VELOX-1 NSat (NanoSatellite) and VELOX-1 PSat (PicoSatellite)
EO
Mission complete
ISSL UT
Quick facts
Overview
Mission type | EO |
Agency | ISSL UT |
Mission status | Mission complete |
Launch date | 30 Jun 2014 |
End of life date | 30 Jun 2016 |
CEOS EO Handbook | See VELOX-1 NSat (NanoSatellite) and VELOX-1 PSat (PicoSatellite) summary |
VELOX-1
Overview Spacecraft Launch Mission Status Sensor Complement References
The VELOX-1 mission is comprised of the VELOX-1 NSat (NanoSatellite) and the VELOX-1 PSat (PicoSatellite) to demonstrate inter-satellite communications in orbit. Also, the project allows engineering students to participate in a multidisciplinary hands-on space project as VELOX is the first nanosatellite of Singapore, designed and built at NTU (Nanyang Technological University).
Secondary mission objectives include the acquisition of high-resolution Earth imagery and the demonstration of various payloads such as a vision system, a dual-field of view sun sensor and a quantum physics payload. 1) 2)
Spacecraft
The VELOX NSat is a 3U CubeSat that measures 10 x 10 x 34 cm with a total mass of 4.3 kg that include the PSat of mass 193 gram with a size of 6 x 7 x 3 cm. The NSat consists of an Al alloy structure with load bearing parts made from stainless steel. The separation system, solar panel deployer and the optics extension system all use spring-loaded mechanisms for deployment after launch.
Attitude determination is provided by two IMUs (Inertial Measurement Units), one dual-field of view sun-sensor and eight coarse sun sensors. Stabilization and three-axis control is accomplished by three reaction wheels with three magnetic torquers for momentum dumps. A GPS receiver is used for orbit determination.
NSat features four deployable solar panels hosting GaAs solar cells delivering a peak power of 28.8 W. A 5.2 Ah Li-ion battery is used for power storage and a dedicated distribution unit delivers power to all satellite subsystems and payloads. Thermal control is ensured by multilayer insulation and heaters that maintain survival temperatures.
Dipole antennas are installed on the satellite for communications in the UHF and VHF bands reaching data rates of 9.6 kbit/s for downlink using BPSK modulation and 1.2 kbit/s for uplink that uses the AFSK protocol. NSat is controlled by a main processor operating at 100 MHz using a 2 GB SD card for data storage and a UART (Universal Asynchronous Receiver/Transmitter) and I2C data interface to connect to the various satellite systems.
Dimensions | NSat: 10 cm x 10 cm x 34 cm; PSat: 6 cm x 7 cm x 3 cm |
Mass | NSat: 4087 gram; PSat: 193 gram; total: 4.28 kg |
Expected lifetime | NSat: 24 months; PSat: 12 months |
Orbit | Sun-synchronous LEO (Low Earth Orbit), 650 – 700 km altitude |
ADCS (Attitude Determination and Control Subsystem) | NSat: 3-axis stabilized and controlled with 1 GPS receiver, 2 IMUs, 1 dual-FOV sun sensor, 8 coarse sun sensors, 3 magnetic torquers and 3 RWs (Reaction Wheels) |
On-board data handling | Main board with 100 MHz 8051 MCU, 2 GB SD card, UART and I2C data interfaces |
RF communications | 9.6 kbit/s BPSK downlink / 1.2 kbit/s AFSK uplink, UHF & VHF dipoles |
EPS (Electric Power Subsystem) | NSat: 4 deployable GaAs panels for 28.8 W peak, 5200 mAh Li-ion battery |
Structure | Al. 7075 chassis, with stainless steel/Ti-6Al-4V load bearing parts; spring-loaded separator, solar panel deployer, and optics extension mechanism |
Thermal control | MLI (Multi-Layer Insulation) and battery heaters |
Payloads | Vision system with extended optics for 20 m GSD, quantum physics payload |
Ground segment | Ground station in NTU with UHF/VHF high-gain cross Yagi-Uda antennas |
3)
Wireless Standard and Protocols for Satellite Applications:Table 2 shows a summary of the specifications of some wireless communication standards. The use of existing wireless standards and protocol approach for the implementation of a WSN (Wireless Sensor Network) in space applications can lower the costs and risks of development, integration and operations . For some applications, a custom wireless solution may be required to be developed and integrated into the system. In any case, this should also be included in an effort into the standardization of wireless standards and protocols for future satellite missions. Investigations have been conducted on the use of wireless standards for space applications. A short review is provided of the wireless standards and assess their suitability for implementation in intra-satellite and inter-satellite communication in a WSN.
