ITASat-1 (Instituto Tecnológico de Aeronáutica Satellite-1)
EO
Operational (nominal)
AEB
ITASat-1 (Instituto Tecnológico de Aeronáutica Satellite-1) is a university-led, technological 6U CubeSat mission, funded by the Brazilian Space Agency (AEB) and launched in December 2018 on a SpaceX rideshare mission. As a joint collaboration between the Technological Institute of Aeronautics (ITA) and the National Institute of Space Research (INPE), the development process of ITASat-1 allowed Brazilian students to gain experience working with space technology, while the satellite itself has enabled in-orbit testing of new components and technologies for future Brazilian missions. It is still operational, being operated by the ITA ground team in São José dos Campos.
Quick facts
Overview
Mission type | EO |
Agency | AEB |
Mission status | Operational (nominal) |
Launch date | 03 Dec 2018 |
CEOS EO Handbook | See ITASat-1 (Instituto Tecnológico de Aeronáutica Satellite-1) summary |
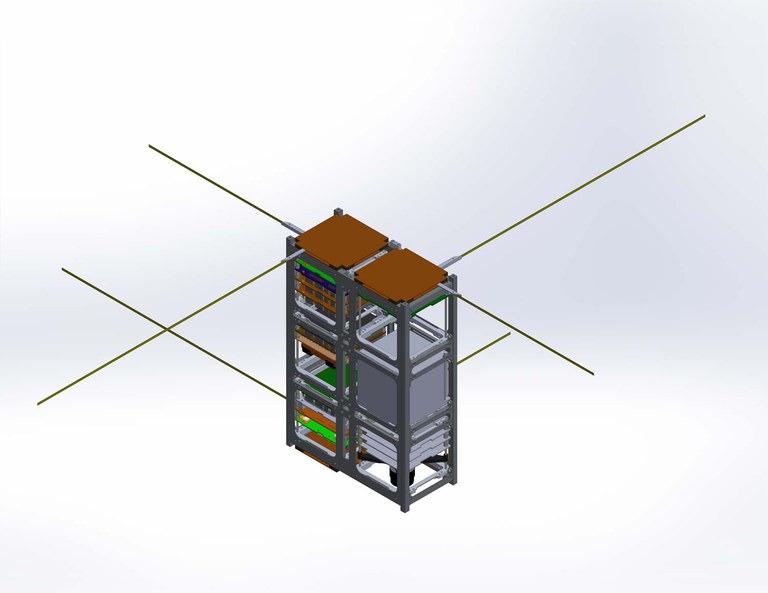
Summary
Mission Capabilities
ITASat-1 is equipped with a Data Collection System (DCS) transponder, a GPS (Global Positioning System) receiver, a camera named ‘Cam’ and the DCX-2 (Communication Experiment-2).
The DCS transponder was developed as a miniature version in order to fit in a CubeSat in terms of size and power consumption. Since it is compatible with the existing Brazilian Data Collection System, this transponder can collect data from more than 900 Data Collection Platforms, spread over the Brazilian territory and over coastal areas, offering insight into the water quality, ocean levels, air composition and animal migration. Cam is a commercial NanoCam C1U camera, operating in the visible range of the spectrum with the main objective to prove the attitude control system performance. DCX-2 was proposed by the Brazilian Amateur Community as an experiment of providing a communication channel with amateur radios over the globe. The experiment has three modes of operation (‘Beacon’, ‘Transponder’, ‘Store and Forward’), allowing for different types of messages (UHF/VHF: Ultra High Frequency and Very High Frequency, respectively) to be transmitted to various receivers.
Performance Specifications
Cam is a three megapixel colour sensor with a 35 mm lens. It has a performance of 60 m per pixel at 650 km altitude. In terms of image acquisition, the 4:3 colour CMOS sensor provides an image with a resolution of 2048 pixels x 1536 pixels in a 10-bit RGB Bayer pattern.
ITASat-1 maintains a sun-synchronous circular orbit at an altitude of 575 km and an inclination of 98°, with its LTDN (Local Time of Descending Node) being 10:30 hours.
Space and Hardware Components
ITASat-1 has a CubeSat architecture with 6U shape and a weight of 5.2 kg. The 6U CubeSat bus features two OBCs (On-Board Computers): one dedicated to the On Board Data Handling Subsystem (OBDH) and the other one designed for the Attitude Determination and Control Subsystem (ADCS). The OBDH is composed by a commercial, high-performance ARM-Cortex M3 based computer, running on a Free Real Time embedded Operating System. The ADCS computer is based on an ARM 7 MCU, which connects directly to the sensors (Sun sensor, gyrometer and magnetometer) and acts on the actuators (magneto-torquers and reaction wheels).
The spacecraft includes a Telemetry and Telecommand Subsystem (TMTC), a Structure and Thermal Subsystem (STS), as well as an Energy and Power Supply Subsystem (EPS). Solar panels are mounted onto five sides of the CubeSat, generating 6-7 W of power in total. The sixth side hosts the camera. The energy is stored in Lithium-ion batteries.
ITASat-1 (Instituto Tecnológico de Aeronáutica Satellite-1)
Spacecraft Sensor Complement Launch Payloads LEOP Mission Status References
ITASat-1 is the first university and technological microsatellite of Brazil, funded by AEB (Agência Espacial Brlaunchasileira - Brazilian Space Agency) in the framework of the Small Technological Satellite Development and Launching Program. The development and operation of ITASat-1 is a great opportunity to educate and to train Brazilian students on space technology. It enables to test in-orbit new components and technologies for future Brazilian missions. 1) 2)
The project enjoys technical coordination of INPE (Instituto de Pesquisas Espaciais - National Institute of Space Research), and academic coordination of ITA (Technological Institute of Aeronautics). Like INPE, ITA is also located in Sao José dos Campos in the state of Sao Paulo, Brazil. ITA is also responsible for the ITASat-1 project, construction, testing and operation.
Other participating universities in the development of ITASat-1 satellite are: Escola de Engenharia de São Carlos (EESC/USP), Universidade Federal do Rio Grande do Norte (UFRN), Universidade Estadual de Londrina (UEL), Universidade de Brasília (UnB), Universidade Estadual de Campinas (UNICAMP), Universidade Estadual Paulista "Júlio de Mesquita Filho" (FEG-UNESP) and TUB (Technical Universität of Berlin), Germany, with the ISL experiment and interchange of students.
The ITASat Project has had its origin in 2005, as a project to provide a space product in order to train and educate students. This type of space project has the endeavor to motivate students from many different backgrounds to integrate a team in a multidisciplinary field to develop capabilities not even in the universities, but also to provide competences to the Brazilian space industry sector. Hence, give the students the chance to participate in project to develop engineering skills and to solve a real space problem. - Along the project years several changes happened on the satellite configuration, going through a 100 kg microsatellite to a 6U CubeSat and this last configuration was designed, assembled and tested.
