FormoSat-5 (Formosa Satellite 5)
EO
Operational (nominal)
NSPO
AIP
FormoSat-5 is the fourth mission in the FormoSat series of Taiwan’s National SPace Organization (NSPO), in collaboration with the Instrument Technology Research Center (ITRC) of the National Applied Research Laboratories (NARL). As Formosat-3 was responsible for observations on meteorology and climate, and the number 4 is considered to bring bad luck in the Taiwanese culture, FormoSat-5 is a follow-up to FormoSat-2. It was launched in August 2017 with the objective to enhance the domestic capability for a high-resolution optical remote sensing instrument. It is nominally operational until the end of 2022.
Quick facts
Overview
Mission type | EO |
Agency | NSPO |
Mission status | Operational (nominal) |
Launch date | 24 Aug 2017 |
Instruments | AIP, RSI |
CEOS EO Handbook | See FormoSat-5 (Formosa Satellite 5) summary |

Summary
Mission Capabilities
FormoSat-5 is equipped with a Remote Sensing Imager (RSI) as its primary payload, as well as an Advanced Ionospheric Probe (AIP).
The RSI instrument includes a Cassegrain telescope, whose Focal Plane Assembly (FPA) is of utmost importance in terms of technology and performance for space applications. Its detector arrays utilise CMOS (Complementary Metal Oxide Semiconductor) technology and are grouped into a panchromatic sensor and a multispectral one. The mission employs DWT (Discrete Wavelet Transfer) and BPE (Bit Plane Encoder) techniques for on-board compression of the very high imagery volume.
Applications of the RSI imagery include urban development, environment and crop health monitoring, as well as disaster assessment.
The AIP instrument is a plasma sensor, capable of measuring ionospheric plasma concentrations, velocities, temperatures and ambient magnetic fields over a wide range of spatial scales. To perform these different types of measurement under various conditions, AIP operates in four modes: PLP (Planar Langmuir Probe), RPA (Retarding Potential Analyzer), IT (Ion Trap) and IDM (Ion Drift Meter). The data collected by AIP is used for space science and seismic studies.
Performance Specifications
RSI works as a pushbroom imager featuring either panchromatic imagery with a 2 m GSD (Ground Sample Distance), or multispectral imagery with a 4 m GSD. In the latter operation mode, it is sensitive to four spectral bands: near-infrared, red, green, blue. The Cassegrain telescope’s primary mirror has an aperture of 0.45 m. RSI has a swath width of 24 km.
AIP has a FOV (field-of-view) of 56°. Its accuracy varies from one parameter to another: it is 10% for ion composition and total ion concentration, ±50 m/s for cross track direction of ion velocity and ±200 m/s for ram direction, ±200 K for ion and electron temperature.
FormoSat-5 maintains a sun-synchronous near-circular orbit at an altitude of 720 km and an inclination of 98.28°, with a 99.19 minute period. It has a revisit time of 2 days.
Space and Hardware Components
FormoSat-5 is a minisatellite of octagonal shape (3 m in height and 1.2 m in diameter), with a wet mass of approximately 525 kg. With a design life of five years, the spacecraft is 3-axis stabilised and has an agile body-pointing capability, with a ±45° field-of-regard (cross-track or along-track).
The Attitude Orbit and Control Subsystem (AOCS) will be provided by a gyro-stellar attitude determination system, which utilises attitude data provided by three Camera Head Units and rate data provided by four single-axis fibre-optics gyros.
The Command and Data Management Unit (CDMU) is used for spacecraft control.
The EPS (Electrical Power Subsystem) is composed of a PCDU (Power Control Distribution Unit), a rechargeable battery and two deployable solar generators.
FormoSat-5
Spacecraft Launch Mission Status Sensor Complement Ground Segment References
The FormoSat-5 (FS-5) program represents the first indigenous development of remote sensing capabilities at NSPO (National SPace Organization) of Taiwan. After successfully conducting three satellite missions during in the timeframe 1991-2006, NSPO started a new space initiative in 2004 which emphasizes on building up the capabilities for independent development of spacecraft and payload instruments. The strategy for the program is to acquire key technology and setup a heritage bus design for NSPO. The key technology to be developed by NSPO includes flight software, EGSE and IPS. 1) 2) 3) 4) 5) 6) 7)
FormoSat-5 is a follow-on mission of FormoSat-2. An important objective for FormoSat-5 is to build up the domestic capability for the high-resolution optical remote sensing instrument by integrating NSPO with the ITRC (Instrument Technology Research Center) of NARL (National Applied Research Laboratories). Both NSPO and ITRC belong to the NARL, a non-profit organization under the supervision of NSC (National Science Council).
Development strategy: In the past two decades, NSPO has successfully finished three space programs mainly through prime contractors from either USA or Europe. NSPO engineers have been broadly involved in system & subsystem designs and became familiar with the systems engineering practices utilized by both European and USA aerospace companies. - For the FormoSat-5 program, NSPO has decided to take a bold step on taking up the full responsibility on satellite systems/subsystems analyses/designs, component development, and payload/satellite integration and test, as shown in Figure 1. 8)
This program is the first self-reliant space program in Taiwan and is serving as a launching platform to domestically build important spacecraft components. Under the guidance of NARL, NSPO has integrated high technology industries, research institutes and companies into a competent team to execute the program. Key team players include CSI (COMOS Sensor Inc.), Camels, ITRC (Instrument Technology Research Center), the National Chip Implementation Center (CIC), AIDC (Aerospace Industrial Development Corporation), and CSIST (ChungShan Institute of Science and Technology). Key components for the FormoSat-5 spacecraft bus include the PCDU (Power Control and Distribution Unit), CDMU (Command and Data Management Unit), and FSW (Flight Software). The key components for the RSI payload include: FPA (Focal Plane Assembly) and EU (Electronic Unit) are 100% developed in Taiwan.
In addition, NSPO obtains outsourcing components through STI (SpaceTech GmbH, Immenstaad), Germany. SpaceX (Space Exploration Technologies Corporation) will provide the launch service.

Spacecraft
NSPO started with the development of the FormoSat-5 project in 2005. The design strategy of the spacecraft bus includes the following major considerations: 9) 10)
1) To develop an NSPO heritage platform architecture capable of extending to the future high-resolution RSS (Remote Sensing Satellite).
2) To develop the avionics, flight software, and control algorithms extendable and reusable to the future missions
3) To achieve cost effective designs through revising product assurance strategies and acquiring COTS (Commercial Off The Shelf) components.
The spacecraft bus is shown in Figure 2. FormoSat-5 is a minisatellite of octagonal shape (3 m in height and 1.2 m in diameter) with a wet mass of ~ 525 kg. The spacecraft design life is 5 years. The spacecraft is 3-axis stabilized. The design provides for an agile body-pointing capability of the spacecraft with a FOR of ±45º (cross-track or along-track).