The satellites (NSat as well as PSat) carry a commercial off-the-shelf wireless RF module to establish a ZigBee network. The successful execution of this experiment will provide a platform for future formation flying mission.
ZigBee is a protocol which builds on the physical layer (PHY) and MAC (Medium Access Control) layer of the IEEE 802.15.4 standard by providing the network (NWK) and framework for application layer. The support in ZigBee for star, mesh and network topologies allows for the integration of a large number of wireless sensor modules for intra-satellite communication. The use of ZigBee PRO systems, which can operate at higher transmission powers (up to 20 dBm), can also be considered for inter-satellite communication in which satellites are orbiting in close distances (<5 km).
Parameter | IEEE 802.15.4 (ZigBee, WirelessHART, ISA100.11a) | IEEE 802.15.1 | IEEE 802.11 | IEEE 802.16 (d/e) |
Maximum data rate | 250 kbit/s | 1 Mbit/s (Classic) | 600 Mbit/s | 1 Gbit/s (fixed) |
Modulation | DSSS | GFSK =4-DQPSK, | DSSS OFDM | OFDM |
Operating frequencies | 868 MHz, 915 MHz, | 2.4 GHz | 2.4, 3.65, 4.9, 5, 5.9 GHz | 2–66 GHz (fixed) |
Typical Tx power | 1–100 mW | 100 mW max (class 1) | 100 mW (up to 1 W) | Base station (20 W) |
Network topologies | Star, mesh, ad hoc | Piconet | Point-to-multipoint, | Point-to-multipoint mesh |
Network size | 65536 | 8 | 32 | Dependent on system capacity |
Typical terrestrial range | 10–50 m (indoors) | 100 m (class 1) | Up to 150 m (indoors) | Up to 48 km outdoor |
Satellite communication applications | Intra-satellite Intersatellite (<5 km) | Intra-satellite Inter-satellite (<5 km) | Intra-satellite Inter-satellite (<5 km) | Inter-satellite (≥5 km) |
The CSMA/CA contention-based strategy employed by ZigBee in its MAC layer does not provide reliability of data transfer as packet loss may occur which is unacceptable for critical applications. As such, WirelessHART or IEC 62591, a wireless protocol designed for industrial measurement and control process in harsh environment with severe interference may be more suitable for critical intra-satellite communication applications instead. WirelessHART uses a channel hopping technique to switch between different frequency channels in the presence of disruptive interference. In addition, WirelessHART is based on a TDMA scheme to provide a time-guaranteed communication. TDMA avoids contentions among nodes and ensures a bounded transmission delay. A time synchronization scheme is thus required to exchange time offset data which means that the power consumption of a RF module using WirelessHART is typically higher than ZigBee.
PSat (PicoSatellite)
The PSAT, also referred to as VELOX-PIII, is about the size of an i-phone (70 x 60 x 30 mm) and will be released in the later part of operation (few months later). It uses body-mounted solar cells to generate sufficient electrical power to operate the satellite controller and a communications system that will be used for a demonstration of inter-satellite communications. After launch and checkouts, the PSAT will be separated from NSAT by springs and start drifting away. Communication demonstrations will then begin to test out a space-to-space data link between the two satellites as the distance increases to determine the maximum possible range.
For wireless communication between the satellites, the ZigBee network protocol is used, based on the IEEE 802.15.4 standard in the 2.4 GHz frequency band (Ref. 3).
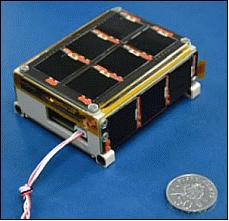
WSN (Wireless Sensor Network) for Inter-Satellite Communication Experiment Plan:
The NSat RF module is powered off until it is scheduled to turn on from the ground station command. When powered up, the NSat RF module forms a ZigBee network and initiates a thermal knife cutting process to separate the PSat by spring. The PSat is powered on after separation and will start searching for the network formed by the NSat. Once the network is found, the PSat will send an association request to join the network.