In December 2018, ITASat was launched and since its launch has been tracked and operated by the ground operation team.
Spacecraft
Developed as a 6U CubeSat, ITASat was designed to serve as a platform for future mission. In this design some aspects were considered, such as:
- Focus on the system engineering and Assembly, Integration and Testing
- Use of COTS (Commercial-Off-The-Shelf) equipment
- Modularity (in hardware and in software)
- Development of interface boards (for electrical and mechanical purposes)
- Operation of the satellite.
The decision to focus on the System Engineering, AIT, software development and Verification and Validation (V&V) allowed the team to finalize the CubeSat in two years. To be able to achieve this timeframe, most of the subsystems were COTS, specified from different suppliers in order to fulfill the requirements of the payloads. This decision required for the team quite some efforts to keep the compatibility among all the COTS components.
The 6U CubeSat bus consists of the conventional subsystems in a space platform. However, it features two OBCs (On-Board Computers). One is dedicated to on board data handling and the other is dedicated to the ADCS (Attitude Determination and Control Subsystem).
STS (Structure and Thermal Subsystem): The ITASat CubeSat internal disposition provided 2U's for the payloads and reserves 4U's for the platform, as shown in Figure 1 (right). The thermal control of ITASat is passive.

EPS (Energy and Power Supply Subsystem): The EPS is based on a COTS solution that provides 40 Wh/2600 mAh of autonomy in the Lithium-ion batteries and regulated (5 V and 3 V3) and unregulated (battery voltage – 16,8 V maximum) power lines. There are six controlled power lines used mainly for payload lines. Due to the requirements of some payloads and components selected to the project, an interface board providing additional controlled lines and a 12 V power line was developed by the team.
To generate energy, the solar panels are fixed on 5 faces of the CubeSat, with an exception to the face where the camera is placed. Triple junction solar cells are applied in the project and have an efficiency of 28%. In this configuration the EPS system has a capacity to generate an of average 6 - 7 W, considering the ITASat orbit. The conception of the EPS system is presented on Figure 2.

OBDH (On Board Data Handling Subsystem): The OBDH system is composed by a commercial ARM-Cortex M3 based computer running on FreeRTOS (Free Real Time embedded Operating System). The main features of the OBDH are:
- High performance, low power 32-bit ARM Cortex-M3-based MCU
- 4-48MHz @ 1.25 DMIPS/MHz
- Internal & external watchdog for added reliability
- 256 kB EEPROM
- 4 MB flash for code storage and 2 MB external SRAM for data storage
- SEU protection by means of an FPGA-based EDAC and SEL protection by detecting and isolating latchup currents
- MicroSD socket for storage up to 2 GB
- GPIO, I2C, SPI, CAN, and UART interfaces
- Vibration & heated vacuum tests
- Radiation tests (TID @ 20 krad, SEE @ 60 MeV).
All the embedded software was developed by the team following the architecture presented on Figure 4. The software development in layers allowed the team to divide the work and have more than one person working in the software. Also, project-based libraries could be shared between the developers. The development in layers also permitted changing the drivers without changing the application layers and aided the integration of software application developed by "third-parties".
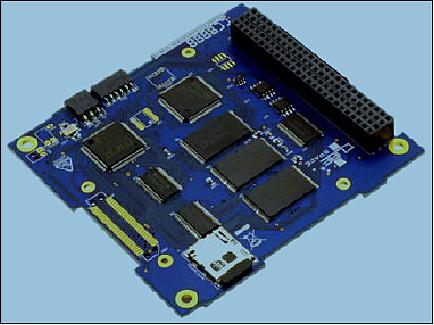
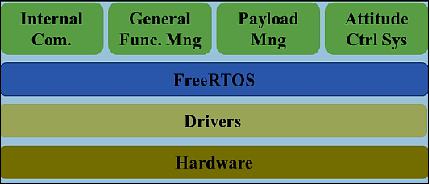
ADCS (Attitude Determination and Control System): ITASat has a dedicated computer for the ADCS, based on an ARM 7 MCU that connects directly to the sensors (sun sensor, gyrometer and magnetometer) and acts on the actuators (magneto-torquers and reaction wheels) and was designed to implement three attitude control algorithms:
• A B-dot attitude control system with the purpose to detumbling ITASat, and count on the magneto torquers as actuators and the magnetometers as sensors
• A magnetic-based control system that aligns ITASat with the Earth Magnetic Field, and
• A three-axis control system based on reaction wheels and magnetorquers as actuators and gyrometer, magnetometer and photodiode as sensors.

ITASat has also implemented an attitude determination and estimation system. A more complete description of ITASat attitude control system can be found in 3). Figure 5 presents the main components of ITASat ADCS.
TMTC (Telemetry and Telecommand Subsystem): The uplink channel of this project is based in a COTS UHF receiver in the satellite, amateur radio band coordinated with a data rate of 1200 bit/s and AFSK modulation scheme.
The CubeSat features two downlink channels using amateur radio band coordinated, one using a VHF COTS transmitter in the satellite, transmitting at 1200 to 9600 bit/s, BPSK modulation scheme and 22 dBm of RF output power, and a second COTS transmitter an S-band transmitting up to 144 kbit/s, BPSK modulation scheme and adjustable RF output power from 27 to 33 dBm. The VHF and UHF radio are integrated in only one board and are for satellite command, control and status data reception. Payload data is also transmitted using the VHF band. The S-band radio is responsible for data streaming larger science data. Figure 6 presents the UHF/VHF Transceiver (on the left) and the S-band radio (on the right).

Sensor Complement
DCS (Data collection System) Transponder
The DCS transponder was developed by INPE/CRN (Centro Regional Norte) in Natal to update the technology applied in the transponder and to miniaturize it to fit in a CubeSat (in size and power consumption). This transponder is compatible with the existing Brazilian Data Collection System.
The DCS transponders allow ITASat, a 6U CubeSat with 5.2 kg, to perform the same function as SCD-1 (Satélite de Coleta de Dados-1) and SCD-2, a 115 kg and a 117 kg satellite, respectively.