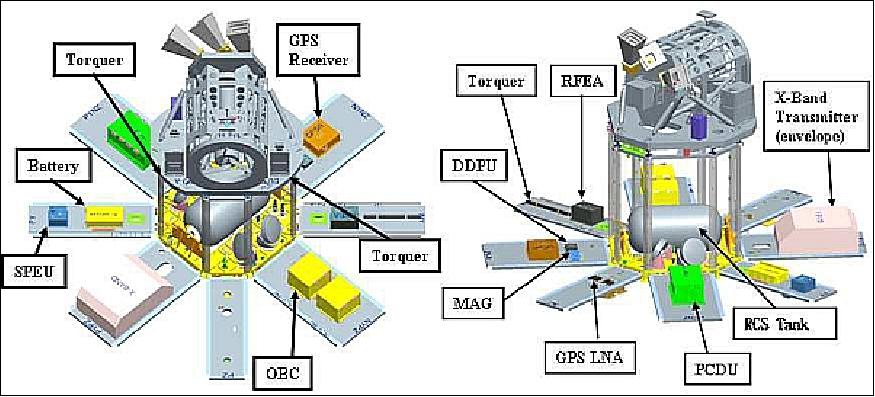

Pointing requirements: The FormoSat-5 spacecraft is 3-axis stabilized. The geo-location accuracy requirement on-board defined in FS-5 system requirement is based on the FS-2 design heritage and operational experience. Adequate adjustment and margin has been put into consideration based on orbit altitude difference between FS-2 and FS-5. The resultant pointing knowledge allocation is derived in Table 1 based on the geolocation requirements. 11)
Parameter | Pointing knowledge |
In-flight calibration residual | 0.0004º |
Thermal distortion between RSI Line-Of-Sight and STR reference | 0.010º |
Pointing knowledge requirement | 0.01697º |
RSI Line Of Sight wrt inertial reference frame (summation of three items above) | 0.03097º |
Pointing knowledge impact on geo-location (720 km altitude) | 390 m (3σ) |
Three major contributions for the pointing knowledge allocation are needed:
1) RSI (Remote Sensing Imager) Line-of-Sight orientation mis-knowledge with regard to the STR (Star Tracker ) reference frame after in-flight calibration. The given value is heritage from FS-2.
2) Orientation mis-knowledge between RSI LOS (Line-of-Sight) and the STR reference frame induced from thermal distortion in orbit. The value is also heritage from FS-2 and will be verified with analysis.
3) Pointing mis-knowledge of the STR alignment reference frame with regard to J2000 inertial reference frame. This item is used to derive the attitude pointing knowledge requirement in roll and pitch axes.
To meet the 0.012º ( 3σ) per axis, the absolute bus attitude knowledge requirement of the RSI for meeting its geolocation accuracy, the µASC (micro-Advanced Stellar Compass ) consisting of three CHUs (Camera Head Units) along with four single-axis fiber-optics gyros is mounted on a stable optical bench common to the RSI structure. The µASC is a highly advanced and fully autonomous star tracker produced by DTU (Technical University of Denmark). The gyroscopes considered in this mission are the fiber-optics rate sensors, µ-FORS6U, manufactured by Litef, a Northrop Grumman branch in Germany.
AOCS (Attitude Orbit and Control Subsystem): AOCS will be provided by a gyro-stellar attitude determination system, which utilizes attitude data provided by three CHUs and rate data provided by the four single-axis fiber-optics gyros. To account for the possibility of more than one gyro failure due to lack of space-flight heritage, a gyro-less attitude and rate determination algorithm is developed as a backup for the AOCS design.
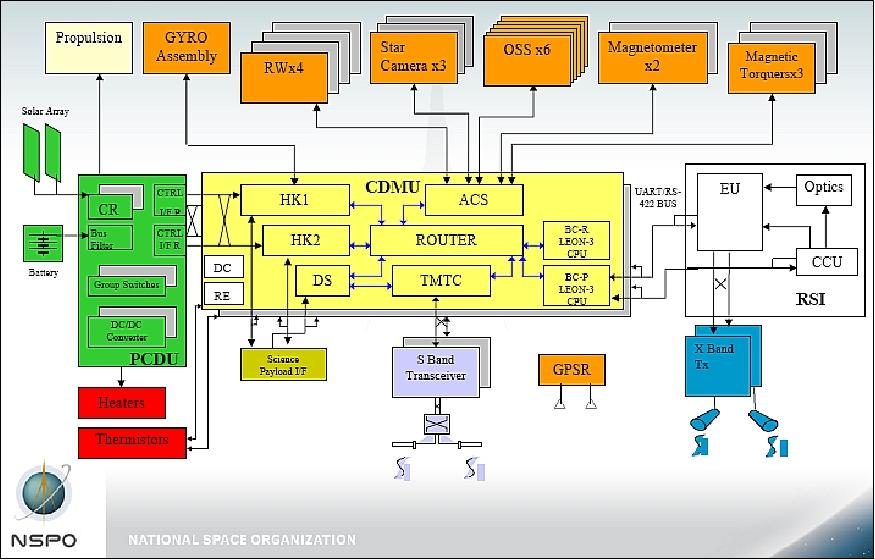
The CDMU (Command and Data Management Unit) is used for spacecraft control. It is composed of the following functional elements:
- OBC (Onboard Computer) is a Leon3-FT (Fault Tolerant ) processor implemented on an Actel AX-2000 FPGA. The processor is highly configurable, and includes functions such as the Floating Point Unit, three SpaceWire links, and a memory controller. The board also features UART (Universal Asynchronous Receiver/Transmitter) ports, CAN (Controller Area Network) bus controller and a clock system. The OBC itself also features the capability of board-level redundancy control of the other unit.
The LEON-3 CPU, developed by Gaisler Research, is a 32 bit synthesisable processor core based on the SPARC V8 architecture. It was chosen because of its advantages such as its high level of configurability and fault immunity, the wide availability of the source code, a deep pipeline and multi-processor support, and its suitability for the SOC (System-On-Chip) design through its implementation in a flight qualified FPGA (Field Programmable Gate Array).
- DS (Data Storage) module. Used to store satellite health telemetry and scientific data. DS has a memory capacity of 256 MB SDRAM with EDAC protection. Among other interfaces, it comprises also two SpaceWire nodes with CRC protection.
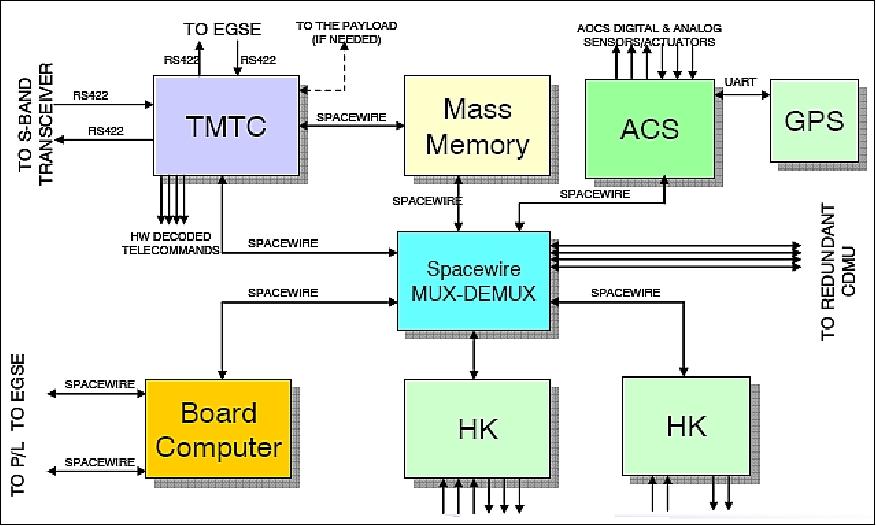
- Reconfiguration Electronics (RE) module. The objective is to manage the redundancy of the CDMU. The module monitors the major alarms of the CDMU; in case of a system irregularity, the module is able to reset the current CDMU, or switch over to the other CDMU, or employ a cross-strapping configuration.
- TMTC (Telemetry and Telecommand) module. The TMTC module encodes/decodes the telemetry/telecommand data according to the ESA packet telemetry standard. There are two
- Housekeeping (HK1, HK2) module. The objectives are to acquire the housekeeping data and to control satellite components. There are two identical modules in each CDMU. Each housekeeping module provides analog inputs and outputs, digital inputs and outputs, and serial interfaces. The BC communicates with two GPSR modules via housekeeping modules.
- AOCS (Attitude Orbit and Control Subsystem) module. The implementation includes the following interfaces: 2 star cameras, 2 magnetometers, 6 sun sensors, 4 reaction wheels, 3 magnetic torquers, and 2 pressure transducers.
- SpaceWire Mux-Demux (Multiplexer-Demultiplexer), developed by Carlo Gavazzi Space S.p.A., Italy. It features two master nodes connected to OBC-A and OBC-B, and 6 slave nodes connected to HK1, HK2, AOCS, DS, TMTC and one spare node. The data rate used for CDMU is 50Mbit/s.
