Destinies: To trace the sources, transport, and sinks of plasma populations, including solar wind entry at the magnetopause, boundary-layer flows and convection patterns, acceleration and heating in the near-Earth plasma sheet, and acceleration of ionospheric plasma in the polar regions.
3) Magnetospheric Evolution: To observe the evolution of the global magnetospheric structure as solar-wind coupling and internal processes change the state of the magnetosphere from quiescence, through moderate (substorms) or extreme (storms) levels of activity, to relaxation and a return to quiescence.
4) Magnetospheric Structure: To visualize and map the global configuration and organization of the magnetosphere in three dimensions using stereo imaging, forward modeling, and image inversion.
These measurement goals provide the inputs required to establish the spatial connections and temporal causalities between the various magnetospheric components and regions for both active and inactive states of the magnetosphere. For example, the location in the near-Earth magnetotail current sheet where substorm current disruptions initiate remains elusive, and the timing, as well as the mapping, between the equatorial plane and the auroral zone is still unknown. Multi-point imaging holds our best hope for finally resolving these fundamental and long-standing problems by:
• providing a framework for understanding the role of localized processes in establishing the global magnetospheric configuration and dynamics
• allowing the project to extract quantitative information about the configuration and evolution of global electromagnetic parameters, such as the electric field configurations of the inner magnetosphere and the generation of the region 2 magnetic field aligned current system.
Spacecraft
The TWINS payloads are hosted on classified NRO (National Reconnaissance Office) reconnaissance missions operated by the USAF. Hence, the spacecraft cannot be identified nor described - except that they are NRO Improved Trumpet spacecraft. Their code names are USA-184 and USA-200.
Each spacecraft is three-axis stabilized and approximately nadir pointing.
Launch
The hosted TWINS-1 payload was launched aboard the NROL-22 (NRO Launch-22) mission, also referred to as USA-184, on June 28, 2006. The launcher was a Delta-4-M (4+2) vehicle and the site was VAFB, CA, USA.
- The TWINS-2 payload followed aboard NROL-28 mission, also referred to as USA-200, on March 13, 2008. The launcher on flight was was an Atlas-5-411 vehicle and the site was VAFB, CA, USA.
Prior to launch, the TWINS-1 and -2 payloads were referred to as TWINS-A and TWINS-B, respectively. — The host spacecraft USA-184 and USA-200 are being operated by NRO.
Orbit
HEOs (Highly Elliptical Orbits), also referred to as a Molniya orbits, were selected for both missions. Perigee of ~ 1100 km , apogee = 7.2 RE or 46,000 km, inclination = 63.4º, period of about 12 hours. The majority of the orbital period is spent near apogee.
Molniya orbits are unique because they maintain a fixed argument of perigee. The orbit has a period of one half of a sidereal day, giving two fixed geographic longitudes for alternate apogees. The TWINS spacecraft provide approximately nadir pointing surfaces that the TWINS instruments sit on. Such an arrangement is ideal for the TWINS scientific goals, since the imagers rotate about an axis pointed roughly towards the Earth at all times and thus will always observe the magnetosphere. Since the two spacecraft have a significant offset in their orbital phases (apogees at different times), the pair can provide nearly continuous magnetospheric observations in addition to simultaneous, dual platform viewing for a portion of each orbit.

Sensor Complement
TWINS (Two Wide-angle Imaging Neutral-atom Spectrometers)
The TWINS payloads, launched as NASA Explorer Missions-of-Opportunity , conduct stereoscopic imaging of Earth's magnetosphere for the first time. TWINS extends our understanding of magnetospheric structure and processes by providing simultaneous Energetic Neutral Atom (ENA) imaging from two widely separated locations. The Ly-α monitor measures the geocoronal hydrogen density to aid in ENA analysis while environmental sensors provide contemporaneous measurements of the local charged particle environments. By imaging ENAs with identical instruments from two widely spaced, high-altitude, high-inclination spacecraft, TWINS enables three-dimensional visualization of the large-scale structures and dynamics within the magnetosphere for the first time (Ref. 2). 4)
The TWINS ENA imager instrumentation is essentially the same as the state-of-the-art MENA (Medium Energy Neutral Atom Imager) instrument on NASA's IMAGE mission. This instrumentation consists of a neutral atom imager covering the ~1-100 keV energy range with 4° x 4° angular resolution, a 1 minute time resolution, and a simple Lyman-alpha imager to monitor the geocorona.
The sensor heads and the signal-processing electronics of TWINS, including signal amplification, trajectory calculation, time-of-flight (TOF) determination, and pulse-height analysis, are nearly identical to those of MENA, minimizing cost and risk. The largest difference between MENA and TWINS is in how the viewing plane is scanned across the sky, which is driven by fundamental differences between the spacecraft on which they are flying. For IMAGE, the spacecraft spin rotates the MENA FOV (Field of View) across the sky. Since the TWINS spacecraft are 3-axis stabilized, the sensor heads are mounted on a rotating actuator, which sweeps back and forth over an approximately Earth-centered viewing cone. This cone spans the inner and middle magnetosphere for the majority of each TWINS orbit.
Instrument mass, power | 18.7 kg, 25.3 W |
Instrument size | 57 cm x 57 cm x 30 cm |
IFOV (Instantaneous Field of View) | ± 60º centered on rotation axis |
Commanding | One 16-bit word |
Telemetry rate | 50 kbit/s |
Angle FOV | ≥ 2.5 sr centered approximately about nadir from each of 2 spacecraft |
Angle resolution | 4º x 4º for H atoms with energies > 10 keV |
Energy range | 1-100 keV for H atoms (higher energy O is also measured) |
Energy resolution | ΔE/E ≤ 1.0 for H atoms |
Time resolution | Full images in 60 s taken every 82s |
Lifetime | > 2 years |
Species identified | H, O |
Instrument geometric factor | TWINS1: 8.7 x 10-3 cm2 sr |
TWINS separately images fluxes of hydrogen and oxygen ENAs. Hydrogen ENAs from ~1-100 keV are measured with an angular resolution of 4° x 4° above ~10 keV (lower energies and oxygen ENAs are provided at reduced angular resolution). In normal mode, a full image from each of the TWINS is made in 60 s each ~82 s (including the 22 s required for the actuator to reverse direction).