This transponder may collect data from more than 900 DCPs (Data Collection Platforms) spread over the Brazilian territory and over the coastal area. These data are related to water quality, water levels, air quality, animals' migration and so on. More information about the Brazilian Data Collection System can be found in: http://sinda.crn.inpe.br/PCD/SITE/novo/site/index.php
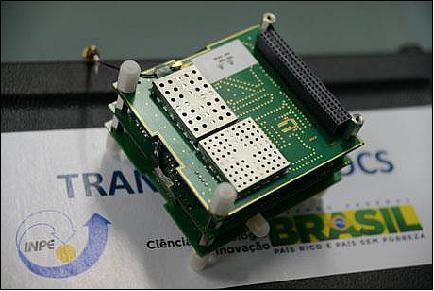
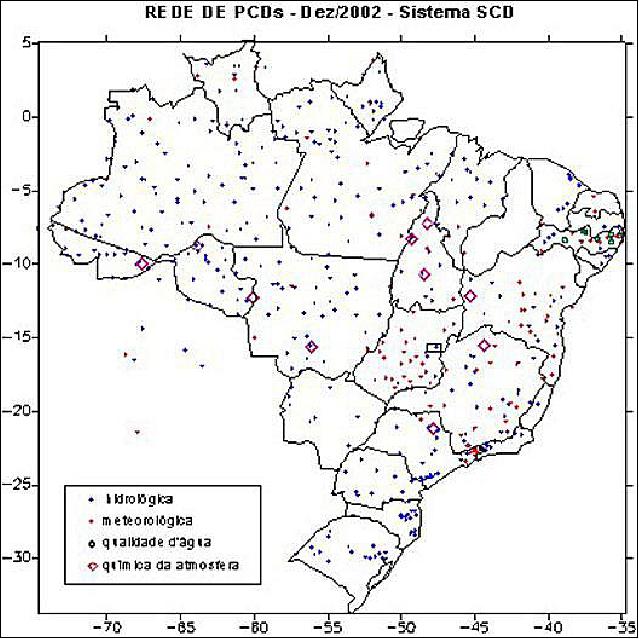
GPS Receiver
The GPS receiver was developed by the Federal University of Rio Grande do Norte (UFRN – Universidade Federal do Rio Grande do Norte) and the Aeronautics and Space Institute (IAE - Instituto de Aeronáutica e Espaço) from the Air Force Department (DCTA – Departamento de Ciência e Tecnologia Aeroespacial).
This GPS receiver has already flown on a VSB-30 rocket. To integrate the GPS receiver in the CubeSat Standard, a Mechanical Interface had to be developed as well as an electrical interface.
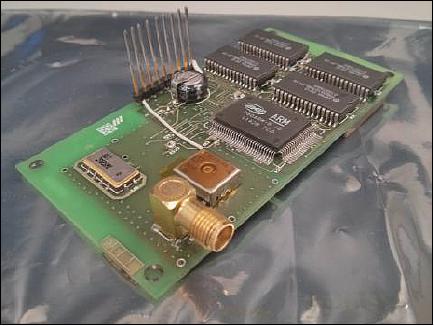
Cam (Camera)
Cam is a commercial camera observing in the visible range of the spectrum (https://gomspace.com/shop/payloads/earth-observation.aspx). The main objective is to prove the attitude control system performance. The camera is a 3 Mpixel color sensor with 35 mm lens performance of 60 m per pixel @ 650 km of altitude. In terms of image acquisition, the 1/2" (4:3) format color CMOS sensor provides an image of 2048 x 1536 pixels in a 10-bit RGB Bayer pattern.

DCX-2 (Communication Experiment-2)
The DCX-2 Experiment was proposed by the Brazilian Amateur Community to carry on board of ITASat an experiment of providing a communication channel with amateur radios over the globe. The experiment has three modes of operation:
1) Beacon: Once in beacon mode the Cubesat transmits up to 10 messages stored on board in a regular interval between the messages and the CubeSat regular beacon.
2) Transponder: in transponder mode the CubeSat re-transmits in VHF a message received in UHF to all ground station in the same footprint (coverage area) than the satellite.
3) Store and Forward: in this mode the CubeSat is able to receive a message in UHF from an amateur radio and store on board this message until a second amateur radio request this message to the satellite that is then transmitted in VHF. This allows communication of two people in different countries (or different coverage areas).
Launch
The SSO-A rideshare mission of Spaceflight was launched on 3 December 2018 (18:34:05 GMT) on a SpaceX Falcon-9 Block 5 vehicle from VAFB (Vandenberg Air Force Base) in California. 4) 5) 6)
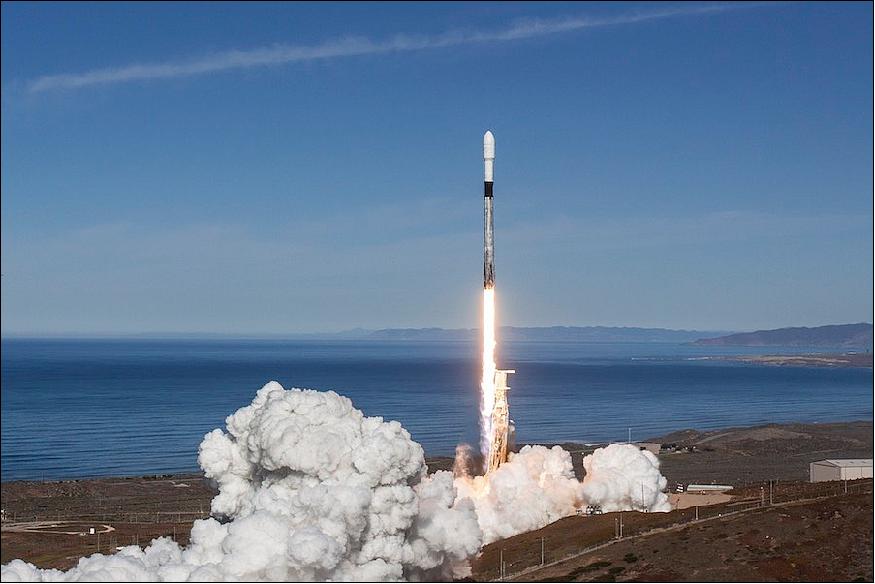
SpaceX statement: On Monday, December 3rd at 10:34 a.m. PST (18:34 GMT), SpaceX successfully launched Spaceflight SSO-A: SmallSat Express to a low Earth orbit from Space Launch Complex 4E (SLC-4E) at Vandenberg Air Force Base, California. Carrying 64 payloads, this mission represented the largest single rideshare mission from a U.S.-based launch vehicle to date. A series of six deployments occurred approximately 13 to 43 minutes after liftoff, after which Spaceflight began to command its own deployment sequences. Spaceflight's deployments are expected to occur over a period of six hours. 7)
This mission also served as the first time SpaceX launched the same booster a third time. Falcon 9's first stage for the Spaceflight SSO-A: SmallSat Express mission previously supported the Bangabandhu Satellite-1 mission in May 2018 and the Merah Putih mission in August 2018. Following stage separation, SpaceX landed Falcon 9's first stage on the "Just Read the Instructions" droneship, which was stationed in the Pacific Ocean.