- GPS receiver (GPSR) modules. Two GPS receiver modules are used in the CDMU. One GPS receiver is selected as the master and the second one as the backup receiver. Each GPSR module has its own PPS signal, however just one is selected. The PPS (pulse per second) signal shall be used for synchronization of the OBC clock which is distributed within the satellite.
- DC to DC power supply (DCDC1 & DCDC2) modules. The DCDC1 and DCDC2 convert the external unregulated 28V power to stable secondary powers for the CDMU modules. The DCDC1 module provides power to TMTC, SpaceWire MUX-DEMUX, RE and backplane modules. The DCDC2 module provides power to BC, DS, GPSR1, GPSR2, HK1, HK2, and AOCS modules.

EPS (Electrical Power Subsystem): The EPS is composed of a PCDU (Power Control Distribution Unit), a rechargeable battery, and two deployable solar generators. The PCDU is designed to handle over 150 FET switch outlet channels, converts the primary 28 V to the users demand secondary voltage, as well as regulates solar power input to the spacecraft. The FS-5 battery has a capacity of 24 Ah provided by small cells in a 8 series 16 parallel configuration. Each solar panel contains of 19 solar strings with 20 TJ GaAs solar cells in series. The solar generators can support FS5 mission in 280 W orbit average power.
The PCDU was domestically developed. The device contains seventeen circuitry modules with more than a hundred power distribution channels. Power is collected from 38 solar electricity control paths. The PCDU EM has been intensively tested on the satellite EDM (Engineering Development Module) along with the OBC EM and an early-released flight software build to verify the satellite command and telemetry contents. 12) 13)
The main functions of the PCDU are to condition energy from the solar arrays and distribute power for all subsystems on the satellite. The PR (Power Relay) module, one of the major modules in the PCDU, is to manage the switchover of redundant DC-DC converter sections, secondary power distribution and power protections. Each set of internal/external DC-DC converter section which is protected by an OC (Over Current) protection with LCL (Latch-up Current Limiter) is controlled by the PR module's FPGA. To avoid a short circuit in the DC-DC converter sections, the PR module provides UV (Under Voltage) protection to switch the nominal DC-DC converter to the redundant DC-DC section. In order to monitor the primary power voltage, an UVLO (Undervoltage-Lockout) detection circuit with majority voting in the FPGA is integrated in PR module. Figure 7 shows the main functional blocks of the PR module.
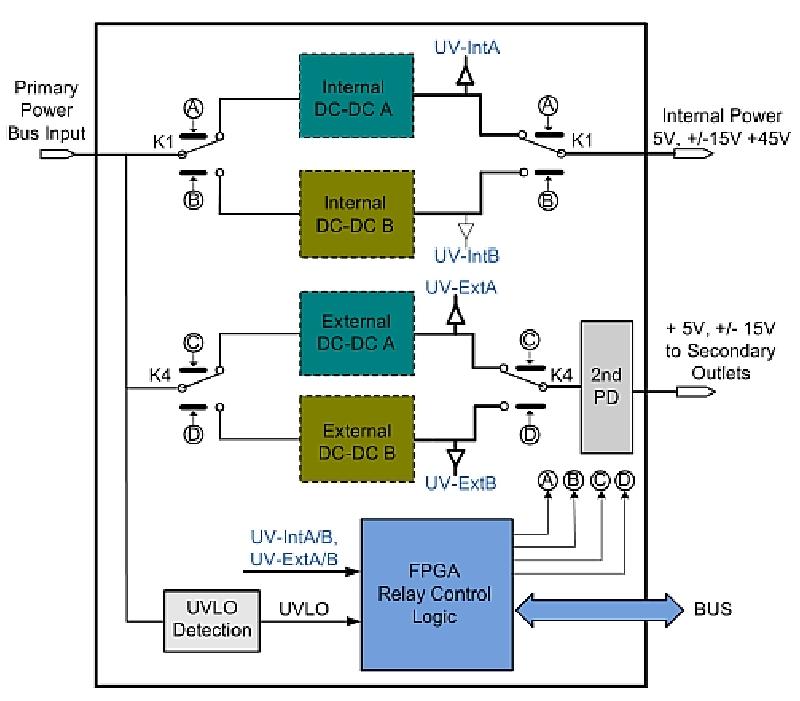

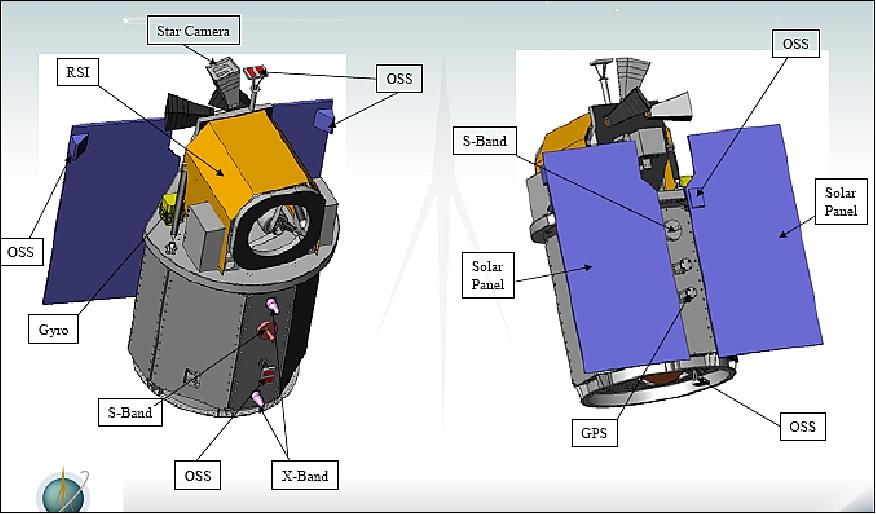
RF communications: The X-band is used for payload data downlink with a data rate of up to 150 Mbit/s. The TT&C functions are provided in S-band. An onboard image storage capability of 80 Gbit is provided. 14)
Key parameter | FormoSat-5 | FormoSat-2 |
Orbit, SSO (Sun Synchronous Orbit) | 720 km | 891 km |
Revisit time (capability) | Every other day | Daily |
LTDN (Local Time of Descending Node) | 9:45-10:15 hours | 9:30-10:30 hours |
Spacecraft design life | 5 years | 5 years |
Reliability | > 0.6 as a design goal at EOL | > 0.6 at EOL |
GSD (Ground Sample Distance) | 2 m (Pan), 4 m (MS) | 2 m (Pan), 8 m (MS) |
Swath width | 24 km | 24 km |
Spectral bands | 1 Pan + 4 MS | 1 Pan + 4 MS |
System CTF (Contrast Transfer Function), Pan | ≥ 0.1 | ≥ 0.12 |
SNR (Signal-to-Noise Ratio), Pan | ≥ 92 | ≥ 92 |
Duty cycle of RSI (Remote Sensing Imager) | ≥ 8% per orbit | ≥ 8% per orbit |
FOR (Field of Regard) | ±45º | ±45º |
Spacecraft agility | Roll : 24º/60 s | Roll : 60º/60 s |
Pointing accuracy | 0.1º per axis | 0.1º per axis |
Geo-accuracy of imagery | With GCP: 2 m | With GCP: 2 m |
On-board storage capacity | 80 Gbit | 45 Gbit |
Downlink availability for image data | ≥ 7 orbits/day | ≥ 7 orbits/day |
Spacecraft mass at launch | ~475 kg | 764 kg |
Development Status
• July 17, 2017: FormoSat-5, the first domestically developed satellite operated by Taiwan's NSPO (National Space Organization), was packaged and shipped to the U.S. over the past weekend for the launch by a SpaceX Falcon 9 rocket on August 24 at VAFB, in California - according to a report in the Taiwan News written by Teng Pei-ju. 15)
- This is the first remote sensing satellite designed, manufactured, and controlled by the NSPO and domestic teams and the satellite succeeds FORMOSAT-2, which was decommissioned in 2016. FORMOSAT-5 will embark on a five-year mission, orbiting the globe to conduct Earth Observation (EO) missions, according to the NSPO.