Figure 3 provides a photo of the TWINS-1 instrument just prior to final flight delivery. The major subsystems of TWINS are all labeled:
• Toward Sensor Head (viewing back toward the DPU box)
• Away Sensor Head
• Lyman-α detector
• Electronics box
• Sensor Head Mounting Structure
• TWA (TWINS Actuator)
• Tilt Bracket.
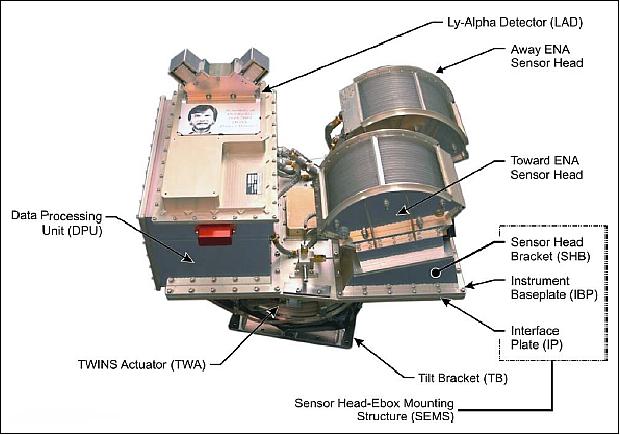
Legend to Figure 3: The DPU and sensor heads are mounted on the TWINS Actuator, which rotates the instrument back and forth. The LAD (Layman-Alpha Detector) is mounted on top of the DPU and aligned so that the "toward" and "away" LAD sensor heads point along the centers of the "toward" and "away" ENA sensor head FOVs.
The instrument package consists essentially of TWINS and a LAD to monitor the geocorona. Both instruments are mounted onto a rotating actuator platform to allow 360º azimuthal viewing. The function of the TWA (TWINS Actuator) is to rotate the TWINS instrument package over a ±90º viewing range in a windshield-wiper motion and to transfer power and data between TWINS and the spacecraft.
Mechanical Design
The design of the mechanical system was driven by the requirements to view the 3D magnetosphere from fixed (non-rotating) spacecraft in high altitude, high inclination orbits. Accommodation of the FOV of the ENA sensors and the LAD is shown in Figure 4. In order to map out the magnetosphere, the ENA sensors were mounted, along with the LAD and DPU box, on top of a rotational actuator. The ENA sensors are canted ±15° from the central rotation axis of the instrument, providing ~140° of viewing coverage with significant overlap for in-flight cross calibration. The project also tilted the actuator axis slightly (~10°) away from nadir so that sunlight would not shine continuously down into the instrument when the Earth lies in the plane precisely between the spacecraft and the Sun. A purge system was included in the instrument design so that the sensors, which contain the contamination sensitive MCP detectors, could be continuously purged throughout assembly, integration and testing.
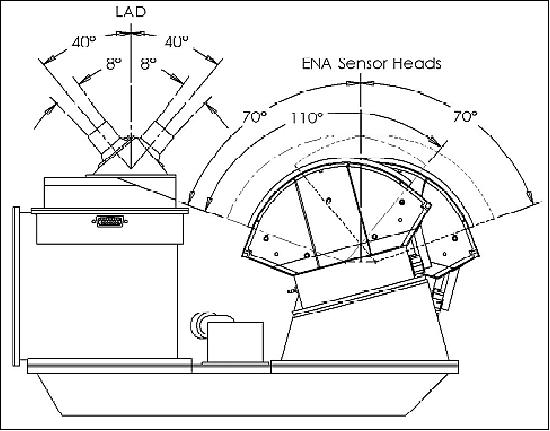
The spacecraft interface requirements also drove the mechanical system design. TWINS was designed so that it could be accommodated on the S/C in both its stowed and deployed positions. Figure 5 shows the TWINS instrument mounted in the deployed position half way through its rotation.
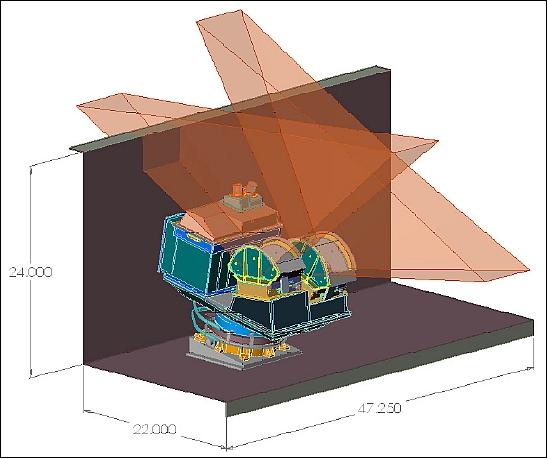
Another important driver for the TWINS mechanical system was the mission's environmental requirements. The TWINS instrument was analyzed and tested to verify that it would survive all environmental conditions. During the design of the instrument, a structural analysis was performed. In addition, a thermal analysis was performed to facilitate the thermal design of the instrument including radiator and MLI (Multi-Layer Insulation) placement. TWINS successfully completed EMI (Electromagnetic Interference), thermal vacuum, and vibration testing to all required environments following completion of instrument assembly.
Electrical system: The electronics box houses the FEE (Front-End Electronics), high and low voltage power supplies (HVPS, LVPS), and DPU (Data Processing Unit), consisting of the DP (Data Processing) and IO (Input/Output) boards. Each ENA sensor head has two pairs of CHAMPs (Charge preAmplifiers) that produce pulse signals proportional to charge collected on various anode segments. These analog signals are passed to the FEE, consisting of a PHA (Pulse Height Analyzer) board and TOF (Time of Flight) analyzer board. The digital outputs from the PHA and the TOF boards are delivered to the DP board for neutral-atom species classification and telemetry processing. The LAD (Lyman-α Detector), mounted on top of the electronics box, interfaces directly to the IO board, which provides the counters for the two LAD channel electron multiplier (CEM) detectors. The rotating actuator is under high-level control by a dedicated microcontroller on the IO board. The TWA sweeps the sensors through 180° field-of-regard in a back-and-forth, windshield-wiper mode of operation, and provides a position encoder, which is used by the DP board to resolve the scan range into 4° telemetry sectors.