Orbit: Sun-synchronous circular orbit with an altitude of 575 km, inclination of ~98º, LTDN (Local Time of Descending Node) of 10:30 hours.


Payloads on the Spaceflight SSO-A Rideshare Mission
The layout of the list follows the alphabetical order of missions as presented on the Wikipedia page "2018 in spaceflight" https://en.wikipedia.org/wiki/2018_in_spaceflight#November — as well as with the help of Gunter Krebs's short descriptions at https://space.skyrocket.de/doc_sdat/skysat-3.htm
This mission enabled 34 organizations from 17 different countries to place spacecraft on orbit. It's also special because it was completely dedicated to smallsats. Spaceflight launched 15 microsatellite and 49 CubeSats from government and commercial entities including universities, startups, and even a middle school. The payloads vary from technology demonstrations and imaging satellites to educational research endeavors.
• AISTechSat-2, a 6U CubeSat for Earth observation of AISTech (Access to Intelligent Space Technologies), Barcelona, Spain.
• Al Farabi-2, a 3U CubeSat technology demonstration mission of the Al-Farabi Kazakh National University, Kazakhstan.
• Astrocast-0.1, a 3U CubeSat technology demonstration mission of Astrocast, Switzerland, dedicated to the Internet of Things (IoT)
• Audacy-0, a 3U CubeSat technology demonstration mission of Audacy, Mountain View, CA, built by Clyde Space.
• BlackSky-2, a microsatellite (55 kg) of BlackSky Global (Seattle, WA) which will provide 1 m resolution imagery with improved geolocation accuracy.
• BRIO, a 3U CubeSat of SpaceQuest Ltd. of Fairfax, VA to test a novel communications protocol that uses SDR (Software Defined Radio).
• Capella-1, a microsatellite (37 kg) of Capella Space, San Francisco, CA featuring a X-band SAR (Synthetic Aperture) payload.
• Centauri-1, a 3U CubeSat of Fleet Space Technologies, Adelaide, South Australia. Demonstration of IoT technologies.
• CSIM-FD (Compact Spectral Irradiance Monitor-Flight Demonstration), a 6U CubeSat of LASP (Laboratory for Atmospheric and Space Physics) at the University of Boulder, CO, USA. The goal is to measure solar spectral irradiance to understand how solar variability impacts the Earth's climate and to validate climate model sensitivity to spectrally varying solar forcing.
• Eaglet-1, the first 3U CubeSat (5 kg) of OHB Italia SpA for Earth Observation.
• Elysium Star-2, a 1U CubeSat of Elysium Space providing space burial services.
• ESEO (European Student Earth Orbiter) sponsored by ESA, a microsatellite of ~40 kg with 6 instruments aboard.
• Eu:CROPIS (Euglena and Combined Regenerative Organic-Food Production in Space), a minisatellite (230 kg) of DLR, Germany. The objective is to study food production in space in support of future long-duration manned space missions (life sciences). The main payloads are two greenhouses, each maintained as a pressurized closed loop system, simulating the environmental conditions of the Moon or of Mars.
• eXCITe (eXperiment for Cellular Integration Technology), a DARPA (Defense Advanced Research Projects Agency) mission to demonstrate the 'satlets' technology. Satlets are a new low-cost, modular satellite architecture that can scale almost infinitely. Satlets are small modules that incorporate multiple essential satellite functions and share data, power and thermal management capabilities. Satlets physically aggregate in different combinations that would provide capabilities to accomplish diverse missions. Built by NovaWurks, eXCITE has a mass of 155 kg. eXCITE also carries the See Me (Space Enabled Effects for Military Engagements), a prototype microsatellite (~22 kg) built by Raytheon for DARPA to obtain on-demand satellite imagery in a timely and persistent manner for pre-mission planning.
• ExseedSat-1, a 1U CubeSat mission by the Indian company Exseed Space. The goal is to provide a multifunction UHF/VHF NBFM (Narrow Band Frequency Modulation) amateur communication satellite.
• FalconSat-6, a minisatellite (181 kg) of the USAFA (U.S. Air Force Academy) and sponsored by AFRL. FalconSat-6 hosts a suite of five payloads to address key AFSPC (Air Force Space Command) needs: SSA (Space Situational Awareness) and the need to mature pervasive technologies such as propulsion, solar arrays, and low power communications.
• Flock-3, three 3U CubeSats (5 kg each) of Planet Labs to provide Earth observation.
• Fox-1C, a radio amateur and technology research 1U CubeSat developed by AMSAT and hosting several university developed payloads.
• HawkEye, a formation-flying cluster of three microsatellites (13.4 kg each) of HawkEye 360, Herndon, VA, USA. The goal is to demonstrate high-precision RFI (Radio Frequency Interference) geolocation technology monitoring.
• Hiber-1 and-2, these are 6U CubeSats, a pathfinder mission of Hiber Global, Noordwijk, The Netherlands, for Hiber Global's planned (IoT) communications CubeSat constellation.
• ICE-Cap (Integrated Communications Extension Capability), a 3U CubeSat of the US Navy. The objectives are to demonstrate a cross-link from LEO (Low Earth Orbit) to MUOS (Mobile User Objective System) WCDMA (Wideband Code Division Multiple Access) in GEO (Geosynchronous Orbit). The objective is to send to users on secure networks.
• ICEYE-X2, a X-band SAR (Synthetic Aperture Radar) microsatellite (~ 80 kg) of Iceye Ltd, a commercial satellite startup company of Espoo, Finland.
• Irvine 02, a 1U CubeSat educational mission by the Irvine Public School Foundation, Irvine, CA. The Irvine CubeSat STEM Program (ICSP) is a multi-year endeavor that directly impacts over a hundred students from six high schools and two school districts.
• ITASat-1 (Instituto Tecnológico de Aeronáutica Satellite), a Brazilian 6U Cubesat (~8kg) built by the Instituto Tecnológico de Aeronáutica (ITA). A former rescoped microsatellite mission.
• JY1-Sat, a 1U CubeSat of Jordan developed by students of various universities. The satellite will carry a UHF/VHF amateur radio.
• KazSTSAT (Kazakh Science and Technology Satellite), a microsatellite (<100 kg) of Ghalam LLP, Astana, Kazakhstan. Developed by SSTL on a SSTL-50 platform including an SSTL EarthMapper payload designed for global commercial wide-area imaging with a resolution of 17.5 m on a swath of 250 km.