- Premier Lin Chuan visited the NSPO in Hsinchu on July 15 to oversee the final shipment preparation of the satellite. "We hope to push for the cooperation or expansion of the satellite-related industries and create opportunities for collaborations between Taiwan and other countries on manufacturing satellites," he said.
- The high-resolution images taken by FORMOSAT-5 will be able to contribute to state governance, national security, technological diplomacy, academic research, environmental monitoring, disaster prevention and relief, and international humanitarian relief, said Chen Liang-gee, the head of the Ministry of Science and Technology.
- Chang Guey-shin, director general of the NSPO, said that the satellite is due to arrive in the U.S. on July 20 where the spacecraft will undergo final testing prior to being integrated into Space X's Falcon 9 rocket on August 16 at Vandenberg Air Force Base in California.
- The launch of FORMOSAT-5 was originally scheduled for 2016 but was delayed due to an explosion of a Falcon 9 rocket in September, which disrupted the SpaceX launch schedules. Falcon 9 is a two-stage launch vehicle that can transport satellites and spacecraft into Earth orbit and was built by SpaceX, a private aerospace company established by Tesla founder Elon Musk in 2012 — Falcon 9 is the first rocket that is capable of re-use of the vehicle's first stage.
• March 3, 2017: Delays in SpaceX's launch schedule have led an aggregator of secondary payloads to find alternative rides for dozens of satellites it planned to fly on a Falcon 9. 16)
- In a March 2 message, Curt Blake, president of Seattle-based Spaceflight, said that "significant" delays in the planned launch of the Formosat-5 mission on a Falcon 9 from Vandenberg Air Force Base in California forced the company to find alternative rides for nearly 90 satellites that were to launch as secondary payloads on a payload adapter called Sherpa. "We learned our launch would occur potentially much later than expected," Blake wrote, not giving a specific launch date. "While delays are inevitable in the launch business, we made the decision to rebook all our customers slated to launch on the FormoSat-5 mission."

- Formosat-5 and Sherpa were scheduled to launch on a Falcon 9 last year (2016) but were delayed, in part because of the Sept. 1 pad explosion of a Falcon 9 during preparations for a static-fire test at Cape Canaveral. That halted all Falcon 9 launches for four and a half months.
- Blake, in an October 2016 interview, said Spaceflight was waiting on the Falcon 9 return to flight before getting a new launch date, and at the time didn't expect a launch before early 2017. The company had told the owners of the satellites not to ship them to Spaceflight for integration onto the Sherpa adapter until it got a confirmed launch date from SpaceX.
- SpaceX has not disclosed a launch date for Formosat-5, or many other upcoming launches from Vandenberg. SpaceX President Gwynne Shotwell, speaking at a Feb. 17 press conference at NASA's Kennedy Space Center, said the company had delayed the next Falcon 9 launch of Iridium satellites, from Vandenberg, from mid-April to mid-June "to fill in the queue of folks that have been waiting for a flight since we were down last September." The company hasn't identified those customers.
- Blake said that, given the extended delays, the company decided to find other rides for the satellites that were to fly on Sherpa. "It took a huge effort, but within two weeks, the team hustled to have all customers who wanted to be rebooked confirmed on other launches!" he wrote.
- Spaceflight spokeswoman Jodi Sorensen said March 2 that most of the satellites that had been flying on Sherpa will be rebooked on one of two launches. One is on the company's own dedicated Falcon 9 mission, dubbed SSO-A, scheduled to launch from Vandenberg later this year. The other is an unspecified "international launch" scheduled for this summer or fall.
- Spaceflight has brokered launches of small satellites as secondary payloads on a number of different vehicles. That included nine cubesats that launched on an Indian Polar Satellite Launch Vehicle (PSLV) Feb. 14. Eight of the nine satellites were from Spire Global, a company deploying a constellation of cubesats for ship tracking and weather data. The ninth satellite was a cubesat from an Israeli university.
• Oct. 16, 2015: According to NSPO, FormoSat-5 has passed space environment and function tests and is set to take over duties from FormoSat-2, which has been in orbit since 2004. 17)
• May 12, 2015: NSPO announced that the FormoSat-5 primary payload, a CMOS-type Remote Sensing Instrument (RSI), has been successfully developed, integrated to the spacecraft, and tested to its operational requirements in April 2015. 18)
• January 29, 2015: The AIP (Advanced Ionospheric Probe), developed by the National Central University (NCU), was delivered and is being integrated into the spacecraft. 19) 20)
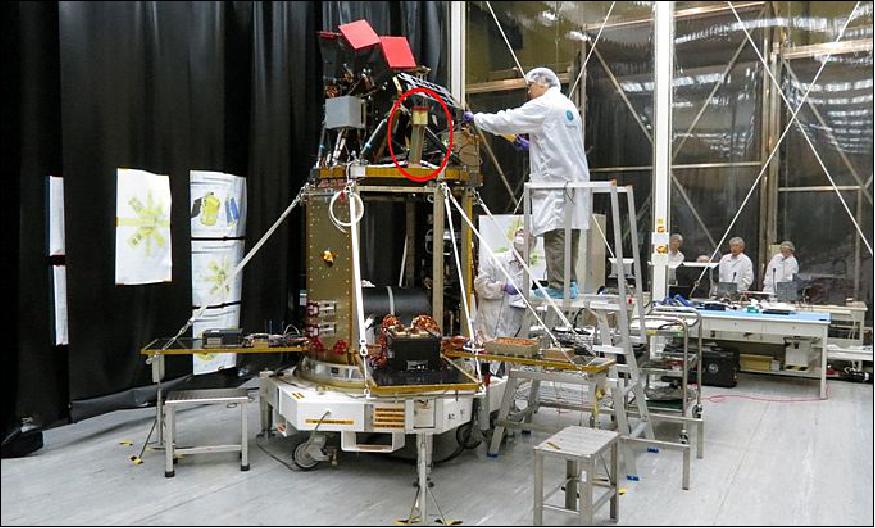
• The program officially has been in the phase of integration and test. FORMOSAT-5 program has passed all design reviews, successfully integrated and tested 85% of the satellite components together and is finishing up the primary payload, the Remote Sensing Instrument (RSI). All subsystems (except RSI) have passed Comprehensive Performance Test #2 on Engineering Development Model (EDM). 21)
Launch
The FormoSat-5 spacecraft of NSPO was launched on August 24, 2017 [18:51 GMT, or at 11:51 a.m. PDT (Pacific Daylight Time), corresponding to 2:51 hours Taiwan Time on August 25] — on a Falcon-9 v1.2 (Full Thrust) vehicle of SpaceX. The launch site was VAFB (Vandenberg Air Force Base), CA, SLC-4E (Space Launch Complex-4E, Ref. 15). 22) 23) 24) 25) 26) 27)
A launch contract between NSPO and SpaceX was signed in June 2010. 28) 29)
Orbit of primary payload: Sun-synchronous near-circular orbit of FormoSat-5, altitude = 720 km, inclination = 98.28º, period = 99.19 minutes, LTDN (Local Time on Descending Node) at ~ 10 hours.
Some post launch events:
• Following stage separation, Falcon 9's first stage successfully landed on the "Just Read the Instructions" droneship stationed in the Pacific Ocean.
• The first stage of Falcon 9 was separated at 2 minutes 35 seconds after launch at an altitude of 90 km. The fairing was ejected 24 seconds later at an altitude of 132 km. At 11 minutes 19 seconds after launch, FormoSat-5 was deployed into mission orbit. The teams in Taiwan and in the United States congratulated each other excitedly for FormoSat-5 launched into space successfully.