Two redundant 8-wire flex-circuit ribbon cables pass the spacecraft electrical interfaces across the rotation plane. The spacecraft supplies unregulated, 28 V power to the TWINS instrument. Latching mechanical relays on the spacecraft side are used to provide independent control of primary and redundant power flow. To protect the TWINS instrument and spacecraft power system, a 5 amp, DC-rated fuse is placed on each primary and redundant harness. The fuses are sized with 100% margin on TWINS peak-power requirements during mission operations. Heater power is supplied by the spacecraft on a similar, unregulated, 28 V interface having the same redundancy and fusing topology as the main power interface.
All command and telemetry data are transmitted over a bi-directional redundant MIL-STD-1553B interface. The transfer of data is controlled by an external telemetry processing unit, which uses a polling scheme to determine if TWINS has data available for transmission. The average TWINS telemetry data rate is 50 kbit/s in the normal scanning imaging mode. There are passive telemetry interfaces on TWINS for monitoring the status of the main power relays and the instrument temperature in both power-on and power-off conditions. Two analog thermistors, one located on the rotor and one on the stator side of the actuator flex cable, are converted by the spacecraft's 8-bit housekeeping system.
Four tape heaters installed inside the TWINS interface plate provide up to 30 W of heater power when necessary. Heating is controlled by thermostatic relays, also located in the interface plate but separate from the heaters. The relays turn on at –15°C and turn off at –10°C, providing a 5° hysteresis to prevent switching noise near the thermal threshold. Four thermostats are wired in a series-parallel redundant fashion to prevent either a failed-short or failed-open condition causing loss of thermal control.
Each ENA sensor head has an internal sliding door that protects the flight gratings from acoustic energy and particulate contamination during launch. The doors are opened by dimple motors activated with a DPU circuit that delivers a high-current surge from the spacecraft 28 V heater bus. When the dimple motor is activated, its resistance changes from 1 ohm to open circuit. Another circuit in the DPU monitors this resistance and reports a status bit in telemetry.
The rotating actuator is locked during launch by a Marmon clamp. A Starsys wax pin-pusher actuator is used as the deployment mechanism for the clamp. A DPU circuit delivers 28 V, also from the heater interface, to one of two redundant wax heating elements. Depending on the instrument temperature, the wax actuator requires approximately 3 minutes to release the clamp.
All deployments are one-time activations commanded by the ground station. An arming relay is located in the power supply and in series with the three deployment circuits to protect unintentional commanding of the doors or clamp mechanisms.
ENA Sensor Heads
The TWINS sensor heads are improved versions of the sensor heads on MENA. A careful analysis of the response of MENA, using space data was carried out; this analysis was instrumental in understanding some aspects of the detailed response of that instrument. The sensor heads measure the trajectory and the velocity of each incident ENA. Figure 6 schematically illustrates how these measurements are obtained. ENAs initially pass through a collimator, which has a parallel series of alternately biased plates that collimate the sensor FOV and reject ambient ions and electrons. The sensor therefore has a planar FOV; subsequent 1-D imaging identifies the incident polar angles of the ENAs within this plane. ENAs continue to the sensor aperture, which consists of a freestanding transmission grating that rejects UV light while allowing a significant fraction of the ENAs to pass. After passing through the grating, the ENAs transit a thin foil, continue across a drift region, and are detected using a MCP (Microchannel Plate) detector with a 1D position-sensitive "stop" anode.
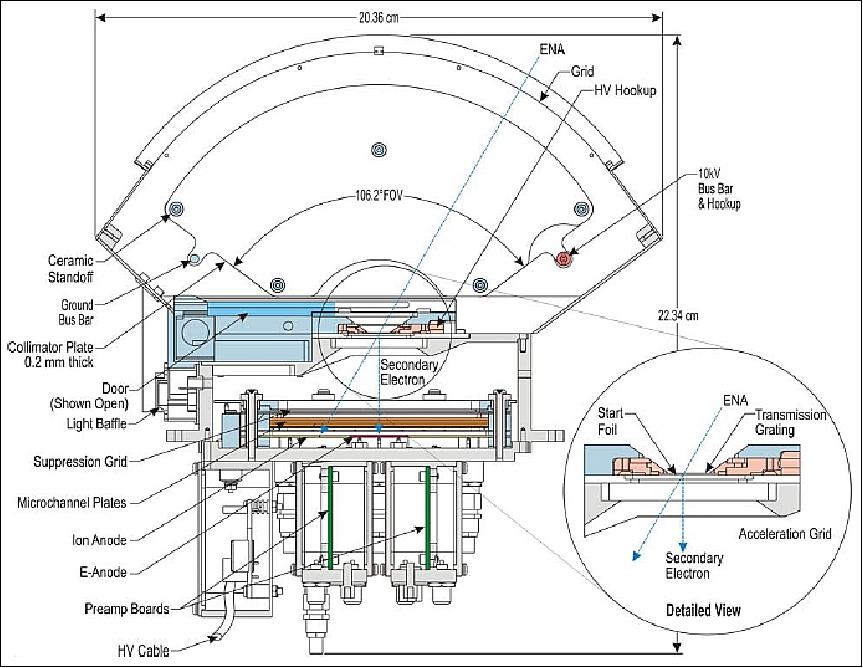
Secondary electrons generated by the ENAs at the exit surface of the thin foil are accelerated toward and detected by the same MCP detector but with a separate, 1D position-sensitive "start" anode. The detected position of the secondary electrons provides knowledge about the location at which an ENA transited the foil, and, when combined with the detected position of the ENA, the path length that the ENA traversed through the drift region. Furthermore, the secondary electrons provide information about the time that the ENA transited the foil. Therefore, comparing the detected positions and times of the ENA and its correlated secondary electrons enables measurement of the incident polar angle (Φ) and velocity (or energy assuming the ENA is hydrogen) of the ENA.