After NSat determines that PSat has joined the network, it will start transmitting a test packet to the PSat every 2 seconds. Upon successful reception of the test packet, PSat will also send back its housekeeping data that includes the temperature, voltage and current sensors' readings. The received signal strength (RSSI) from the last packet is also recorded by NSat to estimate the distance between the satellites. If NSat does not receive a data poll request from PSat for the predefined period of time, it will assume that PSat has moved out of the communication range and will dissociate the PSat from the network. NSat will then continue to monitor its network until the PSat module reassociates.
To save power, PSat is configured to sleep for a period of 320 ms when it is associated and then will wake up to check for data. If no data is available, it will go back to sleep after 10 ms. Otherwise, it will send a regular poll transmission for every 100 ms and will go back to sleep for 5 seconds after the last received packet. On the other hand, if no acknowledgement is received by the PSat after 3 consecutive polls, it will leave the current network and attempt to join a new one. When it is not associated with a network, it will go into sleep and wake up to perform 9 scans/minute for the first 5 minutes, and 3 scans/minute thereafter (each scan takes ~1.69 s).
The inter-satellite communication experiment is terminated, when the mission time exceeds 30 minutes by switching off power to the NSat RF module. In addition, if the NSat and PSat are determined to meet up again after the existing ground pass, the ground station will issue a command to power on the NSat RF module to repeat the experiment.
Preflight Experimental Results:
Range validation: The RF communication range between the satellites has been evaluated experimentally. Since it is impossible to conduct experiments in a space environment, the range validation was conducted in the university campus. To ensure sufficient clearance is available in LoS (Line of Sight), the NSat and the PSat are placed at the highest points on campus.
For the experiment, 10 bytes of data were sent in each packet and at least 200 packets were sent for each set of measurement. The distances between the two RF modules were determined using GPS. The results are presented in Table 3.
Distance (m) | Mean RSSI (dBm) | Number of valid RSSI packets | Packet success rate (%) | Total number of packets sent |
88 | -72.1096 | 146 | 83.9024 | 277 |
The test results in Table 3 show that the RF modules can communicate up to 1000 m. At 1100 m, only two packets can be received out of the 292 packets sent. Hence, it can be concluded that a range of 1 km is achievable for the inter-satellite communication on the University campus.
The data obtained are shown in Figure 5 which shows the RSSI measurements at distances up to 1000 m together with the best fit line to the log normal shadowing model. Fitting the experimental data to the log normal shadowing model gives a path loss exponent of 1.76 with RSSI of -70.68 dBm at a reference distance of 100 m.

Power consumption: The power consumption of the PSat is shown in Table 6. From Table 6, it is observed that the battery of the PSat can last up to 33 h without charging with the satellites within communication range. Assuming a power tracking efficiency of 65%, the 6-cell solar panel on one side of the PSat is estimated to provide an input current of 0.2535 A at a voltage of 2.35 V which translates in to an input power of 0.596 W. Assuming that the PSat is in eclipse for 40 min in an orbital period of 97 min, the available input energy over one orbit is 0.596 W x 57/60 = 0.566 Wh. The energy consumption assuming the satellites are within communication range over one orbital period is 0.3233W x 97/60 = 0.5227 Wh. Thus, even if there is only one side of the PSat solar panel facing the sun, it should provide enough solar power for continuous operation.
In actual operation, the PSat is rotating after it is separated from the NSat. Hence, more than one side of the PSat solar panels will be facing the sun to provide a higher operating power budget (Ref. 3).
This experiment represents the first worldwide inter-satellite communication system using ZigBee with a picosatellite being piggyback launched from a nanosatellite. It is expected, that the execution of the VELOX-1 mission will provide invaluable data for future studies into intra-satellite and inter-satellite communication systems with more complex design.
Launch
VELOX-1 was launched as a secondary payload on June 30, 2014 on the PSLV-C23 vehicle of ISRO from SDSC (Satish Dhawan Space Center), Sriharikota, India. The primary payload on this flight was SPOT-7, a commercial imaging satellite of Airbus Defence and Space (Ref. 2). 4) 5) 6)
Orbit: Sun-synchronous orbit, altitude of ~660 km, inclination = inclination = 98.2º.