• NSPO received the state vector transmitted from SpaceX and used it to propagate FormoSat-5's orbit. The orbit data was sent to the Svalbard station to prepare for the first pass. At 82 minutes and 56 seconds after launch, the ground antenna of Svalbard station received the signal from FormoSat-5 as expected. The success of the first contact marks a milestone of FormoSat-5.
• During the 5th orbit of FormoSat-5, the Chungli ground station in Taiwan communicated with the FormoSat-5 spacecraft successfully. Minister Liang-Gee Chen, watching the live broadcast from his MOST office, delivered his appreciation to Narlabs and NSPO immediately (Ref. 22).
Mission Status
• The FormoSat-5 minisatellite and its payload are operating nominally in March 2018. The FormoSat-5 Image Processing Team is finalizing its processing software system now in order to get ready for an official operation in few months. 30)
Sensor Complement
RSI (Remote Sensing Imager)
RSI, the primary payload, is being developed domestically by a team including NSPO institutions and commercial partners: ITRC (Instrument Technology Research Center), CIC (National Chip Implementation Center), CSIST (Chung-Shan Institute of Science and Technology), AIDC (Aerospace Industrial Development Corporation), CIS (CMOS Sensor Inc.), and CAMELS Vision Technologies.
NSPO/ITRC/CIC of NARL (National Applied Research Laboratories) coordinate the domestic multi-discipline capabilities to collaborate for developing the fully made-in-Taiwan (MIT) RSI payload and establishing the background for the future higher performance RSI instrumentation. 31) 32) 33) 34) 35)
The objective is to establish capabilities of system design, integration & test, and opto-mechanical system calibration. The goal is to develop a self-reliant infrastructure in all key technologies of the project. The imaging objectives are to provide a 2 m GSD (Ground Sample Distance) for Pan imagery and 4 m GSD for multispectral imagery on a swath of 24 km.
The imagery of RSI will be used for such applications as: planning tool for urban development, environment and crop health monitoring, disaster assessment, etc.
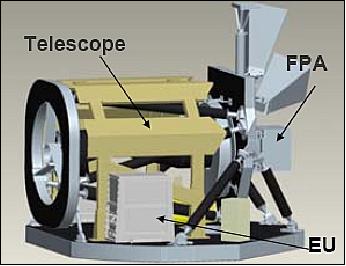
RSI instrument type | Pushbroom imager |
Spectral bands | 1 Pan |
GSD (Ground Sample Distance) | 2 m (Pan), 4 m (MS) |
Swath width | 24 km |
Data quantization | 12 bit |
CTF (Contrast Transfer Function) | ≥ 0.1 |
SNR (Signal-to-Noise Ratio) | ≥ 92 (Pan), ≥ 100 (MS) |
Detector type | TDI CMOS array, 5 bands, Pan =12,000 pixels, MS = 6000 pixels |
Optics | Cassegrain telescope with an aperture of 45 cm (primary mirror) |
The RSI instrument consists of a Cassegrain telescope and an EU (Electronic Unit). The telescope is composed of the primary mirror (entrance pupil diameter = 45cm), secondary mirror, correct lens, baffles, CFRP (Carbon Fiber Reinforced Plastic) structure, and FPA (Focal Plan Assembly ). The FPA is the most crucial component in terms of its technology and performance for space applications (Ref. 5).
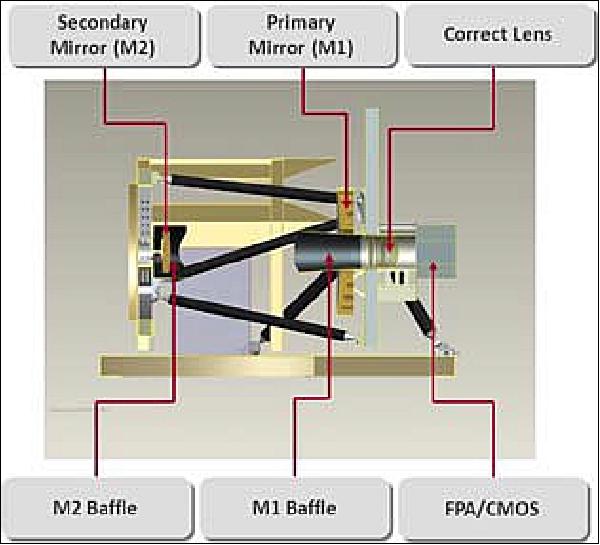
The FPA is composed of the image sensor, bandpass filter, control electronics, and the housing structure. Detector arrays with CMOS (Complementary Metal Oxide Semiconductor) technology are used. The Pan detector is composed of 12,000 pixels with 10 µm/pixel and the MS image sensor is composed of 6,000 pixels with 20 µm per pixel, respectively, that provide the spatial resolutions of 2 m (Pan) and 4 m (MS) in a swath of 24 km. The detector array chip of CIS (CMOS Sensor Inc.) has a size of 12 cm x 2.4 cm. This advanced detector array has been designed by CIS and fabricated on an 8" wafer with 0.18 µm process manufactured by UMC (United Microelectronics Corporation) in Taiwan.
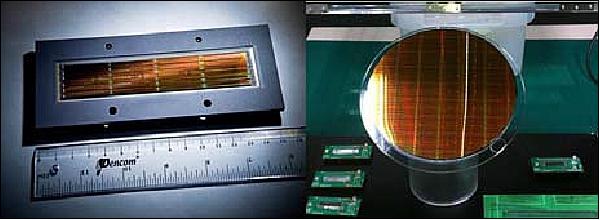
A 5-band filter has been designed and developed in-house, meeting (actually exceeding) all performance requirements for RSI integration (Figure 15).

The acquisition of on-board image data compression techniques represents another achievement for this program. Collaboration between NSPO and its vendor has mastered the CCSDS format through applying DWT (Discrete Wavelet Transfer) and BPE (Bit Plane Encoder) techniques to compress the very high volume imagery in real time.
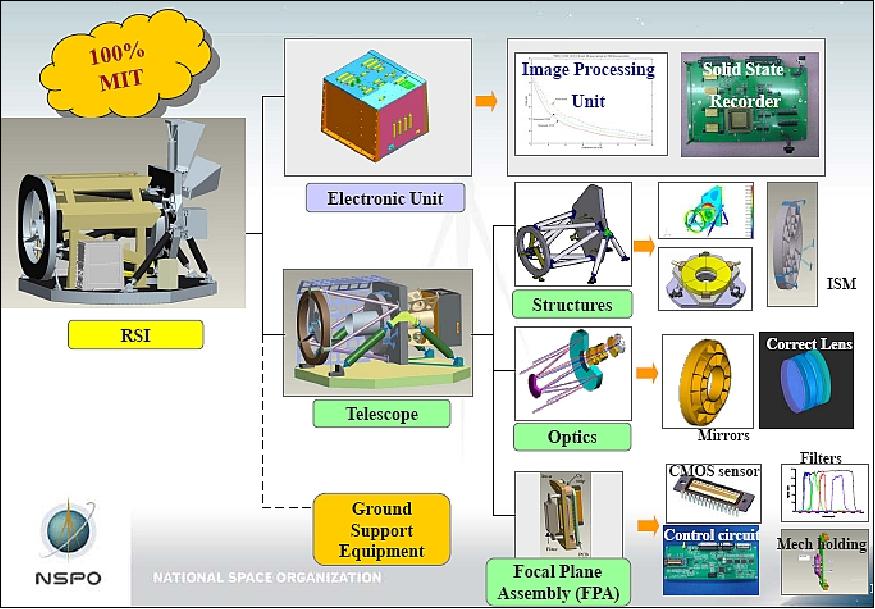
On June 11, 2012, the FormoSat-5 program held the AIT (Assembly, Integration, and Test) kick off meeting at NSPO for the RSI (Remote Sensing Instrument). 36)
AIP (Advanced Ionospheric Probe)
The AIP instrument is designed and developed at NCU (National Central University), Jhongli City, Taiwan. It is an all-in-one plasma sensor with a sampling rate up to 8,192 Hz to measure ionospheric plasma concentrations, velocities, temperatures, and ambient magnetic fields over a wide range of spatial scales. With the comprehensive dataset from the AIP observations, the project will be able to conduct a systematic examination of the plasma parameters in the topside F region of the ionosphere, with regard to longitudinal and latitudinal distributions and seasonal variations. The collected data will not only be used for space science studies, but can also be shared by the scientific community in seismic precursors associated with strong earthquakes and co-seismic effects.