LAD (Layman-α Detector)
Inversion of the TWINS line-of-sight integrated ENA measurements requires knowledge of the geocoronal neutral density distribution. This is because the hydrogen geocorona produces the ENAs from local ions via charge exchange. For TWINS, the project has directly addressed this need by including a dual-headed Lyman-α sensor, called the TWINS-LAD, which determines via Lyman-α sky map inversions the actual geocoronal hydrogen density distribution along two central lines-of-sight within the TWINS ENA FOVs. These detectors provide line of sight integrated Lyman-α resonance emission intensities from all directions and positions over the TWINS mission profile. With the help of a numerical inversion routine the actual hydrogen densities are obtained needed for detailed analysis of the ENA signals.
The LAD is mounted on top of the DPU and consists of two identical Lyman-α detectors, which are arranged in a plane parallel to the two TWINS sensor heads and inclined by ±40° to the actuator's rotation axis. Figure 7 shows the basic measurement principle of the LAD detectors: the Lyman-α radiation enters a collimator (baffle) through an optical interference filter (BP-Filter) centered at 122 nm with a width of 10 nm FWHM. The collimator is made of blackened aluminum honeycomb material, with a length of 25.4 mm and a cell pitch of 1.53 mm, defining a circular FOV of approximately 4°. The Lyman-α radiation is then detected by a CEM (Channel Electron Multiplier) with an attached amplifier/discriminator circuit. The output pulses of the discriminator are measured by a digital pulse counter with the frequency of these pulses being proportional to the instantaneous Lyman-α intensity.
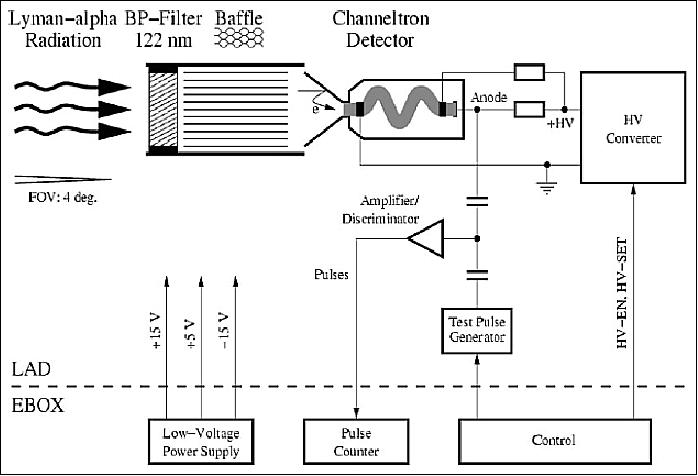
The LAD sensors were designed by the Institute for Astrophysics and Space Research, University of Bonn. The company von Hoerner & Sulger GmbH, in Schwetzingen, Germany, was the main contractor for the LAD, and did all of its development, manufacturing, and qualification. All LAD sensors were calibrated at the Berlin Electron Synchrotron (BESSY II). This facility was operated at very low currents yielding Lyman-α radiation with an intensity covering the range of expected geo-coronal values.
During the mission the actuator rotates the instrument in a windshield-wiper motion back and forth through 180° with a rotation speed of 3°/s. Since the two sensors are symmetrically oriented about the rotation axis, a full circle is mapped each rotation. The counters are read out each 0.67 seconds (corresponding to 2° at the nominal 3°/s TWA rotation rate), which yields a spatial resolution of approximately 4° x 6°. At the two end points (0° and 180°) the detectors alternately view the same region of space, thus enabling a near continuous check of their relative calibration.
To calculate the 3D geocoronal hydrogen density distribution n = n(r,ϕ,θ) from the LAD line-of-sight measurements, a theoretical hydrogen model is needed. The R. R. Hodges-model (Hodges 1994), which describes the terrestrial hydrogen exosphere up to ~60,000 km from the geocenter, based on a third-order spherical harmonic expansion, was initially used. To reduce the large number (~200) of free Hodges-parameters, a PEM (Parameterized Exospheric Model) was derived by fitting the r-dependences of the expansion coefficients given by Hodges in a set of functions. As a result, the PEM needs only 30 parameters, while still providing good agreement with the densities of the Hodges-model for different solar F10.7 cm fluxes between 80-230 x 10-22 W m-2 Hz-1.
The solar radiation input of the Lyman-α line-centered flux is derivable from terrestrial measurements of the F10.7cm flux or the equivalence-width of the solar He1083-absorption line. Depending on what other assets are available over the TWINS mission, the project will also attempt to use direct solar Lyman-α observations as input. For example, while SOHO is still functioning, the Layman-α line-centered flux will be directly available from UARS/SOLSTICE.
To separate and eliminate the interplanetary Lyman-α background radiation (~102 R) from the geocoronal radiation (~103-104 R), LISM (Local Interstellar Medium), Lyman-α intensity-maps were calculated for background subtraction, using the hydrogen distribution model of the solar system from Bzowski et al. (2002, 2003). Stellar Lyman-α emissions, for example from young, bright OB-stars, can be easily eliminated by their typical peak-profile within the LAD scans. In addition, those stellar peaks could also be used to provide an absolute "in flight" calibration of the LAD detectors. Based on the PEM and TWINS orbital geometry, the project produced a simulated set of line-of-sight, Lyman-α data. These pseudo-data have been successfully used to reconstruct the PEM by fitting over as little as one-half of an orbit.
Aperture area | 3.2 cm2 |
FOV (Field of View) | 4º FWHM, 2 detectors with lines-of-sight offset by ±40º with respect to rotation axis |
Wavelength, wavelength resolution | 121.584 nm, ±5 nm |
Sensitivity | 0.1-0.5 counts/s/R |
Sampling | Every 2º azimuthal motion (0.667 s) |
Instrument mass, power | 1 kg, 165 mW |

The LAD instrument consists of the following elements: baffle, bandpass filters, and channeltron detector. Data is collected with 2º azimuthal angle spacing. The LAD channeltrons require high voltage for operation. This is provided by a HVC (High Voltage Converter) within LAD. The HVC output voltage is enabled/disabled through a logic enable line. The exact HV value can be set through an analog control line from the TWINS electronics.