• KNACKSAT (KMUTNB Academic Challenge of Knowledge SATellite) of Thailand, a 1U technology demonstration CubeSat, the first entirely Thai-built satellite, developed by students of King Mongkut's University of Technology North Bangkok (KMUTNB). Use of an amateur radio for communication.
• Landmapper-BC (Corvus BC 4), a 6U CubeSat (11 kg) of Astro Digital (formerly Aquila Space), Santa Clara, CA, USA. The satellite features a broad coverage multispectral (Red, Green, NIR) imaging system with a resolution of 22 m.
• MinXSS-2 (Miniature X-ray Solar Spectrometer-2), a 3U CubeSat(4 kg) of LASP (Laboratory for Atmospheric and Space Physics) at the University of Colorado at Boulder,CO, USA. The objective is to study the energy distribution of solar flare SXR (Soft X-ray) emissions and its impact on the Earth's ITM (Ionosphere, Thermosphere, and Mesosphere) layers. MinXSS-2 is a copy of the MinXSS-1 but with some improvements. — MinXSS-1 was launched on 06 December 2015 onboard of Cygnus CRS-4 to the ISS, were it was deployed into orbit on 16 May 2016. It reentered Earth's atmosphere on 6 May 2017.
• NEXTSat-1, a multi-purpose microsatellite (~100 kg) of Korea designed and developed at SaTReC (Satellite Technology Research Center) of KAIST (Korea Advanced Institute of Science and Technology). The goal is to conduct scientific missions such as star formation and space storm measurements and also technology demonstration in space. Instruments: ISSS (Instrument for the Study of Space Storms) developed at KAIST to detect plasma densities and particle fluxes of 10 MeV energy range near the Earth. NISS (NIR Imaging Spectrometer for Star formation history), developed at KASI (Korean Astronomy and Space Science Institute).
• Orbital Reflector, a 3U CubeSat project (4 kg) of the Nevada Museum of Art and artist Trevor Paylon. The Orbital Reflector is a 30 m sculpture constructed of a lightweight material similar to Mylar. On deployment, the sculpture self-inflates like a balloon. Sunlight reflects onto the sculpture making it visible from Earth with the naked eye — like a slowly moving artificial star as bright as a star in the Big Dipper.
• ORS-7 (Operationally Responsive Space 7), two 6U CubeSats (-7A and -7B) of the USCG (US Coast Guard) in cooperation with DHS (Department of Homeland Security), the ORS (Operationally Responsive Space Office) of DoD, and NOAA. The objective is to detect transmissions from EPIRBs (Emergency Position Indicating Radio Beacons), which are carried on board vessels to broadcast their position if in distress.
• PW-Sat 2 (Politechnika Warszawska Satellite 2), a 2U CubeSat of the Institute of Radioelectronics at the Warsaw University of Technology, Warsaw, Poland. The objective is to demonstrate a deorbitation system - a drag parachute opened behind the satellite - which allows faster removal of satellites from their orbit after it completes its mission.
• RAAF-M1 (Royal Australian Air Force-M1), an Australian 3U CubeSat (~4 kg) designed and built by UNSW (University of New South Wales) for the Australian Defence Force Academy, Royal Australian Air Force. RAAF-M1 is a technology demonstration featuring an AIS receiver, and ADS-B receiver, an SDR (Software Defined Radio).
• RANGE-A and -B (Ranging And Nanosatellite Guidance Experiment), two 1.5 CubeSats of Georgia Tech (Georgia Institute of Technology), Atlanta, GA, USA, flying in a leader-follower formation with the goal of improving the relative and absolute positioning capabilities of nanosatellites.
• ROSE-1, a 6U CubeSat of Phase Four Inc., El Segundo, CA, USA. ROSE-1 is an experimental spacecraft designed to provide an orbital test-bed for the Phase Four RFT (Radio Frequency Thruster), the first plasma propulsion system to fly on a nanosatellite.
• SeaHawk-1, a 3U CubeSats of UNCW (University of North Carolina, Wilmington), NC. The goal is to measure the ocean color in project SOCON (Sustained Ocean Observation from Nanosatellites). SeaHawk is considered a prototype for a larger constellation. The SOCON project is a collaboration between Clyde Space Ltd (spacecraft bus), the University of North Carolina Wilmington, Cloudland Instruments, and NASA/GSFC (Goddard Space Flight Center).
• See Me (Space Enabled Effects for Military Engagements), a prototype microsatellite (~22 kg) built by Raytheon for DARPA to obtain on-demand satellite imagery in a timely and persistent manner for pre-mission planning.
• SkySat-14 and -15. Planet Labs of San Francisco has 13 SkySats in orbit. The commercial EO satellites were built by Terra Bella of Mountain View, CA, which Planet Labs acquired from Google last year. At the time of the purchase, there were 7 SkySats in orbit. On 31 October 2017, Planet launched an additional six on a Minotaur-C rocket. The 100 kg SkySats are capable of sub-meter resolution – making them the most powerful in the constellation. Customers can request to have these high-resolution satellites target their locations of interest.
• SNUGLITE, a 2U CubeSat designed by the SNU (Seoul National University) for technology demonstrations and amateur radio communication.
• SpaceBEE, four picosatellites of Swarm Technologies (a US start-up), built to the 0.25U form factor to make up a 1U CubeSat.
• STPSat-5 is a science technology minisatellite of the US DoD STP (Space Test Program), managed by the SMC of the USAF. STPSat-5 will carry a total of five technological or scientific payloads to LEO (Low Earth Orbit) in order to further the DoD's understanding of the space environment. The satellite was built by SNC (Sierra Nevada Corporation) on the modular SN-50 bus with a payload capacity of 50-100 kg and compatible with ESPA-class secondary launch adaptors.
• THEA, a 3U CubeSat built by SpaceQuest, Ltd. of Fairfax, VA to demonstrate a spectrum survey payload developed by Aurora Insight, Washington DC. The objective is to qualify Aurora's payload, consisting of a proprietary spectrometer and components, and demonstrate the generation of relevant measurements of the spectral environment (UHF, VHF, S-band). The results of the experiment will inform future development of advanced instrumentation by Aurora and component development by SpaceQuest.
• VESTA is a 3U CubeSat developed at SSTL in Guildford, UK. VESTA is a technology demonstration mission that will test a new two-way VHF Data Exchange System (VDES) payload for the exactEarth advanced maritime satellite constellation. Honeywell Aerospace is providing the payload. VESTA is a flagship project of the National Space Technology Program, funded by the UK Space Agency and managed by the Center for EO Instrumentation and Space Technology (CEOI-ST).