The AIP instrument is being developed through a collaborative effort between scientists of ISS (Institute of Space Science) and engineers of IOM (Institute of Opto-Mechatronics) at NCU, led by Chien-Ming Huang. These two teams have participated in many space payload missions, funded by NSPO, and in international collaborations, providing the scientific payloads. The payloads obtained their flight heritage on sounding rocket flight and on spacecraft throughout the last decade.
Note: In the early stage of the program planning, the two instruments MFI (Magnetic Field Instrument) and MDRP (Marine Data Relay Payload) were among the scientific payload candidates for the FormoSat-5 mission. However, since the later part of 2011, AIP (Advanced Ionospheric Probe) has become the only scientific payload on board FormoSat-5. 37)
The AIP instrument features measurement modes like a PLP (Planar Langmuir Probe), RPA (Retarding Potential Analyzer), an IT (Ion Trap), and an IDM (Ion Drift Meter) to obtain the characteristics of the ionospheric plasma concentration (Ni), velocities (Vi), and temperatures (Ti and Te). 38)
1) PLP: When AIP operates in PLP mode, the PLP electrode (shown on the left of Figure 17) is isolated with aperture plane and applied to a sweeping voltage between -10 V and +10 V. Once the sweeping voltage is lower than the plasma potential, the electrode repels electrons and accelerates ions. As the voltage decreases, the electron current decreases rapidly. The electron temperature (Te) can be determined by the slope of the I-V curve by the following equation:

where I is the current through the PLP electrode, V the voltage applied to PLP electrode, κ is the Boltzmann constant, and e is the electric charge. As the slope of the I-V curve is flat, the Te is higher. As the slope is steep, the Te is lower.
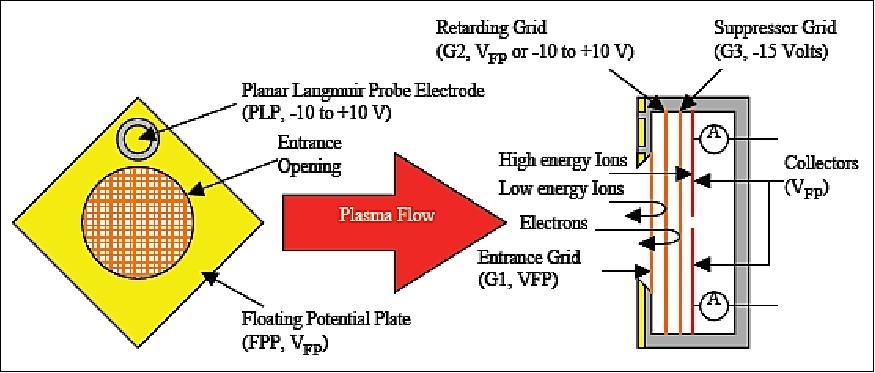
2) RPA and IT: AIP consists of three metal grids to handle the incoming plasma (Figure 17). The first grid, G1, is an aperture grid arranged in the front of the probe and connected to a floating potential device to allow a smooth entry of the incoming plasma into the probe, not to be affected by the transverse electric field. The second grid, G2, is a retarding grid connected to a programmable sweeping voltage circuit between -10 and 10 V. If AIP operates in the IT mode, the grid is maintained at the floating potential without repelling the incoming ions. In the RPA mode, the grid forms a potential barrier (retarding voltage) to block the low energy positive ions into the probe. The third grid, G3, is a suppressor grid, maintained at -15 V to repel the incoming electrons into a quadrant metal collector; it can also reduce the photoelectrons escaped from the collector when the sunrays strike on the collector. All four metal plates of the quadrant collector are maintained at the floating potential and are connected together to an ammeter via an analog switch in these two modes.
As the retarding voltage increases, the positive ions with low kinetic energy are repelled out of the probe or neutralized by the grids and interior boundary of the probe. The positive ions with high kinetic energy can penetrate through the retarding grid to the collector if they do not contact with the suppressor grid or the interior boundary of the probe. Basically the higher the retarding voltage, the lower the current is measured at the collector. Such a sampling process can be recorded as I-V curves that are embedded with information of ion temperature, composition and ram velocity. A 1-dimensional ion current equation for this application has been written down in the following form:

where I is the current measured at the collector, q is the electric charge, A is the effective collection area of the collector, n is the ion concentration, U is the ion ram velocity,M is the mass of the ion, κ is the Boltzmann constant, Ti is the ion temperature, Vr is the maximum electric potential along the path for plasma from outside environment to the probe (it is usually the retarding voltage). It should be noted that the electric potential is referenced to far-away plasma (the electric potential of the far-away plasma is zero).
The initial ion temperature and the concentrations can be deduced from the I-V curves via a half-current approximation. To improve the quality of the output parameters, an iterated half-current method is prepared for initial guesses and a grid search method is used to refine the output parameters with possible uncertainties. To derive precise ion temperature and ram velocity, a numerical model to estimate the effects of the grid alignment and electric potential depression on the grids is required.
3) IDM: In IDM mode, the G1 and G2 grids are maintained to the floating potential. However, each metal plate of the quadrant collector is connected to an individual current meter (ammeter). The arrival angle of the incoming ions can be estimated from the current differences of the adjacent ammeters (Figure 18). The possible current ratio can be estimated by the following relation:
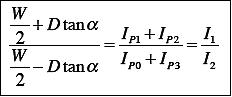
where W is the width of the aperture, D is the depth between the aperture and the collector. The incident angle, , of the incoming ion can be expressed as:


4) Geophysical Parameters: In default mode, the AIP is set to measure the ionospheric plasma in a cycle of PLP, RPA, IT, and IDM mode sequentially. It is assumed that all ionospheric parameters have a slow variation within a 4-second period. After one cycle is complete, the geophysical parameters, Ni, Vi, Ti, and Te, can be derived from the steps shown in Figure 19.
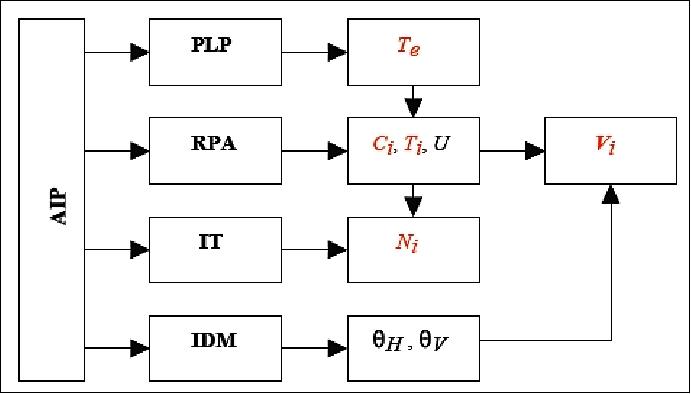
Vi can be obtained by the arrival angles,θH and θV, from the IDM mode and U from the RPA mode. Ni can be obtained by ion flux from IT mode and U from the RPA mode. Ti and U can be obtained by the I-V curve from the RPA mode, and Te from the PLP mode.