DPU and Electronics
The DPU (Data Processing Unit) is a completely new design for TWINS, owing to the differences between the IMAGE and TWINS spacecraft interfaces and the need to incorporate a rotational actuator. The DPU provides the spacecraft command and telemetry interface to TWINS and controls the ENA sensors, LAD, and TWA operations. The DPU is comprised of two boards, the data processor (DP) and interface (IO) boards, designed specifically for the TWINS instrument to optimize for low power, low mass, and fast telemetry processing. Figure 9 is a block diagram of the DP board. The DP board is an 80C186-based, single-board computer, running at 12 MHz with 256 kB of static RAM and 64 kB of program ROM. An additional 512 kB of EEPROM is provided for storing code revisions and lookup tables. An Actel RH1280 FPGA provides the glue logic for the microprocessor as well as a custom co-processing machine for constructing neutral-atom images from the FEE (Front End Electronics) raw data. The DP board also contains the 1553 interface electronics, which use UTMC's remote-terminal chipset and a dedicated 64 kB of ram for the telemetry and command buffering.

FEE (Front End Electronics): The FEE services the two ENA sensor heads and provides all of the analog and digital processing to measure the angle and speed of each incoming neutral atom and to estimate its species based on the amplitude of the MCP pulses. Together they serve to create the analog signals START_A, START_SUM, STOP_A, and STOP_SUM which are then processed by the TOF board (FEE_TOF) and the pulse height analysis board (FEE_PHA). Each sensor head is serviced by a pair of FEE_TOF/FEE_PHA boards which convert the analog pulses from the sensor into digital values for the position and pulse height of the start and stop signals.

The FEE Control board (FEE_CTL) provides an interface to the front end electronics for the software on the DPU board. The difference in arrival time between the START_SUM and the STOP_SUM signals is used to measure the TOF of the ENA. The FEE_TOF board and the FEE_PHA board have a board-to-board connector that allows them to work together as a single unit. Until a pulse breaks the low level discriminator (LLD) of the START_SUM signal, the FEE_TOF and FEE_PHA are held in reset. Once the Start signal has exceeded the LLD, a high speed, low dispersion comparator is enabled that looks for the transition of the bipolar pulse back through ground. Since the bipolar outputs of the CHAMP is a tuned circuit, like a pendulum, the time to return to 0 V will be independent of the pulse amplitude. Once this signal is received, a constant current source is used to begin charging a capacitor. A similar circuit is used to process the STOP_SUM signal and steer the current away from the capacitor after a Stop signal has arrived. The voltage on the capacitor is subsequently digitized by an A/D Converter (7672RP) and then cleared. A 15 ns time delay is inserted into the STOP_SUM signal processing to eliminate non-linearity for very short TOFs. The FEE_TOF measures TOF from 5 ns to 330 ns with a nominal resolution of 1.4 ns/LSB. After a valid ENA event has been processed, the 8-bit digital value for the TOF is passed to the FEE_CTL board.
On-board Neutral-atom Image Processing: FEE raw event data, consisting of digitized pulse heights and positions from the start and stop anodes in addition to TOF data, are input to a sequence of lookup tables encoded in an FPGA. These tables classify up to 25,000 events/s into one of 3,072 pixels. Five lookup table cycles are performed as shown in Figure 28 to construct a scalar array of polar angle, species, and energy pixels. Each pixel is initially 16 bits, which is compressed to 8 bits for transmission.
TWA (TWINS Actuator)
To provide broad magnetospheric viewing from the 3-axis stabilized TWINS spacecraft, the project included a TWA (TWINS Actuator) as shown in Figure 11 under the instrument baseplate between the TWINS interface Plate and Tilt Bracket (Figure 3). The TWA rotates back and forth in a windshield-wiper motion about an axis that is pointed ~10° from nadir. The TWA actuates over 180° in 60 s at a constant rate of 3° s-1 and then takes 22 s to slow, stop, and reaccelerate to the nominal rotational rate in the opposite direction. Thus, the TWINS entire FOV is mapped out over 60 s each 82 s. Control of the actuator is provided by the DPU.

The TWA design is based on the CAPS/ACT (Cassini Plasma Spectrometer) actuator currently flying on the Cassini mission. The TWA has a minimum operational lifetime of 4 years and was designed to minimize mechanical disturbances to the spacecraft. Starting with the CAPS actuator design, we made only the changes necessary to accommodate differing TWINS functional requirements, differences between the Cassini and host spacecraft, and upgrades of several parts. The main TWA interior parts are shown in Figure 12.

TWINS Environmental Sensor
In addition to the TWINS instruments, the TWINS spacecraft carry environmental monitors developed under the auspices of the Aerospace Corporation's Internal Research programs. The TWINS Environmental Sensor, Flight Model 1 (TWINS-ES FM1) consists of a classic three-channel dosimeter (DOS) and an electrostatic plasma analyzer that serves as a SCM (Surface-Charging Monitor). This instrument is provided for the TWINS host vehicle to gain an understanding of the charged-particle environment and its variations over the mission as well as to develop an understanding of the magnetospheric environment and dynamics observed in Molniya orbits. In addition, these observations will be of great value for interpreting the TWINS ENA observations. While not funded by the NASA TWINS program, these data are planned to be made available to the community in parallel with the TWINS observations at the TWINS web site.
The TWINS-ES is depicted in Figure 13. It has a mass of 5.9 kg and dissipates 13 W. The DOS domes are located as shown on a plate on the top of the instrument. The domes are aluminum hemispheres of thickness 0.30 mm, 1.2 mm, and 3.2. A direct measurement of total dose is made. In addition, counts are accumulated in electron and proton channels for all three dosimeters. Thresholds for electrons (protons) are, respectively, approximately 0.6 (8.5) MeV, 1.5 (16) MeV, and 3 (25) MeV. Rate and integrated counts for each channel are telemetered once per second. Figure 35 contains on-orbit measurements from the DOS. It compares the dose measured over a 149-day period with the dose predicted by the NASA models over the same time period. The measured and calculated doses are given for a 4π geometry. As has been observed in the past, the measured dose in a HEO or Molniya orbit is less on the average than that predicted by NASA models.