• VisionCube-1, a 2U CubeSat designed by the Korea Aerospace University (KAU) to perform research on Transient Luminous Events in the upper atmosphere. The image processing payload consists of a multi-anode photon multiplier tube(MaPMT), a camera, and a real-time image processing engine built by using SoC (System-on-Chip) FPGA technologies.
Spaceflight has contracted with 64 spacecraft from 34 different organizations for the mission to a Sun-Synchronous Low Earth Orbit. It includes 15 microsatellites and 49 CubeSats from both commercial and government entities, of which more than 25 are from international organizations from 17 countries, including United States, Australia, Italy, Netherlands, Finland, South Korea, Spain, Switzerland, UK, Germany, Jordan, Kazakhstan, Thailand, Poland, Canada, Brazil, and India. 8)

Statement from the Mission Manager of Spaceflight
Most will never know all that is necessary to plan a launch, and then add to that the challenge of managing and being responsible for the launch of 64 satellites, a record breaking event to be sure, but that's exactly what Spaceflight did. 9)
Spaceflight, the leading rideshare and mission management provider, today announced the success of its SSO-A: SmallSat Express mission, the largest single rideshare mission from a U.S.-based launch vehicle to date. The company successfully launched 64 spacecraft to sun-synchronous low Earth orbit via a SpaceX Falcon 9 that launched today from Vandenberg Air Force Base.
"This was an incredibly complex mission, and I'm extremely proud of what our talented team at Spaceflight has achieved," said Curt Blake, president of Spaceflight. "SSO-A is a major milestone for Spaceflight and the industry. We've always been committed to making space more accessible through rideshare. This mission enabled 34 organizations from 17 different countries to place spacecraft on orbit. It's also special because it was completely dedicated to smallsats."

Spaceflight launched 15 MicroSats and 49 CubeSats from government and commercial entities including universities, startups, and even a middle school. The payloads vary from technology demonstrations and imaging satellites to educational research endeavors.
One research payload includes the University of North Carolina Wilmington's CubeSat, SeaHawk-1 carrying the HawkEye Ocean Color Imager. UNCW has been funded by the Gordon and Betty Moore Foundation, and NASA serves in an advisory capacity to ensure the maximum scientific utility of the science data. NASA's Science Mission Directorate and UNCW have created a partnership to expand accessibility to the data.
"We are thrilled to have SeaHawk-1 on orbit and to be part of such a historic launch superbly executed by Spaceflight," said Professor John Morrison, SeaHawk's co-project manager and lead principal investigator. "SeaHawk will make ocean observations at significantly higher spatial resolution and at much lower costs than standard satellite systems. Since the data collected will be publicly available, our hope is that it will benefit not only researchers, but policymakers and others to make informed decisions when addressing issues related to the environment."
To accommodate the large number of payloads, Spaceflight built an integrated payload stack that was nearly 20 feet tall. Once the launch vehicle reached orbit, the upper and lower free flyers separated from the vehicle. The free flyers then successfully deployed all spacecraft, dispensing one payload every five minutes over five hours.
"This launch was an impressive undertaking and an important milestone for the smallsat industry as well as for many of the organizations involved," said Payam Banazadeh, founder and CEO of Capella Space Corporation. "Capella's first satellite is now on orbit and we are one step closer to our goal of providing timely, reliable, and frequent information using Synthetic Aperture Radar technology."
With the success of SSO-A, Spaceflight has now launched more than 210 satellites since its founding in 2011. In addition, the company is contracted to launch nearly 100 satellites in 2019. Among the upcoming launches is Spaceflight's next dedicated rideshare mission, which will occur in 2019 on a Rocket Lab Electron.
ITASat LEOP (Launch and Early Orbit Phase)
A description of the ITASat ConOps is presented.

For ITASat operation a ground station located at ITA, in São José dos Campos, was elected as the main station and the MOC (Mission Control Center). A ground Station located at Universidade Federal de Santa Maria (UFSM) was selected as a backup station. Afterwards a ground station located at INPE CRN in Natal was also include in the operation. All three stations are from INPE and work in a straight cooperation with ITA. These three stations can send commands and receive telemetries of ITASat. ITA ground operation team is responsible for providing to the other stations regularly the operation plan. Figure 18 shows the real location of the three ground stations inside the Brazilian territory (a) and ITA Ground Station (b).

Mission Status
• According to our orbit propagation, after jettison and the requirement to stay 30 minutes in silence, the first telemetry of ITASat (beacon) should occur in about 3h and 30 minutes from the T0 , the launch event (Ref: 1).
- By the time, ITASat should be flying over Europe. In this first pass over Europe, any European amateur radio had noticed the reception of the beacon. At around 10:20 pm (BRST), in the first pass over Brazil, the first ITASat beacon was received by an amateur radio called Roland (PY4ZBZ). This amateur radio is located in the city of Sete Lagoas, Minas Gerais, in the South-west region of Brazil. From this moment, more amateur radios and ground station were able to receive telemetries of our CubeSat. ITASat has been operated since then and the payloads commissioning has started.
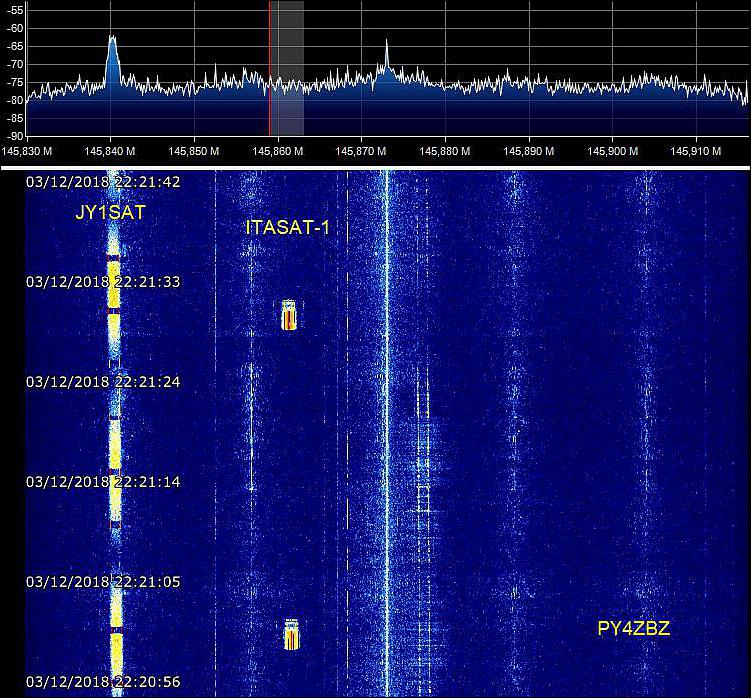
Lessons Learned
During the project life cycle many lessons were learned in the process to develop, integrate, test and operate ITASat. Some of these lessons will be present in this section (Ref. 1).