AIP instrument: The AIP consists of two main units, a sensor unit located on the top panel of the satellite and a SPEU (Science Payload Electronics Unit) inside the satellite. The sensor unit has a sensor head mounted on a stand to enlarge the FOV (Field of View). The SPEU hosts the controllers and the DC/DC converters to control the sensor unit and interface with the CDMU (Command and Data Management Unit) of the satellite bus.
Dimension | 100 mm (L) x 100 mm (W) x 100 mm (H) for the sensor head |
Mass | 0.582 kg for the sensor head |
Materials | Al alloy 6061-T6 for the sensor head |
The sensor head is illustrated in the Figure 20 and its three-grid configuration is shown in Figure 21. The FOV, with the angle centered velocity vector (sensor look direction) and originating at the perimeter edge of the AIP aperture plane, is about 56º. The opening of the sensor is 50 mm. All the grids are made of stainless steel 316, coated with gold, with 50 lines per inch in grid density and 0.0045 inch in wire diameter.
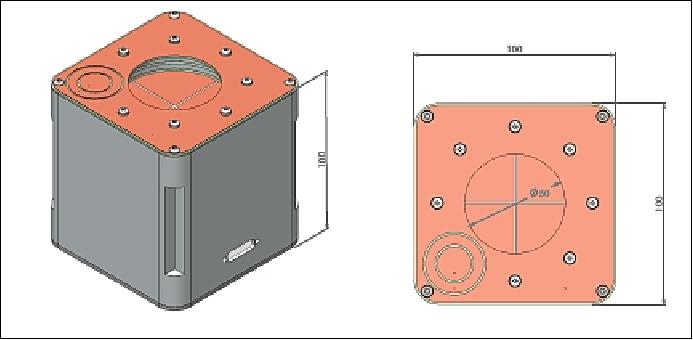
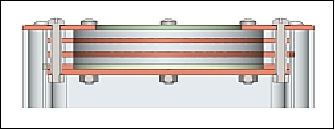
Electrical specification: The functional diagram of the AIP is shown in the Figure 22. Dual CDMUs and a PCDU (Power Control Distribution Unit) with dual power channels are interfaced with the SPEU. A redundant design is provided for the controllers, DC/DC converters, A/D converters, and D/A converters; the ammeters are implemented to reduce a single point failure of the system. Since there are no hardwires from the PCDU to activate the primary or redundant controller, the PCDU can provide power to the primary/redundant DC/DC converter to startup the primary/redundant controller. However, there is only one controller on at a time. It is forbidden to provide power to the primary and the redundant DC/DC converters simultaneously. This would cause a cross-strapping malfunction of the RS-422 interfaces to the CDMU and digital lines to the sensor.

Input voltage | 28±6 VDC |
Average power | 2.24 W for sensor, ~2.5 for SPEU |
Command and telemetry interface | Dual channels, RS-422 serial asynchronous transmission with a baud rate of 19.2 kbit/s |
Science data interface | Dual channels, RS-422 serial synchronous transmission with a baud rate of 1 Mbit/s |
In the command and telemetry interface, 3-byte commands and 64-byte ancillary data are designed to deliver from the CDMU to the SPEU. The 19-byte status of health information of the AIP is delivered to from the SPEU to the CDMU.
In the science data interface, a science data packet with a fixed length of 1024 bytes can be delivered from the SPEU to the CDMU. For the normal/fast/burst mode, the SPEU can deliver 1/8/64 science data packet(s) every 3 seconds.
Ion composition (light ions and O+) | Range: 3% to 100% |
Total ion concentration | Range: 4 x 102 to 1.2 x 107 # cm-3 |
Ion velocity | Range: ±4 km/s for cross track direction, ±5 km/s for ram direction |
Ion temperature | Range: 500 to 10,000 K |
Electron temperature | Range: 500 to 10,000 K |

Ground Segment
Ground segment, which includes ground operation facility and image processing system, is being upgraded to meeting FormoSat-5 requirements. Both hardware and software upgrades are being conducted on top of the existing operational segment by NSPO team in house with minimum external supports. 39)
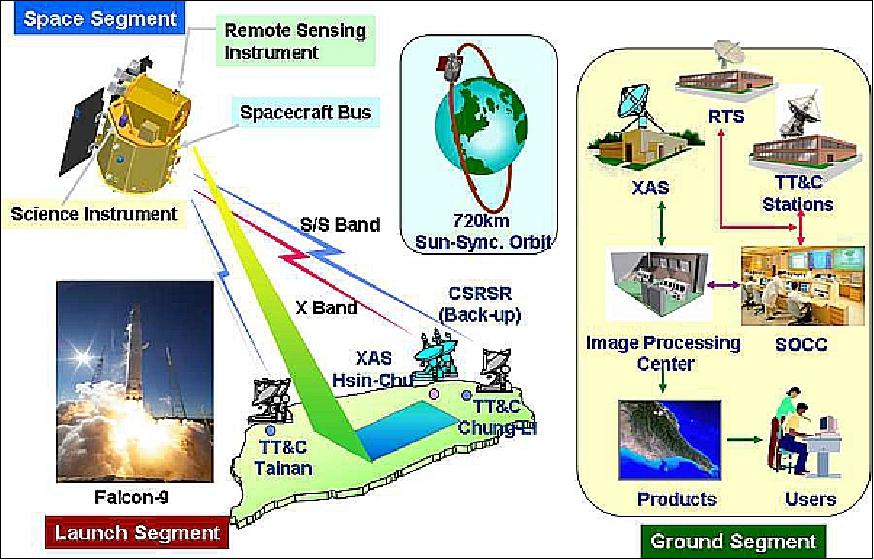
References
1) H. P. Chang, "FormoSat-5 Program," NSPO, Dec. 9, 2010, URL: http://www.csrsr.ncu.edu.tw/08CS
RWeb/ChinVer/C7Info/2010RSDMM/PPTpdf/1.pdf
2) Albert Lin, Chien-Fang Lai, "The Command and Data Management Unit for NSPO FormoSat-5 and Future Satellites," 4th Asian Space Conference (ASC-2008), Taipei, Taiwan, October 1-3, 2008, URL: http://project2049.net/documents/revolutionizing_taiwans
_security_leveraging_c4isr_for_traditional_and_non_traditional_challenges.pdf
3) Jeng-Shing Chern, Jer Ling, Shui-Lin Weng, "Taiwan's second remote sensing satellite," Acta Astronautica, Vol. 63, Issues 11-12, December 2008, pp. 1305-1311
4) Bang-Ji Wang, Chia-Ray Chen, Jih-Run Tsai, Fei-Hon Chou, "Remote Sensing Instrument Development in Taiwan," 4th Asian Space Conference (ASC-2008), Taipei, Taiwan, October 1-3, 2008
5) Guey-Shin Chang, An-Ming Wu, Ho-Pen Chang, "A Perspective on Taiwan's Earth Observation Missions," Proceedings of the 63rd IAC (International Astronautical Congress), Naples, Italy, Oct. 1-5, 2012, paper: IAC-12-B1.2.6
6) Lou-Chuang Lee, "Space Programs in Taiwan - FORMOSAT 5 and FORMOSAT 7," 4th International Conference on Particle and Fundamental Physics in Space (SpacePart12)," CERN, Geneva, Switzerland, Nov. 5-7, 2012, URL: http://indico.cern.ch/getFile.py/access?contribId=15
&sessionId=2&resId=1&materialId=slides&confId=197799
7) "FormoSat-5," NARLabs (National Applied Research Laboratories) of NSPO, 2014, URL: http://www.nspo.narl.org.tw/2011/tw/download/info/DM_E_2014.pdf
8) Ho-Pen Chang, Guey-Shin Chang, Jer Ling, Tony Tsai, "Remote Sensing Satellite FORMOSAT-5," Proceedings of the 63rd IAC (International Astronautical Congress), Naples, Italy, Oct. 1-5, 2012, paper: IAC-12-B4.4.8
9) Jeng-Shing Chern, Jer Ling, Shui-Lin Weng, "Taiwan's second remote sensing satellite," Acta Astronautica, Vol. 63, Issues 11-12, December 2008, pp. 1305-1311
10) "FORMOSAT-5 Component Environmental Specification," NSPO, FS5-SPEC-0003, Issue 01 Revision 00, April 21, 2010, URL: http://www.narl.org.tw/ext/po/po_attach.php?po_file_id=14606
11) Hao-Chi Chang, Peter Chiang, Yeong-Wei Adny Wu, Tom Lin, "Spacecraft attitude and rate determination using numerical gyros," Proceedings of the 65th International Astronautical Congress (IAC 2014), Toronto, Canada, Sept. 29-Oct. 3, 2014, paper: IAC-14-D9.2.8