Legend to Figure 13: The three DOS domes are seen near the top of the photograph. The aperture of the SCM is seen near the center, and the black box at the bottom contains control and interface electronics.
The SCM is an electrostatic "top hat" type plasma analyzer (e.g., Carlson et al. 1983). It includes electrostatic entrance aperture deflectors that deflect incoming particles from out of the nominal acceptance plane by up to about ±30°, allowing sampling over a total solid angle of nearly 2π sr. Counts are accumulated every 5 ms in each of 20, 12° wide azimuthal channels. The potentials applied to the analyzer and deflector electrodes are stepped every 5 ms to measure the energy per charge spectra. The electrodes are driven by high-voltage supplies capable of providing 0 to ±2 kV, and the controlling electronics are capable of driving several sweep patterns. The standard sweep pattern steps through the analyzer voltage range in 100 steps divided equally into positive and negative polarities, thus measuring electrons and ions in turn. Steps are spaced approximately exponentially to cover a particle-energy range of approximately 3 eV – 30 keV. The deflector electrodes are controlled in a similar manner to allow measurements of a given polar-acceptance angle over all 100 energy steps. The standard sweep pattern utilizes a sequence of four deflection angles. Thus the 3D plasma distribution for both ions and electrons is sampled once every 2 s in the standard mode. Other modes are possible under ground command. The geometric factor is approximately 0.017 cm2 sr eV/eV and the data rate is 41 kbit/s.
TWINS Calibration and Instrument Performance
In addition to testing subsystems at their respective home institutions, the fully integrated TWINS instruments underwent extensive testing at the SwRI (Southwest Research Institute) Instrument Calibration Facility. Each Flight Model underwent two calibrations in this facility:
1) An extensive calibration prior to substituting engineering heads and environmental testing
2) An abridged test after reintegrating the flight heads after environmental testing.
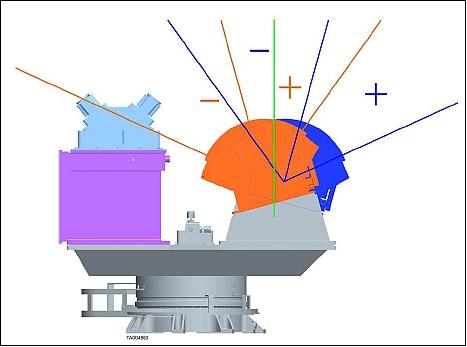
Legend to Figure 14: The TOWARD (AWAY) sensor head is shown in orange (blue) and is designated as the head looking toward or away from the electronics box. Representative FOVs of ± 50º are shown for each head. The sign convention designates negative angles as those coming from the electronics box (EBOX) side. The LAD (light blue) is notched to prevent any obstructions from the TOWARD sensor FOV. The notching in the LAD is independent of rotation angle because the DPU and LAD sit on the actuator and thus rotate with the sensors.
Measurement of the responses of all sensor heads is critical for accurate generation of ENA images. Because the detailed performances of the heads differ slightly, the project has performed extensive calibrations on each. Data from the four sensor heads are shown separately in the sections that follow; the sensor head identifications are abbreviated by flight model and direction. For example, Flight Model 1 TOWARDS head is indicated as FM1T.
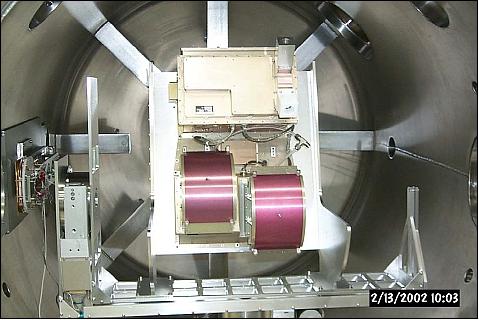
Legend to Figure 15: The TWINS FM1 is mounted in the calibration chamber in the configuration used to calibrate the AWAY head (different mounting locations are used for the TOWARD and AWAY heads so that each sensor head is centered on the beam for its calibration). The red "Remove Before Flight" covers and N2 purge line are still attached in this photo. The sensor heads were kept under continuous N2 purge until the project was ready to close the chamber door and commence pump out. All interruptions in purge (e.g., during sensor head change-out or just prior to closing the vacuum chamber door) are brief and logged. The positioning system is rotated 90° toward the left from the orientation shown in this picture to center the sensor head on the beam tube, which is covered by the beam monitor imaging MCP on the left side of the image.
In addition to performing the complete calibrations on each sensor head, the project carried out a variety of additional tests that required flight-like vacuum conditions and external stimuli. These included opening the doors under vacuum conditions, interference testing between the sensor heads and the LAD, and full level (10 kV) collimator testing. The project also did extensive UV susceptibility testing to determine if the flight heads had any light leaks and to determine the likely UV-induced background that TWINS will experience in space.
With the exception of the charge-particle-rejecting collimators, the TWINS ENA sensors are unable to distinguish between charge particles and neutral atoms. The neutral beam is produced through charge-exchange collisions with the residual background gas remaining in the beam line. The fraction of the ion beam converted into a neutral beam is only a few percent. To get the same counting statistics with a neutral beam as with an ion beam, significantly longer calibration would be required. Because of this, the majority of the calibration of the ENA sensor heads was performed with an ion beam.
TWINS Data and Science Analysis
Data from TWINS is made available to the scientific community and to the general public as quickly as possible after acquisition. The TWINS SOC (Science Operations Center) validates the data and produces higher-level products useful for scientific and educational purposes. TWINS EPO (Education and Public Outreach) includes providing data to the public and communicating the excitement of the first 3D visualization of the structure and dynamics of the Earth's magnetosphere.