The secondary payloads on this flight were:
• CanX-4 and CanX-5, a pair of identical nanosatellites (each of 15 kg) of UTIAS/SFL (University of Toronto, Institute for Aerospace Studies/Space Flight Laboratory), Toronto, Canada.
• AISAT (Automatic Identification System Satellite), a technology demonstration nanosatellite (14 kg) of DLR (German Aerospace Center).
• VELOX-1 is a 3U CubeSat with a mass of 4.3 kg of SaRC (Satellite Research Center) at NTU (Nanyang Technological University), Singapore.
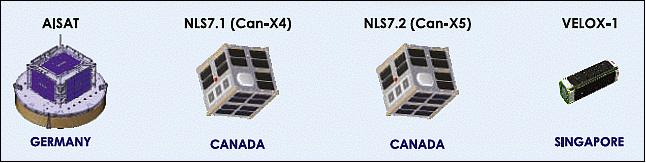
Mission Status
• July 3, 2014: The ground contact has been established successfully. The satellite has been detumbled (i.e., the in-house developed reaction wheel is working well) and is in sun pointing mode. The project has conducted data downloading. The satellite is healthy and all the deployment mechanisms have been successfully activated including the solar panels, antennas, and optics. 7)
Sensor Complement (QPP, Imager)
QPP (Quantum Physics Payload)
The QPP installed on NSAT is 10 cm x 10 cm x 3 cm in size with a mass of about 300 gram. The payload uses a laser diode to create photons, a logic circuit and a pair of photodiode detectors.
QPP uses miniaturized equipment to generate photon pairs to demonstrate quantum entanglement in orbit – a phenomenon that occurs when pairs or groups of particles interact in a way to that the quantum state of each particle can not be described independently, only the state of the system as a whole can be described. Quantum entanglement has been the subject of many studies on the ground and in space; however, many fundamental questions remain open. Flying quantum physics payloads on CubeSats allows many of such experiments with different parameters to be flown to gather a large data set on quantum entanglement in the space environment.
Imager
The second payload of the NSAT nanosatellite is an optical imager that consists of an optics system, a CMOS sensor, an FPGA data acquisition board and a PPU (Payload Processing Unit). The optics unit features use an extendable architecture — the required focal length is achieved by deploying the optics via a spring-loaded mechanism. The imager uses a 10.5 cm focal length that focuses the image into a radiation-hardened CMOS image sensor. Data from the sensor is retrieved via a field gate programmable array with 32 MB RAM.
1) “VELOX-1 Nanosatellite,”Nanyang Technological University, Singapore, URL: http://www.sarc.eee.ntu.edu.sg/Research/Projects/Pages/VELOX-I.aspx
2) Patrick Blau, “India's PSLV Rocket successfully delivers SPOT-7 & Small Satellites to Orbit,” Spaceflight 101, June 30, 2014, URL: http://www.spaceflight101.com/pslv-c23---spot7-launch-updates.html
3) Shuanglong Xie, Guo Xiong Lee, Kay-Soon Low, Erry Gunawan, “Wireless Sensor Network for Satellite Applications: A Survey and Case Study,” World Scientific Publishing Company, Unmanned Systems, Vol. 02, Issue 03, July 2014, pp: 261-267
4) “PSLV-C23 brochure,” ISRO, URL: http://www.isro.org/pslv-c23/pdf/pslv-c23-brochure.pdf
5) Alice Chia, “Singapore’s first nano-satellite launched,” Channel News Asia, July 3, 2014, URL: http://www.channelnewsasia.com/news/singapore/singapore-s-first-nano/1232324.html
6) “Two new Singapore satellites up in space, designed and built by NTU students,” The Straits Times, Singapore, July 3, 2014, URL: http://www.straitstimes.com/news/singapore/more-singapore-stories/story/two-new-singapore-satellites-space-designed-and-built-nt
7) Information provided by Kay Soon Low, Associate Professor of NTU and Director of the Project VELOX, Singapore
The information compiled and edited in this article was provided by Herbert J. Kramer from his documentation of: ”Observation of the Earth and Its Environment: Survey of Missions and Sensors” (Springer Verlag) as well as many other sources after the publication of the 4th edition in 2002. -Comments and corrections to this article are always welcome for further updates (eoportal@symbios.space)
Overview Spacecraft Launch Mission Status Sensor Complement References Back to top