12) Che-Cheng Huang, Jia-Jing Yeh, Zhe-Yang Huang, Chien-Kai Tseng, "FormoSat-5 Satellite Power Protection Design," Applied Mechanics and Materials,Vol. 145, 2012, pp. 536-541
13) http://www.nspo.org.tw/2008e/projects/project5/component.htm
14) I-Young Tarn, "On the Antenna Gain Requirement Derivation and Antenna Orientation Arrangement for Satellite Remote-Sensing Data Downlink," Proceedings of the 4th Asian Space Conference (ASC2008) and the FORMOSAT-3/COSMIC Data User Workshop, Taipei, Taiwan, Oct. 1-3, 2008
15) "Taiwan - FORMOSAT-5 Ships to Vandenberg AFB for SpaceX Launch," Satnews Daily, July 17, 2017, URL: http://www.satnews.com/story.php?number=1572156151
16) Jeff Foust, "SpaceX delays force Spaceflight to find alternative launches," Space News, March 3, 2017, URL: http://spacenews.com/spacex-delays-force-spaceflight-to-find-alternative-launches/
17) "FormoSat-5 slated for February 2016 launch," Taiwan Today, Oct. 16, 2015, URL: http://www.taiwantoday.tw/ct.asp?xItem=237856&ctNode=2194&mp=9
18) "FORMOSAT-5 CMOS RSI Delivery," NSPO, May 12, 2015, URL: http://www.nspo.org.tw/2008e/news/news_content.php?id=000554&hid=ne3qNn6Xvm
19) "AIP Science Payload on-board FORMOSAT-5," NSPO, Jan. 29, 2015: URL: http://www.nspo.org.tw/2008e/news/news_content.php?id=000551&hid=eBWIYp5hSc
20) "Advanced Ionospheric Probe (AIP) Science Payload on-board FORMOSAT-5," NARLabs, January 27, 2015, URL: http://www.narlabs.org.tw/en/news/news.php?news_id=559
21) "FORMOSAT-5 Status," NPSO, URL: http://www.nspo.org.tw/2008e/projects/project5/research.htm
22) "FORMOSAT-5 Was Launched to Space Successfully And Has Contacted with Taiwan Ground Station," NARLabs/NSPO, August 25, 2017, URL: http://www.nspo.narl.org.tw/en2016/info/news.shtml?id=000603&hid=NQcb9gz9Tb
23) "SpaceX's Falcon 9's Round Trip Launch and Land ... Sends FORMOSAT-5 Satellite Skyward," Satnews Daily, Aug. 24, 2017, URL: http://www.satnews.com/story.php?number=1403183138
24) "Launch Announcement for FORMOSAT-5," NARLabs, NSPO, Latest News, July 11, 2017, URL: http://www.nspo.narl.org.tw/en2016/info/news.shtml?id=000594&hid=peHhRcY2MN
25) "FORMOSAT-5," Wikipedia, URL: https://en.wikipedia.org/wiki/FORMOSAT-5
26) "FormoSat-5," NSPO, URL: http://www.nspo.org.tw/2008e/projects/project5/intro.htm
27) "Taiwan Preparing Satellite Launch," Defense News, Aug. 14, 2015, URL: http://www.defensenews.com/story/defense/air-space/space
/2015/08/14/taiwan-preparing-satellite-launch/31706197/
28) "SpaceX And NSPO Sign Contract To Launch Earth Observation Satellite," Space Daily, June 17, 2010, URL: http://www.spacedaily.com/reports/SpaceX_And_NSPO_Sign
_Contract_To_Launch_Earth_Observation_Satellite_999.html
29) Peter B. de Selding, "SpaceX Falcon 1e To Launch Taiwan's FormoSat-5 Craft," Space News, June 15, 2010, URL: http://www.spacenews.com/contracts/taiwan-
orders-spacex-falcon-launch-for-formosat-5.html
30) Information provided by Ho-Pen Chang, FormoSat-5 Program Manager at NSPO.
31) Jer Ling, "Applications of CMOS Sensors on FORMOSAT-5 Remote Sensing Instrument," Workshop on CMOS Applications in Astronomy and Space Sciences, National Tsing Hua University, Hsinchu, Taiwan, Jan. 5-6, 2011, URL: https://web.archive.org/web/20211230013611/http://crab0.astr.nthu.edu.tw/CMOS-workshop-2011/0105/0105_1420-1510_JLing_Applications-of-CMOS-Sensors-on-FORMOSAT5-RSI.pdf
32) "Remote Sensing Instrument Made Domestically - NSPO Spreads the Wings to Fly Again," NSPO, Feb. 2, 2010, URL: http://www.nspo.org.tw/2008e/news/news_
content.php?id=000301&hid=UWjqL7ZqeF
33) http://www.nspo.org.tw/2008e/projects/project5/instrument.htm
34) Wen-Chih Hsu, Jer Ling, Wei-Chun Chen, Ming-Hsien Tsai, Shih-Hung, "The Radiation Qualification of the Taiwanese CMOS Image Sensor for the Remote Sensing Satellite," 8th International Hiroshima Symposium of the Development and Application of Semiconductor Tracking Detectors. Taipei, Taiwan, Dec. 8, 2011, URL: http://indico.cern.ch/getFile.py/access?contribId=71&sessi
onId=9&resId=1&materialId=slides&confId=135986
35) Ching-Wei Chen, Po-Hsuan Huang, Chia-Ray Chen, Chia-Yen Chan, Chun-Chieh Lien, Po-Han Huang, Ming-Ying Hsu, Shenq-Tsong Chang, Ting-Ming Huang, "Integration, alignment, and verification of optical system assembly for FORMOSAT-5 ", Proceedings of. SPIE, Vol. 9195, "Optical System Alignment, Tolerancing, and Verification, VIII," 91950H (September 8, 2014); doi:10.1117/12.2060604;
36) "FORMOSAT-5 kicked off Remote Sensing Instrument AIT Tasks," NSPO, June 12, 2012, URL: http://www.nspo.org.tw/2008e/news/news_content.php?id=000449&hid=RnTiAiDVyQ
37) Information provided by Ho-Pen Chang, FormoSat-5 Program Director of NSPO.
38) Chi-Kuang Chao, Chien-Ming Huang, Yen-Hsyang Chu, Ching-Lun Su, Jann-Yenq Liu, Shigeyuki Minami, "Advanced Ionospheric Probe onboard the FORMOSAT-5 Satellite," Proceedings of the UN/Japan Workshop and The 4th Nanosatellite Symposium, Nagoya, Japan, Oct. 10-13, 2012, URL: http://unisec.jp/nanosat_symposium/4th/pdf/Day2_3_S-1_08_Chi-Kuang_Chao/S-1_08_Chi-Kuang_Chao.pdf
39) http://www.nspo.org.tw/2008e/projects/project5/intro.htm
The information compiled and edited in this article was provided by Herbert J. Kramer from his documentation of: "Observation of the Earth and Its Environment: Survey of Missions and Sensors" (Springer Verlag) as well as many other sources after the publication of the 4th edition in 2002. - Comments and corrections to this article are always welcome for further updates (eoportal@symbios.space).
Spacecraft Launch Mission Status Sensor Complement Ground Segment References Back to Top