Status of the TWINS Mission
• July 2015: The TWINS mission is approved to continue planning against the current budget guidelines. Any changes to the guidelines will be handled through the budget formulation process. The TWINS mission will be invited to the 2017 Heliophysics Senior Review. The NASA SMD (Science Mission Directorate) gave its approval in July 2015. 5)
• June 2015: The 2015 Heliophysics Senior Review panel undertook a review of 15 missions currently in operation in April 2015. The panel found that all the missions continue to produce science that is highly valuable to the scientific community and that they are an excellent investment by the public that funds them. 6)
- Overview of the science plan: The TWINS extended mission for 2016–2020 aims to continue 3D imaging of global ion dynamics, composition, and densities from two spacecraft on two separated orbits that each perform ENA (Energetic Neutral Atom) imaging and Lyman-alpha imaging of exospheric hydrogen atoms. The TWINS science plan continues a program of 10 overlapping science investigations covering 6 science topics. The science topics are divided into two categories: broad and targeted (3 broad and 3 targeted). Each topic comprises specific PSGs (Prioritized Science Goals) for a total of 16 PSGs.
- TWINS science strengths: TWINS' unique observational configuration and measurements provide the capability to address important questions articulated in its 16 PSGs. In particular, TWINS will enable investigation of the distribution of the extended neutral hydrogen (H) exosphere and the relative contributions of hydrogen and oxygen to the terrestrial ring current (RC) and plasma sheet, i.e., basic properties of key components of geospace. TWINS will specify the 3D spatial, energy, and pitch angle distributions of the RC, whose dynamics defines geomagnetic storms. The extended mission will elucidate how the RC and plasma sheet are energized during different phases of the solar cycle. It will also enable studying the global dynamic linkages between magnetospheric (high-altitude) and precipitating (low-altitude) ions.
- TWINS value to the Heliophysics Great Observatory: TWINS' global ENA imaging capability has synergy with other HP Great Observatory missions that perform in situ (single-point or multi-point) measurements by providing synoptic contextual information that amplify the value of local (single-point or multi-point) measurements by placing them in a global macroscopic context in relation to the many coupled geospace phenomena. Embedded within some of the science investigations of the TWINS extended mission are a number of multi-mission collaborative studies that include other members of the HSO (Heliophysics System Observatory).
Synergistic studies using TWINS, THEMIS, Van Allen Probes, and MMS can achieve cross-scale knowledge of reconnection, ring current and radiation belts, gaining insight into the relationship between RC seed populations and outer belt energization. This constellation enables simultaneous measurement of the solar wind input and the global and local geospace response, tracking the flow of energy from the dayside to the nightside and into the inner magnetosphere and ionosphere. Van Allen probes can extend this multi-scale, multi-region knowledge to include composition. Inclusion of MMS data can reveal the timing and energetics of dayside input with respect to the magnetospheric response. Correlation of TWINS inner magnetospheric imaging with IBEX imaging of the day-side magnetosheath, magnetosphere and cusp, and the night side plasma sheet from tens of RE outside the magnetosphere can provide additional information regarding the timing and causal linkages governing ion transport and energization.
- TWINS spacecraft / instrument health and status: The 2 TWINS host spacecraft are expected to continue to perform nominally through the 5-year horizon of this Senior Review. The ENA sensors on both spacecraft are operating well and continue to return high quality data. A temporary anomaly encountered in the TWINS 2 actuator motion was resolved in early 2014 by a routine recovery procedure. During the period 2013–2014, the TWINS 2 LAD (Layman-Alpha Detector) response degraded. TWINS 1 LAD continues to function nominally and is fully adequate to support continued science.
• On June 15, 2013, the TWINS mission of 2 observatories is 5 years on-orbit providing stereoscopic imaging of the ring current. In five years of operation, the TWINS maps have provided three-dimensional images and global characterization of this region. The two observatories track how the magnetosphere responds to space weather storms, characterize global information such as temperature and shape of various structures within the magnetosphere, and improve models of the magnetosphere that can be used to simulate a vast array of events. 7)

• In 2012, the TWINS ENA imagers are fully operational on their respective spacecraft.
• The first combined ENA observations from the two TWINS and the IBEX (Interstellar Boundary Explorer) missions of NASA were performed on April 5, 2010 on the arrival of an interplanetary shock and subsequent geomagnetically effective substorm. The ENA imagers on the three spacecraft were capable to observe from complementary vantage points as a powerful solar storm spewed a stream of charged particles (> 3 million km/hour) and interacted with the invisible magnetic field surrounding Earth. In fact, the TWINS and IBEX imagers observed the impact from inside and outside the Earth's magnetosphere, respectively, providing global imagery of the magnetosphere. 8) 9) 10)
- The IBEX images (taken from a distance of around 300,000 km) show an immediate compression of the magnetosphere as it was impacted by charged particles from the solar wind. ENA global imaging enabled the team to determine the precise timing of the compression, to within ±9 seconds.
- Minutes later, one of the TWINS spacecraft, carrying identical ENA sensors that provide stereoscopic imaging, observed changes in the inner magnetosphere (from a much-closer 45,000 km). A magnetospheric structure called the "ring current" traps charged particles that gyrate around magnetic field lines.
- About 15 minutes after impact, the trapped particles propagated down the field lines toward the poles and into Earth's atmosphere, where they produced additional ENAs. The brief time delay in losing particles to the atmosphere suggests that internal magnetospheric processes take some time after compression from the initial impact.
The solar storm, observed on April 5, 2010, also is thought to have caused an important communications satellite, Galaxy-15, to founder and drift, taking almost a year to return to its station.
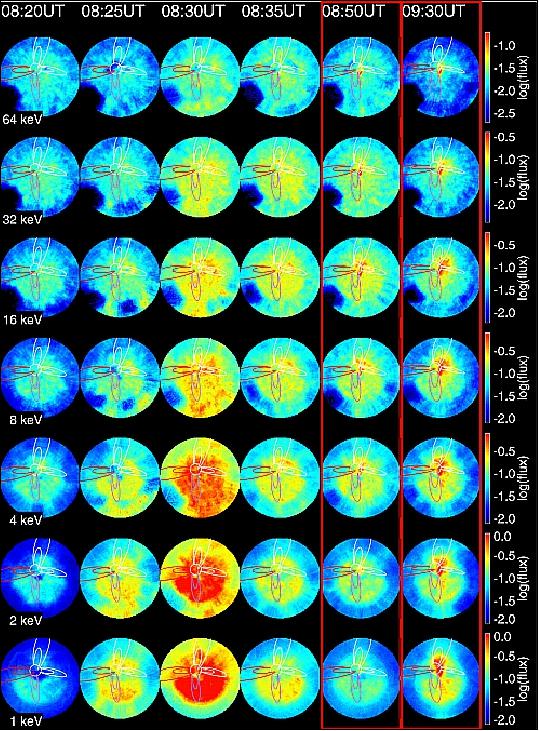
Legend to Figure 17: Each circle represents five minutes of activity, with the start times listed at the top of the graph. Each row shows a different energy band. The images in the last two columns (in red boxes) were taken roughly 20 minutes and one hour after the solar wind impact.