Developing approach: On ITASat, during the development phase, an incremental approach was implemented and used. This approach aided the development process and allowed the verification after each increment, increasing the confidence of the project. This incremental approach was used in the SW development and platform assembly.
Another important aspect was the modular development approach implemented in the project, in addition to the incremental approach. This allowed to isolate functions independently, speeding the development and allowing teams to work in parallel. The modular approach also aided the exchange of parts and facilitate software in the loop development and testing, as well as hardware in the loop development and testing.
The incremental and modular approach was applied in the software architecture as well. The software architecture was defined in layers, since the beginning of the development. Because of this definition, the software development was divided in small parts and layers making it easier to handle the software. Different teams developed the application layer and the supplier heritage codes could be adapted for the project necessities. As an example, the DCX-2 code was developed by an amateur radio and integrated in our software in a few hours; it is working perfectly in the CubeSat. The key point on this approach was the clear definition of the software interfaces.
Electrical Interfaces for communication: On ITASat, the main bus for communication among the onboard computer and all equipment and payload relies on I2C. This approach showed us that the I2C has electrical and logical limitations that causes the bus to overload, resulting in bus locking.
Even following all recommendations of cable lengths, pull up resistor location - for several times the team faced problems in the signal reception due to line capacitance changes, as a consequence electrical changes had to be made. But the main issue was the bus overload and bus locking. The ADCS could not be fully tested because in the 3-axis controlled mode, there is a bus overload that causes a halt in the I2C communication flux. Consequently, the onboard computer resets the system due to a failure on the I2C line. The function to reset the system was implemented as a FDIR (Failure, Detection, Identification and Recovery) mechanism.
Late access on the CubeSat: ITASat has only one connector that concentrates the functions of charging the battery, debug and ABF (Apply Before Flight). No late access for software uploads were provisioned, what caused some restrictions to software upload after final integration.
Another issue on the late access connector was its location. On ITASat the location of the connectors do not prioritize the disassembly, they were placed on the solar panel, so for each disassembly we needed to handle the solar panels, with the risk to cause a damage.
From the project side, we had only little information regarding the CubeSat dispenser during the development phase and we had to make some assumptions that were not the best solution or the best choice for the project. It is recommended that all possibilities be studied to reduce these risks.
Selection of the parts and Procurement: By the time of the development and specification of the parts of the satellite, not all requirements were well defined and not all the characteristics of the parts were well known, this caused some restrictions afterwards during the development.
As an example, on ITASat the "sun sensor" was a simple photodiode with a FOV of 60 degrees, that limits the use of the generated data in the ADCS system, causing blind zones where the sun position cannot be determined, thus reducing the performance of the attitude determination and control algorithm.
On the ITASat project, major project delays occurred due to the delay on the supplier's chain and bureaucracy of the purchase process. Delays in these processes (almost) always occur!
Another point included in the procurement is related to the acceptance of the parts. A well-defined and an established process to accept the parts needs to be implemented in the project.
Communication: One of the biggest issues in any project is the communication, among the developers, with the suppliers, with the payload developers.
However, on ITASat a close contact and frequent communication with the payload developers allowed us to implement and integrate the experiments with a low re-work rate, but it had to be done.
A good channel of communication was also established with most of the suppliers, so creating a good network is fundamental to the success of the mission.
AIT (Assembly, Integration and Test) process: During ITASat assembly, integration and tests, in particular to the dispenser, we realized that it would be a lot easier (and safer) if we had implemented secure mechanisms and devices for handling the satellite. The reflection of having few information of the dispenser in the early phases led us to not consider some restrictions, and we failed in the first fit check.
At the project, the in-house development, due to the lack of time and resources, were not exhaustively tested and it caused minor non-conformities after the acceptance tests. These non-conformities had to be managed by the team to be ready for flight.
Before the AIT of the Protoflight Model, we wrote a detailed procedure and this procedure was checked during the assembly stage. This procedure was tested in the Engineering Model.
The cable specifications and cable lengths were checked using the Engineering Model and 3D printed parts, aiding the team to see the cables routing inside the CubeSat. Each cable has a specification sheet and specific tags to avoid a mismatch.
Use of two on board computers: One of the features of the ITASat CubeSat is the fact that we have two onboard computers on board. One computer for mission data management and the other one dedicated to the attitude control system. This approach brought a lot of challenges to the team, since it was not easy to synchronize the operation of both computers, considering that each computer is the master of the communication in some sort of moment, but it gave to the platform more flexibility to adapt to changes and more flexibility during tests, once it was possible to perform tests separately.
The importance of the System Engineering: According to NASA Systems Engineering Handbook, "the objective of systems engineering is to see to it that the system is designed, built, and operated so that it accomplishes its purpose in the most cost-effective way possible, considering performance, cost, schedule and risk."
So, one of the purposes of System Engineering is to anticipate requirements and analyze the solution in all project phases. Even though efforts were applied on system engineering, the team had to learn how system engineering works during the execution of the project, and it led to a lack of efforts in some aspects and excess in other ones, as an example, many efforts were done on system requirements and modeling for developing purposes, but just few observations were done during operation and post integration.
A further example, already mentioned, we had no information about the dispenser internal envelope for the payload (CubeSat) at early stage, and consequently we did not pass in the FIT check at the first time.
Other points were the considerations in the mission analysis. The simulations of the power generation capacity and battery DoD performance where not confirmed during operation. As a result, we faced difficulties to charge the batteries to secure levels for operation, therefore we have resets more often than expected or designed. In this case it is recommended to include additional margins in the analysis.
The good results of applying system engineering in the project were:
• After some reviews the configuration was baselined, and no changes were made after the project CDR (Critical Design Review).
• Use of SysML/UML to document the requirements and use cases, it reduced a bit the paperwork and aided the traceability.
• An established Document Tree and Configuration Management was implemented.
• Software was documented and configured using a GitHub-like repository, aiding the configuration control and version control with no need of paper and manual control.
• AIT procedures were documented and realized by more than one person, doing a double check reducing the margin of error.
Schedule and Risks: From the beginning to the PFM (Proto Flight Model) system, the project was developed in two years, but some components were bought in very early stages of the project, increasing the risks of incompatibilities. This decision was made to reduce the impacts of delays on the project, and it showed quite important considering that we had delays on the procurement.
On ITASat, a simplified risk analysis was performed, and one major risk was the absence of spare parts for all components of the satellite. The other major risk was the human resource, but this risk was successfully mitigated.