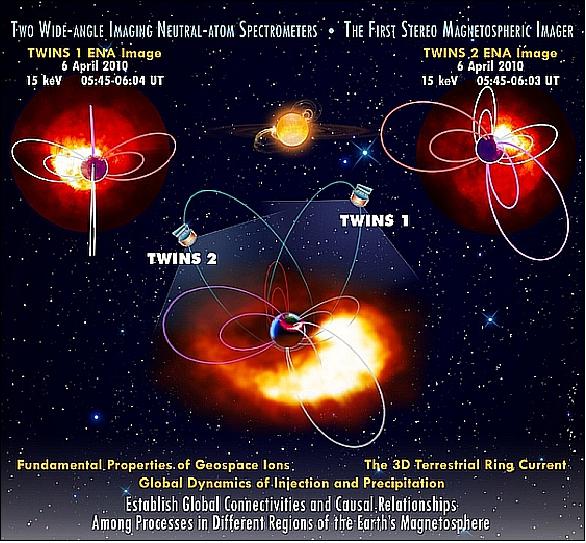
• The TWINS payloads observed the magnetic storm on 22 July 2009, which was the largest storm seen during the prolonged minimum of solar cycle 23. 12)
• Routine stereo imaging by TWINS-1 and -2 started in June 2008 (Ref. 2) during an extremely weak geomagnetic storm season. An extended and unprecendented solar minimum (from solar cycle 23) prevailed, bringing with it very calm magnetospheric conditions ranging from dead quiet to mildly disturbed.

References
1) "The TWINS Mission," SwRI, URL: http://twins.swri.edu/mission.jsp
2) D. J. McComas, F. Allegrini, J. Baldonado, B. Blake, P. C. Brandt, J. Burch, J. Clemmons, W. Crain, D. Delapp, R. DeMajistre, D. Everett, H. Fahr, L. Friesen, H. Funsten, J. Goldstein, M. Gruntman, R. Harbaugh, R. Harper, H. Henkel, C. Holmlund, G. Lay, D. Mabry, D. Mitchell, U. Nass, C. Pollock, S. Pope, M. Reno, S. Ritzau, E. Roelof, E. Scime, M. Sivjee, R. Skoug, T. S. Sotirelis, M. Thomsen, C. Urdiales, P. Valek, K. Viherkanto, S. Weidner, T. Ylikorpi, M. Young, J. Zoennchen, "The Two Wide-angle Imaging Neutral-atom Spectrometers (TWINS) NASA Mission-of-Opportunity," Space Science Review, Vol. 142, July 27, 2008, URL: http://twins.swri.edu/pubs/TWINS_Instrument_Paper_vL_-_Submitted.pdf
3) "Global Imaging of the Ring Current," URL: http://twins.swri.edu/imaging.jsp
4) H. U. Nass, J. H. Zoennchen, G. Lay, H. J. Fahr, "The TWINS-LAD mission: Observations of terrestrial Lyman-α fluxes," Astrophysics and Space Sciences Transactions, Feb. 27-31, 2006, URL: http://www.astrophys-space-sci-trans.net/2/27/2006/astra-2-27-2006.pdf
5) "NASA Response to the 2015 Senior Review for Heliophysics Operating Missions," NASA, July 10, 2015, URL: http://science.nasa.gov/media/medialibrary/2015/07/10/NASAResponse2015SeniorReview_FINAL.pdf
6) "The 2015 Senior Review of the Heliophysics Operating Missions, NASA, June 11, 2015, URL: http://science.nasa.gov/media/medialibrary/2015/07/10/HeliophysicsSeniorReview2015_FINAL.pdf
7) Karen C. Fox, "Five Years of Stereo Imaging for NASA's TWINS," NASA, News, June 21, 2013, URL: http://www.nasa.gov/mission_pages/sunearth/news/twins-5years.html
8) David J. McComas, Natalia Buzulukova, Martin Connors, Maher A Dayeh, Jerry Goldstein, Herbert O. Funsten, Stephen A. A. Fuselier, Nathan Schwadron, Philip Valek, "TWINS and IBEX ENA imaging of the 5 April 2010 substorm," Journal of Geophysical Research, Vol. 117, A03225, March 2012, doi:10.1029/2011JA017273
9) "TWINS and IBEX observe impact of powerful solar storm from inside and outside Earth's magnetosphere," Space Daily, March 30, 2012, URL: http://www.spacedaily.com/reports/TWINS_and_IBEX_observe_impact_of_
powerful_solar_storm_from_inside_and_outside_Earth_magnetosphere_999.html
10) Karen C. Fox, "Teamwork: IBEX and TWINS Observe a Solar Storm,"Space Daily, April 13, 2012, URL: http://www.spacedaily.com/reports/Teamwork_IBEX_and_TWINS_Observe_a_Solar_Storm_999.html
11) TWINS home page, URL: http://twins.swri.edu/index.jsp
12) M.‐C. Fok, N. Buzulukova, S.‐H. Chen, P. W. Valek, J. Goldstein, D. J. McComas, "Simulation and TWINS observations of the 22 July 2009 storm," Journal of Geophysical Research, Vol. 115, A12231, doi:10.1029/2010JA015443, 2010, URL: http://tinyurl.com/porzgqp
The information compiled and edited in this article was provided by Herbert J. Kramer from his documentation of: "Observation of the Earth and Its Environment: Survey of Missions and Sensors" (Springer Verlag) as well as many other sources after the publication of the 4th edition in 2002. - Comments and corrections to this article are always welcome for further updates (eoportal@symbios.space).