Another aspect to be addressed is the shelf life of the satellite. The ITASat shelf-life was longer than expected due to launch opportunities and delays. The battery charge procedures and impacts had to be carried out along the time, but it is clear now that these processes should be carried out in early phases of the project.
Mission Operation: On ITASat, the main station is located at ITA in São José dos Campos. During the operation, we realized that for many times a coordination with other ground stations improved the operation, and in some cases it was mandatory. At the beginning of the ITASat operation, our station was OFF due to some technical problems. Hence, we counted on the data provided by amateur radios and partner ground stations. It showed us the importance of having backup ground stations and spare parts to quickly fix problems.
One other point is that the beacon signal of ITASat is configured by software on the ground to be sent in a fixed time interval and there is no possibility to change this interval on flight. For many times we realized that the capability to change this time interval would give us more information when needed and less information in cases we would like to save power.
At the development time, we intended to implement a history log, but for many reasons, ITASat does not have a history log with the behavior of the observatory over time. We have only beacon information, so we have some lacks in time with no information of the observatory behavior at all. The absence of this information makes a bit more difficulty the analysis of the CubeSat performance.
The team: The team is the heart of the any project, once projects and many other tasks are developed by people for people. The development team was one of the main strengths of this project.
The ITASat team is located in two offices, close to each other and it improved the speed and quality of the development. This proximity allowed more discussion inside the team and a better understanding of the mutual work package, resulting in a team that knows well its role in the project and the impact of their work in each part, because they are close to the tasks for the whole team.
In the project there was a perceptive improvement after the project incorporated workers with experience on aerospace projects. The mix of expertise of students and professionals lead to a more robust team.
Summary
Besides all the challenges that the project experienced throughout the years, since its beginning in 2005 until the launch in 2018, including the main change to a CubeSat approach in 2013, the project was very successful in achieving its main objective, that was to train human resources for space projects and also to develop a platform for future missions. Many of the former ITASat students are now working in the space business or related areas, where the acquired knowledge in the space area creates a competitive advantage.
The project helped ITA to establish an aerospace engineering course and improved the competence in this field in Brazil. The project demonstrated also that a 6U satellite can accomplish missions that were before restricted to bigger satellites, like collecting data for several ground platforms around the country. The project showed also that CubeSats are not toys or plug-and-play devices: they can be so complex than bigger satellites, depending on the mission and the solutions that has been chosen.
ITA made the choice to complexity, expressed by ITASat two on-board computers and payed the 'price', expressed by the difficulties with the I2C bus. But exactly the overcome of these difficulties allowed the technical grow of the students involved in the project. The expertise developed by ITA in the design of this 6U platform allowed ITA to propose a joint mission between Brazil and United States, called SPORT to investigate the ionosphere. In this new mission, all lessons learned from ITASat have been implemented and a more robust platform is under development. The lessons learned comprise things that we did well and things that we decided to change or improve, and tolls that we use and it went so well, that we intend to use in a future mission as a baseline or a project standard for the laboratory projects, like the Systems Engineering Methodology.
References
1) Lidia Hissae Shibuya Sato, Luis Eduardo Vergueiro Loures da Costa, Jonas Bianchini Fulindi, Helio André dos Santos, Linélcio dos Santos Paula, Emerson Henrique Silva de Oliveira, Jéssica Garcia de Azevedo, Breno Aparecido Crucioli, Denis Guilgim Vieira, Valdemir Carrara, Ana Carolina di Iorio Jeronymo, Rafael Barbosa Januzi, Daniel Hideaki Makita, Willer Gomes dos Santos, Pedro Kukulka de Albuquerque, Maria de Fátima Mattiello-Francisco, "The ITASAT – The Lessons Learned from the Mission Concept to the Operation," Proceedings of the 33rd Annual AIAA/USU Conference on Small Satellites, August 3-8, 2019, Logan, UT, USA, SSC19-WKI-01, URL: https://digitalcommons.usu.edu/cgi/viewcontent.cgi?article=4350&context=smallsat
2) Lidia Hissae Shibuya Sato, Osamu Saotome, Christina Timm, David Fernandes, Wilson Yamaguti, "ITASAT-1: Brazilian university microsatellite for payload test and validation in Low Earth Orbit," 8th IAA (International Academy of Astronautics) Symposium on Small Satellites for Earth Observation, Berlin, Germany, April 4-8, 2011, URL: http://media.dlr.de:8080/erez4/erez?cmd=get&src=os
/IAA/archiv8/Presentations/IAA-B8-0601_v2-1.pdf
3) Valdemir Carrar, Rafael Barbosa Januzi, Daniel Hideaki Makita, Luis Felipe de Paula Santos, Lidia Shibuya Sato, "The ITASat CubeSat Development and Design," Journal of Aerospace Technology and Management, Vol.9, No 2, pp.147-156, São José dos Campos, Brazil, April/June 2017, URL: http://www.scielo.br/pdf/jatm/v9n2/2175-9146-jatm-09-02-0147.pdf
4) Stephen Clark, "Spaceflight's 64-satellite rideshare mission set to last five hours," Spaceflight Now, 3 December 2018, URL: https://spaceflightnow.com/2018/12/03
/spaceflights-64-satellite-rideshare-mission-set-to-last-five-hours/
5) Stephen Clark, "Spaceflight preps for first launch of unique orbiting satellite deployers," Spaceflight Now, 23 August 2018, URL: https://spaceflightnow.com/2018/08/23
/spaceflight-preps-for-first-launch-of-unique-orbiting-satellite-deployers/
6) Jeff Foust, "Spaceflight gears up for dedicated Falcon 9 launch," Space News, 6 August 2018, URL: https://spacenews.com/spaceflight-gears-up-for-dedicated-falcon-9-launch/t).
7) "Spaceflight SSO-A: SmallSat Express Mission," SpaceX, 3 December 2018, URL: https://web.archive.org/web/20181204085402/https://www.spacex.com/news/2018/12/03/spaceflight-sso-smallsat-express-mission
8) "Spaceflight - Introducing SSO-A: The smallsat express," Spaceflight, 3 December 2018, URL: http://spaceflight.com/sso-a/
9) "Statement from Spaceflight, the Mission Manager, Launches 64 Satellites on First Dedicated Rideshare Mission," Satnews Daily, 4 December 2018, URL: http://www.satnews.com/story.php?number=270036615
The information compiled and edited in this article was provided by Herbert J. Kramer from his documentation of: "Observation of the Earth and Its Environment: Survey of Missions and Sensors" (Springer Verlag) as well as many other sources after the publication of the 4th edition in 2002. - Comments and corrections to this article are always welcome for further updates (eoportal@symbios.space).
Spacecraft Sensor Complement Launch Payloads LEOP Mission Status References Back to top